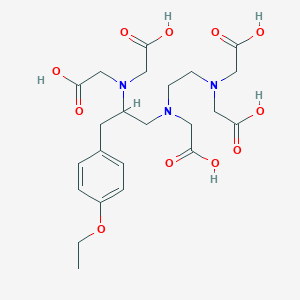
Caloxetic acid
Übersicht
Beschreibung
- Caloxetsäure, auch bekannt als EOB-DTPA, ist ein Chelatbildner.
- Ihre chemische Formel lautet C₂₃H₃₃N₃O₁₁ und ihr Molekulargewicht beträgt etwa 527,52 g/mol .
- Sie erscheint als blassgelber bis gelber Feststoff .
- Caloxetsäure ist hygroskopisch und hat einen vorhergesagten Schmelzpunkt von >167°C (Sublimation) .
Wissenschaftliche Forschungsanwendungen
- Diese Komplexe verbessern den Gewebekontrast und helfen bei der Diagnose verschiedener Erkrankungen .
MRT-Kontrastmittel: Caloxetsäurederivate werden verwendet, um MRT-Bilder zu verbessern, indem sie an Gadoliniumionen binden.
Wirkmechanismus
- Der Wirkmechanismus von Caloxetsäure liegt in ihrer Fähigkeit, Gadoliniumionen zu chelatisieren .
- Sie bildet stabile Komplexe mit Gadolinium, was die Signalintensität in der MRT erhöht.
- Die molekularen Zielstrukturen sind die Gadoliniumionen selbst, und der Weg beinhaltet ihre Wechselwirkung mit Wasserprotonen während der Bildgebung.
Vorbereitungsmethoden
- Synthesewege für Caloxetsäure sind nicht umfassend dokumentiert, aber sie wird üblicherweise durch chemische Synthese hergestellt.
- Industrielle Produktionsmethoden können Modifikationen bestehender Chelatbildner oder kundenspezifische Synthese umfassen.
Analyse Chemischer Reaktionen
- Caloxetsäure kann verschiedene Reaktionen eingehen, darunter Chelatreaktionen mit Metallionen.
- Häufige Reagenzien sind Metallsalze (z. B. Gadoliniumsalze) und organische Basen .
- Die wichtigsten gebildeten Produkte sind Gadoliniumkomplexe , die als Kontrastmittel in der Magnetresonanztomographie (MRT) verwendet werden .
Wirkmechanismus
- Caloxetic acid’s mechanism of action lies in its ability to chelate gadolinium ions .
- It forms stable complexes with gadolinium, which enhances the MRI signal intensity.
- The molecular targets are the gadolinium ions themselves, and the pathway involves their interaction with water protons during imaging.
Vergleich Mit ähnlichen Verbindungen
- Caloxetsäure ist aufgrund ihrer spezifischen Chelateigenschaften für Gadolinium einzigartig.
- Ähnliche Verbindungen umfassen andere Chelatbildner, die in MRT-Kontrastmitteln verwendet werden, wie z. B. DTPA (Diethylentriaminpentaessigsäure) und EDTA (Ethylendiamintetraessigsäure).
Eigenschaften
IUPAC Name |
2-[[2-[bis(carboxymethyl)amino]-3-(4-ethoxyphenyl)propyl]-[2-[bis(carboxymethyl)amino]ethyl]amino]acetic acid | |
---|---|---|
Details | Computed by Lexichem TK 2.7.0 (PubChem release 2021.10.14) | |
Source | PubChem | |
URL | https://pubchem.ncbi.nlm.nih.gov | |
Description | Data deposited in or computed by PubChem | |
InChI |
InChI=1S/C23H33N3O11/c1-2-37-18-5-3-16(4-6-18)9-17(26(14-22(33)34)15-23(35)36)10-24(11-19(27)28)7-8-25(12-20(29)30)13-21(31)32/h3-6,17H,2,7-15H2,1H3,(H,27,28)(H,29,30)(H,31,32)(H,33,34)(H,35,36) | |
Details | Computed by InChI 1.0.6 (PubChem release 2021.10.14) | |
Source | PubChem | |
URL | https://pubchem.ncbi.nlm.nih.gov | |
Description | Data deposited in or computed by PubChem | |
InChI Key |
AQOXEJNYXXLRQQ-UHFFFAOYSA-N | |
Details | Computed by InChI 1.0.6 (PubChem release 2021.10.14) | |
Source | PubChem | |
URL | https://pubchem.ncbi.nlm.nih.gov | |
Description | Data deposited in or computed by PubChem | |
Canonical SMILES |
CCOC1=CC=C(C=C1)CC(CN(CCN(CC(=O)O)CC(=O)O)CC(=O)O)N(CC(=O)O)CC(=O)O | |
Details | Computed by OEChem 2.3.0 (PubChem release 2021.10.14) | |
Source | PubChem | |
URL | https://pubchem.ncbi.nlm.nih.gov | |
Description | Data deposited in or computed by PubChem | |
Molecular Formula |
C23H33N3O11 | |
Details | Computed by PubChem 2.2 (PubChem release 2021.10.14) | |
Source | PubChem | |
URL | https://pubchem.ncbi.nlm.nih.gov | |
Description | Data deposited in or computed by PubChem | |
DSSTOX Substance ID |
DTXSID30869844 | |
Record name | N-{2-[Bis(carboxymethyl)amino]-3-(4-ethoxyphenyl)propyl}-N-{2-[bis(carboxymethyl)amino]ethyl}glycine | |
Source | EPA DSSTox | |
URL | https://comptox.epa.gov/dashboard/DTXSID30869844 | |
Description | DSSTox provides a high quality public chemistry resource for supporting improved predictive toxicology. | |
Molecular Weight |
527.5 g/mol | |
Details | Computed by PubChem 2.2 (PubChem release 2021.10.14) | |
Source | PubChem | |
URL | https://pubchem.ncbi.nlm.nih.gov | |
Description | Data deposited in or computed by PubChem | |
CAS No. |
135306-78-4 | |
Record name | Caloxetic acid | |
Source | ChemIDplus | |
URL | https://pubchem.ncbi.nlm.nih.gov/substance/?source=chemidplus&sourceid=0135306784 | |
Description | ChemIDplus is a free, web search system that provides access to the structure and nomenclature authority files used for the identification of chemical substances cited in National Library of Medicine (NLM) databases, including the TOXNET system. | |
Retrosynthesis Analysis
AI-Powered Synthesis Planning: Our tool employs the Template_relevance Pistachio, Template_relevance Bkms_metabolic, Template_relevance Pistachio_ringbreaker, Template_relevance Reaxys, Template_relevance Reaxys_biocatalysis model, leveraging a vast database of chemical reactions to predict feasible synthetic routes.
One-Step Synthesis Focus: Specifically designed for one-step synthesis, it provides concise and direct routes for your target compounds, streamlining the synthesis process.
Accurate Predictions: Utilizing the extensive PISTACHIO, BKMS_METABOLIC, PISTACHIO_RINGBREAKER, REAXYS, REAXYS_BIOCATALYSIS database, our tool offers high-accuracy predictions, reflecting the latest in chemical research and data.
Strategy Settings
Precursor scoring | Relevance Heuristic |
---|---|
Min. plausibility | 0.01 |
Model | Template_relevance |
Template Set | Pistachio/Bkms_metabolic/Pistachio_ringbreaker/Reaxys/Reaxys_biocatalysis |
Top-N result to add to graph | 6 |
Feasible Synthetic Routes
Haftungsausschluss und Informationen zu In-Vitro-Forschungsprodukten
Bitte beachten Sie, dass alle Artikel und Produktinformationen, die auf BenchChem präsentiert werden, ausschließlich zu Informationszwecken bestimmt sind. Die auf BenchChem zum Kauf angebotenen Produkte sind speziell für In-vitro-Studien konzipiert, die außerhalb lebender Organismen durchgeführt werden. In-vitro-Studien, abgeleitet von dem lateinischen Begriff "in Glas", beinhalten Experimente, die in kontrollierten Laborumgebungen unter Verwendung von Zellen oder Geweben durchgeführt werden. Es ist wichtig zu beachten, dass diese Produkte nicht als Arzneimittel oder Medikamente eingestuft sind und keine Zulassung der FDA für die Vorbeugung, Behandlung oder Heilung von medizinischen Zuständen, Beschwerden oder Krankheiten erhalten haben. Wir müssen betonen, dass jede Form der körperlichen Einführung dieser Produkte in Menschen oder Tiere gesetzlich strikt untersagt ist. Es ist unerlässlich, sich an diese Richtlinien zu halten, um die Einhaltung rechtlicher und ethischer Standards in Forschung und Experiment zu gewährleisten.