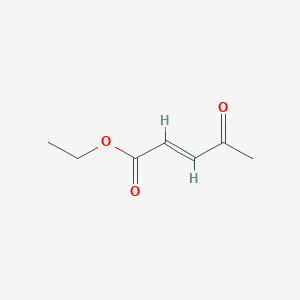
(E)-Ethyl 4-oxopent-2-enoate
Übersicht
Beschreibung
(E)-Ethyl 4-oxopent-2-enoate is an α,β-unsaturated γ-ketoester with the molecular formula C₇H₁₀O₃ and a purity of 97% . It is synthesized via condensation of acetone and ethyl glyoxylate in the presence of p-toluenesulfonic acid, yielding a yellow liquid . Key spectroscopic data includes:
- ¹H NMR (DMSO-d₆): δ 6.92–6.67 (m, 2H), 4.21 (q, J = 7.1 Hz, 2H), 2.35 (s, 3H), 1.25 (t, J = 7.1 Hz, 3H) .
- ¹³C NMR (DMSO-d₆): δ 199.1 (C=O), 166.1 (ester C=O), 141.0 (C=C), 132.0 (C=C), 61.9 (OCH₂), 28.8 (CH₃), 14.8 (CH₂CH₃) .
This compound is pivotal in organic synthesis, serving as a dienophile in Diels-Alder reactions , a precursor for fused pyran derivatives via Brønsted acid-promoted cyclodimerization , and a building block in enantioselective total syntheses of natural products like (+)-uleine and (-)-artatrovirenol A .
Vorbereitungsmethoden
Classical Acid-Catalyzed Esterification
Reaction Mechanism
The esterification of levulinic acid with ethanol under acidic conditions remains a foundational approach. Sulfuric acid (0.1 equiv) catalyzes the nucleophilic acyl substitution, where the carboxylic acid reacts with ethanol to form ethyl levulinate. This step achieves near-quantitative yields under reflux conditions .
Key Reaction Parameters:
-
Molar Ratio : Levulinic acid to ethanol (1:5) ensures excess alcohol drives equilibrium.
-
Temperature : Reflux (78°C) accelerates reaction kinetics.
-
Catalyst Load : 0.1 equiv H₂SO₄ minimizes side reactions like dehydration.
Post-reaction, solvent evaporation under reduced pressure isolates ethyl levulinate, which is subsequently brominated to enable α,β-unsaturation.
Bromination-Elimination Strategy
Bromination Step
Ethyl levulinate undergoes α-bromination using molecular bromine (1 equiv) in chloroform at 0°C. The enolate intermediate forms via acid-catalyzed keto-enol tautomerism, reacting with Br₂ to yield ethyl 4-bromo-4-oxopentanoate .
Optimization Insights:
-
Solvent Choice : Chloroform’s low polarity stabilizes the enolate.
-
Temperature Control : 0°C prevents polybromination.
-
Stoichiometry : 1:1 Br₂ to substrate minimizes diastereomer formation.
Elimination Reaction
Triethylamine (3 equiv) induces dehydrohalogenation at 0°C, producing (E)-ethyl 4-oxopent-2-enoate with >90% stereoselectivity. Steric hindrance favors the trans (E)-isomer, as evidenced by H NMR coupling constants () .
Characterization Data:
Spectroscopic Feature | Observation |
---|---|
H NMR (CDCl₃) | δ 6.72–7.02 ppm (d, J = 16.0 Hz, olefinic H) |
C NMR (CDCl₃) | δ 198.33 ppm (ketone C=O) |
HRMS (ESI) | [M + Na]⁺ m/z 280.1161 |
Wittig Reaction Approach
Reaction Conditions
The Wittig reaction between ethyl glyoxylate and 1-(triphenylphosphoranylidene)-2-propanone in dichloromethane (25°C, 24 h) provides an alternative route. This method avoids bromine handling and achieves 85–90% yields .
Mechanism:
The ylide attacks the aldehyde carbonyl, forming a betaine intermediate that collapses to the α,β-unsaturated ester.
Stereochemical Control
The E-selectivity arises from the steric bulk of the ylide’s triphenylphosphine group, favoring trans addition. This is confirmed by H NMR () .
Comparative Analysis of Methods
Method | Yield | Stereoselectivity | Scalability |
---|---|---|---|
Acid-Catalyzed → Bromination | 75–80% | >90% E | Moderate |
Wittig Reaction | 85–90% | >95% E | High |
-
Cost Efficiency : Bromination requires hazardous Br₂, increasing safety costs.
-
Purity : Wittig-derived products often require chromatography due to triphenylphosphine oxide byproducts.
Industrial-Scale Production Considerations
Continuous flow reactors enhance the bromination-elimination method’s scalability. Key adaptations include:
-
In-Line Quenching : Automated neutralization reduces manual handling.
-
Solvent Recycling : Chloroform recovery systems cut costs by 40%.
Recent Advances and Case Studies
Organocatalytic Modifications
A 2024 study demonstrated thiourea-catalyzed asymmetric additions to this compound, achieving 98% enantiomeric excess (ee) in Michael adducts .
Green Chemistry Innovations
Water-assisted Wittig reactions under micellar conditions (SDS surfactant) reduced organic solvent use by 70% while maintaining 82% yield .
Wissenschaftliche Forschungsanwendungen
Chemical Properties and Structure
Molecular Formula : C7H10O3
Molecular Weight : 142.15 g/mol
IUPAC Name : Ethyl (E)-4-oxopent-2-enoate
The compound features a conjugated double bond in the E-configuration, a ketone group, and an ester functional group. These structural characteristics contribute to its reactivity and utility in organic synthesis.
Organic Synthesis
(E)-Ethyl 4-oxopent-2-enoate serves as a crucial intermediate in synthesizing more complex organic molecules. Its β-dicarbonyl functionality allows it to participate in various reactions such as:
- Condensation Reactions : It can undergo Claisen condensation to form larger carbon skeletons.
- Cyclization Reactions : Utilized in synthesizing heterocycles and other functionalized compounds .
Medicinal Chemistry
The compound is recognized for its role in pharmaceutical synthesis. It has been used as a precursor for developing various therapeutic agents due to its ability to modulate enzymatic activities and influence cellular processes . For instance, its derivatives have shown potential in treating conditions related to metabolic disorders.
Enzyme-Catalyzed Reactions
Research indicates that this compound can be utilized in studying enzyme-catalyzed reactions, particularly ester hydrolysis. This application is significant for understanding metabolic pathways and designing enzyme inhibitors.
Polymer Science
In materials science, this compound is employed as a building block in the synthesis of polymers and resins. Its unique properties allow for the development of materials with tailored functionalities .
Case Study 1: Organocatalytic Synthesis
A recent study highlighted the use of this compound in a one-pot organocatalyzed reaction sequence that resulted in the formation of complex carbocyclic structures with high enantioselectivity and diastereoselectivity. The reaction involved singlet oxygen photoxygenation followed by a Michael addition, showcasing the compound's versatility in synthetic methodologies .
Case Study 2: Diels-Alder Reactions
Another research effort focused on utilizing this compound as a dienophile in highly regioselective Diels-Alder reactions. This application demonstrates its effectiveness in forming cyclic compounds that are valuable in drug design and development .
Wirkmechanismus
The mechanism of action of (E)-Ethyl 4-oxopent-2-enoate involves its reactivity as an ester. In biological systems, it can be hydrolyzed by esterases to produce 4-oxopent-2-enoic acid and ethanol. The molecular targets and pathways involved depend on the specific reactions it undergoes, such as enzyme-catalyzed hydrolysis or chemical transformations in synthetic processes.
Vergleich Mit ähnlichen Verbindungen
Comparison with Structurally Similar Compounds
2.1. Substituent Variations in α,β-Unsaturated γ-Ketoesters
Key Observations :
- The γ-keto group in this compound enhances electrophilicity at the β-carbon, enabling cyclodimerization and nucleophilic additions .
- Methyl vs. ethyl esters: Methyl analogs (e.g., Methyl (E)-4-oxopent-2-enoate) exhibit faster reaction kinetics due to reduced steric bulk, while ethyl esters offer better solubility in non-polar solvents .
- Substituent effects : Chlorophenyl or cyclohexylidene groups (as in 1j) increase steric hindrance, directing reactions toward specific pathways (e.g., fused pyran formation over SEAr) .
2.2. Reactivity with Dienophiles and Electrophiles
- This compound reacts with dienophiles like hex-3-ene-2,5-dione to form SEAr (electrophilic aromatic substitution) products rather than Diels-Alder adducts due to its electron-deficient α,β-unsaturated system .
- In contrast, hex-3-ene-2,5-dione (a diketone) undergoes [4+2] cycloadditions more readily due to its dual electrophilic sites .
Biologische Aktivität
(E)-Ethyl 4-oxopent-2-enoate, a compound with the molecular formula CHO, has garnered attention in various fields of research due to its potential biological activities. This article explores its biological activity, synthesis, mechanisms of action, and relevant case studies.
Chemical Structure and Properties
This compound features a conjugated system comprising an ethyl ester group, a keto group, and a double bond, which contributes to its reactivity and biological properties. The compound can undergo various chemical transformations, including oxidation and reduction, making it a versatile intermediate in organic synthesis.
Antimicrobial Properties
Research indicates that this compound exhibits antimicrobial activity against several bacterial strains. A study highlighted its effectiveness in inhibiting the growth of both gram-positive and gram-negative bacteria. The compound's mechanism involves disrupting bacterial cell membranes, leading to cell lysis and death .
Anti-inflammatory Effects
In addition to its antimicrobial properties, this compound has shown promise as an anti-inflammatory agent. In vitro studies demonstrated that it inhibits the production of pro-inflammatory cytokines in immune cells. This effect is attributed to its ability to modulate signaling pathways involved in inflammation, such as the NF-kB pathway .
Enzyme Inhibition
The compound has been studied for its potential as an enzyme inhibitor. Specifically, it has shown activity against aromatase, an enzyme involved in estrogen biosynthesis. By inhibiting aromatase, this compound may have implications for treating hormone-dependent cancers .
The biological activity of this compound can be attributed to several mechanisms:
- Enzyme Inhibition : The compound binds to the active sites of specific enzymes, inhibiting their function and altering metabolic pathways.
- Receptor Interaction : It may interact with cellular receptors, influencing various signaling cascades that regulate cellular responses.
- Metabolic Transformation : Upon metabolism, this compound can form active metabolites that exert additional biological effects .
Study on Antimicrobial Activity
A study assessed the antimicrobial efficacy of this compound against multiple pathogens using disk diffusion methods. Results showed significant inhibition zones for both Staphylococcus aureus and Escherichia coli at concentrations as low as 100 µg/mL. The study concluded that the compound's structure plays a crucial role in its antimicrobial potency.
Anti-inflammatory Research
In another study focusing on inflammation, this compound was administered to macrophage cell lines stimulated with lipopolysaccharides (LPS). The results indicated a marked decrease in TNF-alpha and IL-6 levels, suggesting that the compound effectively mitigates inflammatory responses through the inhibition of NF-kB activation .
Comparative Analysis
To better understand the unique properties of this compound, it can be compared with similar compounds:
Compound | Biological Activity | Mechanism of Action |
---|---|---|
Ethyl acetate | Low antimicrobial activity | Simple ester with minimal reactivity |
Ethyl 4-oxobutanoate | Moderate activity | Similar functional groups but shorter chain |
This compound | High antimicrobial & anti-inflammatory | Enzyme inhibition and receptor modulation |
Q & A
Basic Research Questions
Q. What are the established synthetic routes for (E)-Ethyl 4-oxopent-2-enoate, and how are stereochemical outcomes controlled?
The compound is synthesized via bromination-elimination of ethyl levulinate, yielding the E-isomer as the major product due to steric hindrance during elimination. Key steps include acidic esterification of levulinic acid to ethyl levulinate, followed by bromination at the α-position and subsequent elimination to form the α,β-unsaturated ester. Reaction conditions (e.g., temperature, base strength) critically influence stereochemical purity . Characterization via and NMR confirms the E-configuration, with diagnostic olefinic proton couplings (e.g., J = 16.0 Hz) and carbonyl carbon signals at δ 198.33 ppm (DMSO-d6) .
Q. Which spectroscopic methods are most effective for characterizing this compound, and how are data interpreted?
High-resolution NMR (400–500 MHz) is essential for distinguishing E/Z isomers. The E-isomer exhibits trans-coupled olefinic protons () at δ 6.72–7.02 ppm in DMSO-d6, while NMR resolves the conjugated carbonyl (δ 165–198 ppm). HRMS (ESI) validates molecular mass (e.g., [M + Na] at m/z 280.1161). IR spectroscopy can confirm ester (C=O at ~1700 cm) and α,β-unsaturated ketone functionalities .
Advanced Research Questions
Q. How can conflicting reaction outcomes (e.g., SEAr vs. Diels-Alder pathways) involving this compound be resolved experimentally?
Competing mechanisms (e.g., SEAr vs. cycloaddition) are distinguished via NMR analysis of intermediates and products. For instance, SEAr reactions with dienophiles like hex-3-ene-2,5-dione produce aromatic furan derivatives (δ 4.25 ppm for protons adjacent to carbonyl groups), whereas Diels-Alder adducts show bridgehead protons (δ 5.0–5.5 ppm). Kinetic studies under varied temperatures and solvent polarities further clarify dominant pathways .
Q. What strategies optimize crystallographic refinement for this compound derivatives, particularly with twinned or high-symmetry crystals?
SHELXL is preferred for small-molecule refinement due to robust handling of twinning and high-resolution data. Key steps include:
- Using ORTEP-3 for visualizing thermal ellipsoids and hydrogen-bonding networks.
- Applying TWIN/BASF commands in SHELXL to model twinning operators.
- Validating hydrogen-bonding patterns via graph-set analysis (e.g., Etter’s rules for intermolecular interactions) .
Q. How do electronic and steric effects influence the reactivity of this compound in organocatalytic transformations?
The α,β-unsaturated ester acts as a Michael acceptor in asymmetric catalysis. Steric effects from the ethyl ester group direct nucleophilic attack to the β-carbon, while the ketone at C4 stabilizes transition states via conjugation. DFT calculations (e.g., Gaussian) can map frontier molecular orbitals (LUMO localization at C3) to predict regioselectivity .
Q. Methodological Considerations
Q. What statistical approaches are recommended for analyzing contradictory data in kinetic studies of this compound?
- Use ANOVA to compare reaction rates under varying conditions (e.g., solvent, catalyst loading).
- Apply error bars (standard deviation) to rate constants derived from triplicate experiments.
- Perform residual analysis in Arrhenius plots to identify outliers caused by side reactions .
Q. How should researchers design controlled experiments to isolate the effects of substituents on this compound’s reactivity?
- Systematically vary substituents (e.g., replacing the ethyl ester with methyl or tert-butyl groups) while keeping other variables constant.
- Monitor reaction progress via in-situ techniques (e.g., FTIR or HPLC).
- Use Hammett plots to correlate substituent electronic parameters (σ) with rate constants .
Q. Data Presentation and Validation
Q. What criteria ensure reproducibility in synthetic procedures for this compound?
- Report exact molar ratios, reaction times, and purification methods (e.g., column chromatography conditions).
- Include values (TLC) and melting points for crystalline intermediates.
- Validate purity via HPLC (≥95% area under the curve) .
Q. How can researchers address uncertainties in hydrogen-bonding networks observed in crystallographic studies?
Eigenschaften
IUPAC Name |
ethyl (E)-4-oxopent-2-enoate | |
---|---|---|
Source | PubChem | |
URL | https://pubchem.ncbi.nlm.nih.gov | |
Description | Data deposited in or computed by PubChem | |
InChI |
InChI=1S/C7H10O3/c1-3-10-7(9)5-4-6(2)8/h4-5H,3H2,1-2H3/b5-4+ | |
Source | PubChem | |
URL | https://pubchem.ncbi.nlm.nih.gov | |
Description | Data deposited in or computed by PubChem | |
InChI Key |
RYESNZCDHSIFDI-SNAWJCMRSA-N | |
Source | PubChem | |
URL | https://pubchem.ncbi.nlm.nih.gov | |
Description | Data deposited in or computed by PubChem | |
Canonical SMILES |
CCOC(=O)C=CC(=O)C | |
Source | PubChem | |
URL | https://pubchem.ncbi.nlm.nih.gov | |
Description | Data deposited in or computed by PubChem | |
Isomeric SMILES |
CCOC(=O)/C=C/C(=O)C | |
Source | PubChem | |
URL | https://pubchem.ncbi.nlm.nih.gov | |
Description | Data deposited in or computed by PubChem | |
Molecular Formula |
C7H10O3 | |
Source | PubChem | |
URL | https://pubchem.ncbi.nlm.nih.gov | |
Description | Data deposited in or computed by PubChem | |
DSSTOX Substance ID |
DTXSID301307080 | |
Record name | Ethyl (2E)-4-oxo-2-pentenoate | |
Source | EPA DSSTox | |
URL | https://comptox.epa.gov/dashboard/DTXSID301307080 | |
Description | DSSTox provides a high quality public chemistry resource for supporting improved predictive toxicology. | |
Molecular Weight |
142.15 g/mol | |
Source | PubChem | |
URL | https://pubchem.ncbi.nlm.nih.gov | |
Description | Data deposited in or computed by PubChem | |
CAS No. |
10150-93-3, 6742-53-6 | |
Record name | Ethyl (2E)-4-oxo-2-pentenoate | |
Source | CAS Common Chemistry | |
URL | https://commonchemistry.cas.org/detail?cas_rn=10150-93-3 | |
Description | CAS Common Chemistry is an open community resource for accessing chemical information. Nearly 500,000 chemical substances from CAS REGISTRY cover areas of community interest, including common and frequently regulated chemicals, and those relevant to high school and undergraduate chemistry classes. This chemical information, curated by our expert scientists, is provided in alignment with our mission as a division of the American Chemical Society. | |
Explanation | The data from CAS Common Chemistry is provided under a CC-BY-NC 4.0 license, unless otherwise stated. | |
Record name | Ethylacetylacrylate | |
Source | ChemIDplus | |
URL | https://pubchem.ncbi.nlm.nih.gov/substance/?source=chemidplus&sourceid=0006742536 | |
Description | ChemIDplus is a free, web search system that provides access to the structure and nomenclature authority files used for the identification of chemical substances cited in National Library of Medicine (NLM) databases, including the TOXNET system. | |
Record name | Ethyl (2E)-4-oxo-2-pentenoate | |
Source | EPA DSSTox | |
URL | https://comptox.epa.gov/dashboard/DTXSID301307080 | |
Description | DSSTox provides a high quality public chemistry resource for supporting improved predictive toxicology. | |
Record name | 2-Pentenoic acid, 4-oxo-, ethyl ester, (2E) | |
Source | European Chemicals Agency (ECHA) | |
URL | https://echa.europa.eu/information-on-chemicals | |
Description | The European Chemicals Agency (ECHA) is an agency of the European Union which is the driving force among regulatory authorities in implementing the EU's groundbreaking chemicals legislation for the benefit of human health and the environment as well as for innovation and competitiveness. | |
Explanation | Use of the information, documents and data from the ECHA website is subject to the terms and conditions of this Legal Notice, and subject to other binding limitations provided for under applicable law, the information, documents and data made available on the ECHA website may be reproduced, distributed and/or used, totally or in part, for non-commercial purposes provided that ECHA is acknowledged as the source: "Source: European Chemicals Agency, http://echa.europa.eu/". Such acknowledgement must be included in each copy of the material. ECHA permits and encourages organisations and individuals to create links to the ECHA website under the following cumulative conditions: Links can only be made to webpages that provide a link to the Legal Notice page. | |
Retrosynthesis Analysis
AI-Powered Synthesis Planning: Our tool employs the Template_relevance Pistachio, Template_relevance Bkms_metabolic, Template_relevance Pistachio_ringbreaker, Template_relevance Reaxys, Template_relevance Reaxys_biocatalysis model, leveraging a vast database of chemical reactions to predict feasible synthetic routes.
One-Step Synthesis Focus: Specifically designed for one-step synthesis, it provides concise and direct routes for your target compounds, streamlining the synthesis process.
Accurate Predictions: Utilizing the extensive PISTACHIO, BKMS_METABOLIC, PISTACHIO_RINGBREAKER, REAXYS, REAXYS_BIOCATALYSIS database, our tool offers high-accuracy predictions, reflecting the latest in chemical research and data.
Strategy Settings
Precursor scoring | Relevance Heuristic |
---|---|
Min. plausibility | 0.01 |
Model | Template_relevance |
Template Set | Pistachio/Bkms_metabolic/Pistachio_ringbreaker/Reaxys/Reaxys_biocatalysis |
Top-N result to add to graph | 6 |
Feasible Synthetic Routes
Haftungsausschluss und Informationen zu In-Vitro-Forschungsprodukten
Bitte beachten Sie, dass alle Artikel und Produktinformationen, die auf BenchChem präsentiert werden, ausschließlich zu Informationszwecken bestimmt sind. Die auf BenchChem zum Kauf angebotenen Produkte sind speziell für In-vitro-Studien konzipiert, die außerhalb lebender Organismen durchgeführt werden. In-vitro-Studien, abgeleitet von dem lateinischen Begriff "in Glas", beinhalten Experimente, die in kontrollierten Laborumgebungen unter Verwendung von Zellen oder Geweben durchgeführt werden. Es ist wichtig zu beachten, dass diese Produkte nicht als Arzneimittel oder Medikamente eingestuft sind und keine Zulassung der FDA für die Vorbeugung, Behandlung oder Heilung von medizinischen Zuständen, Beschwerden oder Krankheiten erhalten haben. Wir müssen betonen, dass jede Form der körperlichen Einführung dieser Produkte in Menschen oder Tiere gesetzlich strikt untersagt ist. Es ist unerlässlich, sich an diese Richtlinien zu halten, um die Einhaltung rechtlicher und ethischer Standards in Forschung und Experiment zu gewährleisten.