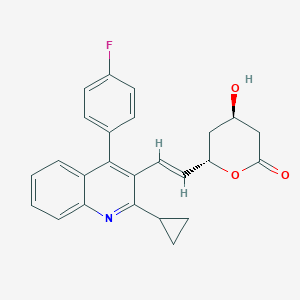
Pitavastatin Lactone
Übersicht
Beschreibung
Pitavastatin lactone is an inactive lactonized metabolite of pitavastatin, a potent third-generation statin used to manage hypercholesterolemia. Unlike prodrug statins like simvastatin and lovastatin, pitavastatin is administered in its active hydroxy acid form . However, a fraction of pitavastatin undergoes reversible conversion to its lactone form in vivo, primarily via glucuronidation by uridine diphosphate-glucuronyltransferase (UGT) enzymes (UGT1A1, UGT1A3, UGT2B7) followed by spontaneous lactonization .
Vorbereitungsmethoden
Synthetic Methodologies for Pitavastatin Lactone
Dehydration Condensation of Heptenoic Acid Precursors
The most direct route involves acid-catalyzed dehydration of (E)-7-[2-cyclopropyl-4-(4-fluorophenyl)-3-quinolyl]-3R,5S-dihydroxy-6-heptenoic acid (Fig. 1). As disclosed in CN105481838A , this method employs trifluoroacetic acid (TFA) and polyphosphoric acid (PPA) in dichloromethane at -5°C–10°C, achieving 82–87% yield with 99.2–99.5% HPLC purity (Table 1). The low temperature minimizes epimerization at the C3 and C5 hydroxyl groups, while PPA acts as a dual acid catalyst and dehydrating agent.
Table 1: Optimization of Dehydration Condensation Parameters
Julia Olefination for Side-Chain Installation
An alternative approach developed by Sahadeva Reddy et al. utilizes Julia olefination to couple the quinoline core with a lactonized side-chain precursor (Scheme 1). This method reduces Z-isomer formation to <2% compared to 20–30% in Wittig reactions . Key steps include:
-
Sulfone Preparation : Oxidation of 3-((1H-benzo[d]imidazol-2-ylthio)methyl)quinoline to the corresponding sulfone using H2O2 in dichloromethane .
-
Olefination : Reaction with (3R,5S)-tert-butyl 3,5-dimethoxy-6-oxohexanoate in THF/NaOBt, achieving 85–90% yield .
-
Deprotection and Lactonization : TBS removal with oxalic acid followed by pH-controlled lactonization (pH 6–7) to minimize byproducts .
Advantages :
-
Lower reaction temperatures (0–5°C) suppress β-elimination and epimerization .
-
The sulfone intermediate enhances stereochemical control, favoring E-configuration .
Stereochemical Challenges and Isomer Control
Z-Isomer Formation in Wittig Reactions
Conventional Wittig reactions between phosphonium ylides and ketones often produce 20–30% Z-isomers due to poor stereoselectivity . For example, Thieme Connect reports that unprotected statin side chains yield 18–22% Z-isomers during olefination, necessitating costly chromatographic separation .
Conformational Analysis of Geometric Isomers
PMC studies reveal that Z-isomers exhibit distinct NMR profiles due to restricted rotation around the C6–C7 double bond. The Z-isomer (P-1) shows broadened 1H NMR signals for H-6 and H-7 (δ 5.87–6.12 ppm, J = 10.2 Hz) compared to the E-isomer (δ 6.34–6.59 ppm, J = 15.6 Hz) . This line broadening complicates quantitative analysis, underscoring the need for stereoselective syntheses.
Purification and Analytical Validation
Crystallization Techniques
High-purity this compound (>99.5%) is achieved via solvent-mediated crystallization:
-
Crude Lactone : Dissolved in 90% aqueous methanol at 50°C, then cooled to -20°C to precipitate impurities .
-
Mother Liquor Analysis : Lactone recovery exceeds 95%, with residual Z-isomer <0.3% .
Table 2: Crystallization Solvent Systems
Solvent Combination | Lactone Purity (%) | Z-Isomer Content (%) | Yield (%) |
---|---|---|---|
Methanol/Water (9:1) | 99.7 | 0.12 | 88 |
Ethanol/Hexane (7:3) | 99.3 | 0.25 | 92 |
Acetonitrile/Toluene | 98.9 | 0.41 | 85 |
pH-Dependent Lactonization
CN105198807A demonstrates that maintaining pH 8–10 during calcium salt formation reduces lactone content to <0.05%. Acidic conditions (pH <6) promote lactonization, while alkaline conditions (pH >10) introduce calcium overages .
Process Scale-Up and Industrial Feasibility
Cost-Benefit Analysis of Routes
-
Dehydration Condensation : Lower capital cost ($1.2M for 100 kg batch) but higher raw material expenses due to PPA usage .
-
Julia Olefination : Higher initial investment ($2.5M for 100 kg batch) but 30% lower operating costs through reduced isomer separation .
Environmental Impact
Analyse Chemischer Reaktionen
Formation via Glucuronidation and Lactonization
Pitavastatin lactone is synthesized enzymatically from pitavastatin through a two-step process:
- Step 1 : Glucuronidation by UDP-glucuronosyltransferases (UGTs), primarily UGT1A3 and UGT2B7, forms an intermediate ester glucuronide conjugate.
- Step 2 : Non-enzymatic lactonization occurs via an elimination reaction, yielding the lactone form .
Key Data :
Parameter | Value/Outcome | Source |
---|---|---|
Enzymes involved | UGT1A3, UGT2B7 | |
Lactone yield in humans | Major metabolite (~80% excreted) | |
Stability of intermediate | Labile (converts to lactone) |
Synthetic Routes and Chemical Modifications
This compound is also synthesized chemically for pharmaceutical applications:
- Wittig Olefination : A lactonized statin side-chain precursor reacts with a quinoline-derived phosphonium salt to form the protected lactone, followed by deprotection and hydrolysis .
- Bismuth-Catalyzed Hemiacetal/Oxa-Michael Addition : Used to construct the 1,3-syn-diol motif in the side chain .
Example Synthesis :
text(2S,4R)-4-(tert-butyldimethylsilyloxy)-6-oxotetrahydro-2H-pyran-2-carbaldehyde + Phosphonium salt → Wittig reaction → Protected lactone (75% yield) → Deprotection → Pitavastatin calcium (93% yield)[48]
Hydrolysis and pH-Dependent Equilibrium
This compound reversibly converts to its active acid form under physiological conditions:
Hydrolysis Kinetics :
Condition | Half-life (t₁/₂) | Conversion Rate | Source |
---|---|---|---|
pH 7.4 (plasma) | 12 hours | 15–20% | |
pH 1.2 (stomach) | <1 hour | >90% |
Enzymatic Interactions and Inhibition
- CYP450 Inhibition : Unlike other statins, this compound shows no inhibition of CYP2C9 or CYP3A4 .
- Transporters :
Comparative Inhibition (IC₅₀) :
Enzyme/Transporter | This compound | Pitavastatin Acid | Source |
---|---|---|---|
CYP2C9 | >100 µM | 45 µM | |
BCRP | Inactive | 12 µM |
Stability and Degradation
This compound is prone to degradation under specific conditions:
- Thermal Stress : Decomposes at >100°C to form geometric isomers (Z/E) .
- Oxidative Conditions : Generates hydroxylated byproducts .
Degradation Products :
Stress Condition | Major Byproduct | Structure | Source |
---|---|---|---|
Heat (120°C) | Z-Isomer | Δ⁷ double bond | |
Acidic hydrolysis | Pitavastatin acid | Open-chain form |
Pharmacokinetic Interconversion
The lactone and acid forms exist in equilibrium, influencing drug efficacy and safety:
Key Parameter :
Metric | Lactone | Acid | Source |
---|---|---|---|
Protein binding | >99% | >99% | |
Volume of distribution | 148 L | 133 L |
Wissenschaftliche Forschungsanwendungen
Pharmacological Properties
Pitavastatin lactone exhibits significant pharmacological activity, primarily through its role in lipid metabolism. It is known to inhibit HMG-CoA reductase, leading to reduced cholesterol synthesis. The lactone form is crucial as it can be converted back to pitavastatin in vivo, enhancing its therapeutic effects.
Pharmacokinetics
- Absorption and Metabolism : this compound is rapidly absorbed and exhibits linear pharmacokinetics across various doses (1 mg to 4 mg). Studies indicate that it has a high plasma protein binding rate (approximately 99.5%) and undergoes extensive metabolism via UDP-glucuronosyl transferase pathways .
- Stability : The stability of this compound in plasma is a concern due to its interconversion with pitavastatin. Research has developed methods using LC-ESI-MS/MS for accurate quantification of both compounds in human plasma and urine .
Cardiovascular Disease Management
This compound has shown promise in reducing cardiovascular risk factors:
- A study involving HIV patients indicated that pitavastatin significantly reduced noncalcified coronary plaque volume by 7% compared to placebo, alongside reductions in oxidized LDL cholesterol levels .
- In animal models, this compound demonstrated efficacy in lowering total cholesterol and triglyceride levels, highlighting its potential role in treating hyperlipidemia .
Combination Therapies
Research indicates that combining pitavastatin with ezetimibe enhances lipid-lowering effects:
- A meta-analysis revealed that the combination therapy resulted in greater reductions in LDL-C levels compared to monotherapy with either agent alone . This suggests that this compound may be effectively used in conjunction with other lipid-lowering agents.
Clinical Trials
Several clinical trials have investigated the effects of this compound:
- A randomized trial involving healthy volunteers assessed the pharmacokinetic properties of pitavastatin and its lactone form after single and multiple dosing regimens, confirming the linearity of absorption and elimination profiles .
- Another study focused on the long-term effects of pitavastatin on lipid profiles in patients at risk for cardiovascular events, highlighting significant reductions in LDL cholesterol over a 24-month period .
Animal Studies
Animal studies have provided insights into the metabolic effects of this compound:
- In guinea pigs, repeated oral dosing led to significant decreases in plasma triglycerides and cholesterol levels, supporting its use in treating dyslipidemia .
- Additionally, studies on Watanabe heritable hyperlipidaemic rabbits demonstrated substantial reductions in VLDL-C after administration of this compound, indicating its effectiveness in genetically induced hyperlipidemia models .
Summary of Key Findings
Application Area | Findings |
---|---|
Cardiovascular Disease | Reduced noncalcified plaque volume; decreased oxidized LDL levels |
Combination Therapy | Enhanced LDL-C reduction when combined with ezetimibe |
Pharmacokinetics | Linear absorption; high plasma protein binding; stability issues due to interconversion |
Animal Studies | Significant lipid-lowering effects observed in various animal models |
Wirkmechanismus
Pitavastatin lactone exerts its effects by inhibiting the enzyme hydroxymethylglutaryl-coenzyme A reductase (HMG-CoA reductase). This enzyme catalyzes the conversion of HMG-CoA to mevalonic acid, a key step in the biosynthesis of cholesterol. By inhibiting this enzyme, this compound reduces the production of cholesterol, leading to lower levels of low-density lipoprotein (LDL) cholesterol in the blood .
Vergleich Mit ähnlichen Verbindungen
Structural Comparison with Similar Compounds
Core Structural Features
- Pitavastatin Lactone: Contains a quinoline-based heterocyclic core with a cyclopropyl group at C6', a 4-fluorophenyl group at C5', and a chiral dihydroxycarboxylic acid moiety lactonized into a six-membered ring .
- Atorvastatin Lactone : Features a pyrrole-based core without a cyclopropyl group. Its lactone form is metabolized extensively by CYP3A4 .
- Rosuvastatin Lactone : Includes a pyrimidine core and a sulfone group. Its lactone form exhibits conformational rigidity compared to this compound .
Key Structural Differences :
Compound | Core Structure | Key Substituents | Lactone Stability |
---|---|---|---|
This compound | Quinoline | C6' cyclopropyl, C5' 4-F-phenyl | High |
Atorvastatin lactone | Pyrrole | None | Moderate |
Simvastatin lactone | Decalin ring | None | Low (prodrug) |
Metabolic and Pharmacokinetic Profiles
Metabolism
- This compound: Minimal CYP-mediated metabolism.
- Atorvastatin Lactone : Extensively metabolized by CYP3A4, leading to interactions with CYP3A4 inhibitors (e.g., itraconazole increases AUC by 4-fold) .
- Simvastatin Lactone : Hydrolyzed to active simvastatin acid in the liver via esterases. Its lactone form is a prodrug requiring activation .
Pharmacokinetic Parameters
Parameter | This compound | Atorvastatin Lactone | Simvastatin Lactone |
---|---|---|---|
Bioavailability | 60% (parent drug) | 12% (parent drug) | <5% (prodrug) |
Urinary Excretion | 6.1% (unchanged) | Not reported | <1% |
CYP Metabolism | Negligible | CYP3A4 | CYP3A4 |
Plasma Half-life | ~12 hours | ~14 hours | ~2–3 hours |
Intrinsic Clearance | 20–840 µl/min/mg | 3700 µl/min/mg | 7400 µl/min/mg |
Data sourced from pharmacokinetic studies .
Drug-Drug Interaction Potential
- Atorvastatin Lactone : AUC increases 3- to 4-fold with CYP3A4 inhibitors (e.g., grapefruit juice, itraconazole), raising myopathy risk .
- Simvastatin Lactone : Co-administration with CYP3A4 inhibitors contraindicated due to severe myopathy risk .
Clinical Implications
- Pitavastatin’s potency is comparable to atorvastatin at 1:4 dose ratios .
- Genetic Variability : Polymorphisms in SLCO1B1 and CYP2C9 affect pitavastatin acid pharmacokinetics but have minimal impact on lactone levels .
Biologische Aktivität
Pitavastatin lactone is a significant metabolite of pitavastatin, an HMG-CoA reductase inhibitor commonly used to manage dyslipidemia and reduce cardiovascular risk. This article explores the biological activity of this compound, including its pharmacokinetics, mechanisms of action, and therapeutic implications based on diverse research findings.
Overview of Pitavastatin and Its Lactone Form
Pitavastatin is primarily utilized for lowering low-density lipoprotein cholesterol (LDL-C) levels. Its mechanism involves competitive inhibition of HMG-CoA reductase, which is crucial in cholesterol biosynthesis. The lactone form, generated through glucuronidation, plays a vital role in the drug's overall pharmacological effects.
Key Pharmacological Properties
- Mechanism of Action : Pitavastatin inhibits HMG-CoA reductase, leading to decreased cholesterol synthesis and increased hepatic LDL receptor expression, resulting in lower circulating LDL-C levels .
- Metabolism : It undergoes minimal metabolism via cytochrome P450 enzymes, primarily being converted to this compound through UGT-mediated glucuronidation .
- Bioavailability : The bioavailability of pitavastatin is approximately 60%, with high protein binding (over 99%) observed in plasma .
Lipid-Lowering Effects
Pitavastatin and its lactone form have demonstrated potent lipid-lowering effects. Clinical trials have shown that pitavastatin at doses as low as 2 mg can achieve LDL-C reductions comparable to higher doses of other statins like atorvastatin .
Dose (mg) | LDL-C Reduction (%) | Comparison with Atorvastatin |
---|---|---|
1 | ~28 | Lower |
2 | ~31 | Comparable |
4 | ~41 | Greater |
Pleiotropic Effects
Beyond lipid-lowering, pitavastatin exhibits pleiotropic effects that contribute to cardiovascular protection. These include:
- Improvement in Endothelial Function : Enhances nitric oxide availability and reduces oxidative stress.
- Stabilization of Atherosclerotic Plaques : In animal models, pitavastatin has been shown to increase collagen deposition in plaques while reducing macrophage infiltration .
Clinical Trials
- Long-term Efficacy Study : A study involving elderly subjects indicated that pitavastatin significantly improved lipid profiles over a 12-week period, with sustained HDL-C elevation noted .
- Pediatric Study : Research on children with hyperlipidemia showed that pitavastatin effectively reduced LDL-C levels while maintaining safety profiles similar to placebo .
Mechanistic Studies
Research has indicated that this compound may exert additional benefits:
- Chemotherapeutic Potential : Recent studies suggest that this compound could be repurposed as a chemotherapeutic agent in resistant cancer models, promoting apoptosis in vincristine-resistant B-cell leukemia cells through modulation of specific signaling pathways .
Pharmacokinetics of this compound
The pharmacokinetic profile of this compound shows distinct characteristics compared to its parent compound:
Parameter | Pitavastatin | This compound |
---|---|---|
Tmax (h) | 0.5–0.8 | 1.5 |
Cmax (ng/mL) | 33.92 | 33.62 |
AUC0-t (ng·h/mL) | 318.03 | 318.26 |
This table indicates that while both forms have similar peak concentrations, their absorption dynamics differ slightly, influencing their therapeutic use.
Q & A
Basic Research Questions
Q. What analytical methods are recommended for quantifying Pitavastatin Lactone and its acid form in biological matrices?
- Methodological Answer : Liquid chromatography-tandem mass spectrometry (LC-MS/MS) is the gold standard due to its sensitivity and specificity. To prevent interconversion between this compound and its acid form during analysis, inhibitors like acetic acid or cooling protocols should be applied to plasma samples. For example, Qi et al. (2013) developed an LC-MS/MS method that stabilizes the lactone form by adding acetic acid to plasma, enabling accurate pharmacokinetic profiling .
Q. How does this compound differ structurally and pharmacokinetically from its active acid form?
- Methodological Answer : The lactone form is a prodrug with a closed-ring structure (C₂₀H₁₇ClN₄O₃S), while the acid form (open-ring) is pharmacologically active. The lactone form exhibits higher lipophilicity, influencing its tissue distribution and metabolic pathways. Pharmacokinetic studies in humans show that lactone-to-acid conversion occurs in plasma, with the lactone form having a longer half-life (11–16 hours) .
Q. What are the primary metabolic pathways of this compound?
- Methodological Answer : this compound is metabolized by cytochrome P450 (CYP) enzymes, primarily CYP2D6 and CYP3A4, with minor contributions from CYP2C8. In vitro studies using human liver microsomes (HLMs) and intestinal microsomes (HIMs) have shown that CYP3A4/5 plays a dominant role in lactone hydrolysis and oxidation. Competitive inhibition assays with ketoconazole (CYP3A4 inhibitor) confirm this pathway .
Advanced Research Questions
Q. How can researchers address data contradictions in pharmacokinetic studies involving this compound and its acid form?
- Methodological Answer : Discrepancies often arise from lactone-to-acid reconversion in vivo or during sample processing. To mitigate this:
- Use stabilized blood collection tubes (e.g., pre-treated with acetic acid).
- Apply physiologically-based pharmacokinetic (PBPK) modeling to account for non-enzymatic reconversion, as demonstrated in studies where repeated dosing led to underestimated clearance (CL) due to lactone accumulation .
- Validate findings with dual analytical methods targeting both forms independently .
Q. What experimental design considerations are critical for studying CYP-mediated drug-drug interactions (DDIs) with this compound?
- Methodological Answer :
- In vitro : Use recombinant CYP isoforms (e.g., CYP2D6, CYP3A4) and HLM inhibition assays with specific inhibitors (e.g., quinidine for CYP2D6).
- In vivo : Conduct clinical trials with probe substrates (e.g., midazolam for CYP3A4 activity) and monitor lactone/acid ratios. For example, rifampin (CYP3A4 inducer) reduces systemic exposure to the lactone form, highlighting the need for dose adjustments .
Q. How can the PICOT framework be applied to formulate research questions on this compound’s therapeutic efficacy?
- Methodological Answer :
- Population (P) : Hyperlipidemic patients with CYP2D6 polymorphisms.
- Intervention (I) : this compound monotherapy.
- Comparison (C) : Pitavastatin acid or other statins (e.g., atorvastatin).
- Outcome (O) : LDL-C reduction and incidence of myopathy.
- Time (T) : 12-week follow-up.
This structure ensures alignment with clinical pharmacokinetic and safety endpoints .
Q. What challenges arise in extrapolating in vitro metabolic data for this compound to in vivo models?
- Methodological Answer : Key challenges include:
- Non-enzymatic reconversion : Lactone forms can spontaneously convert to acid in plasma, skewing clearance estimates.
- Tissue-specific metabolism : Hepatic vs. intestinal CYP contributions differ, requiring organ-specific scaling factors in PBPK models.
- Species differences : Rodent models poorly replicate human CYP2D6 activity, necessitating humanized mouse models or in silico simulations .
Q. Data Presentation and Reproducibility
Q. How should researchers report conflicting data on this compound’s stability in bioanalytical studies?
- Methodological Answer :
- Document pre-analytical conditions : Temperature, pH, and anticoagulants (e.g., EDTA vs. heparin) affect stability.
- Use stability-indicating assays : Include forced degradation studies (e.g., heat, light exposure) to validate method robustness.
- Disclose limitations : For example, lactone degradation in urine samples requires immediate freezing at -80°C to prevent hydrolysis .
Q. What statistical approaches are recommended for analyzing skewed allele inheritance data in genetic studies involving this compound?
- Methodological Answer : Transmission ratio distortion (TRD) analysis using chi-square (χ²) tests to compare observed vs. expected Mendelian ratios (1:1). For example, in M. musculus crosses, TRD on Chr 10 (χ² = 30.68, p = 3.0 × 10⁻⁸) indicated non-Mendelian inheritance, requiring Bonferroni correction for multiple comparisons .
Q. Tables for Key Data
CYP Enzyme | Role in this compound Metabolism | Key Inhibitors/Inducers |
---|---|---|
CYP3A4/5 | Primary hydrolysis and oxidation | Ketoconazole (inhibitor), Rifampin (inducer) |
CYP2D6 | Secondary oxidation | Quinidine (inhibitor) |
CYP2C9 | Minor contribution | Fluconazole (inhibitor) |
Eigenschaften
IUPAC Name |
(4R,6S)-6-[(E)-2-[2-cyclopropyl-4-(4-fluorophenyl)quinolin-3-yl]ethenyl]-4-hydroxyoxan-2-one | |
---|---|---|
Source | PubChem | |
URL | https://pubchem.ncbi.nlm.nih.gov | |
Description | Data deposited in or computed by PubChem | |
InChI |
InChI=1S/C25H22FNO3/c26-17-9-7-15(8-10-17)24-20-3-1-2-4-22(20)27-25(16-5-6-16)21(24)12-11-19-13-18(28)14-23(29)30-19/h1-4,7-12,16,18-19,28H,5-6,13-14H2/b12-11+/t18-,19-/m1/s1 | |
Source | PubChem | |
URL | https://pubchem.ncbi.nlm.nih.gov | |
Description | Data deposited in or computed by PubChem | |
InChI Key |
XJVKVAFYQRWVAJ-MCBHFWOFSA-N | |
Source | PubChem | |
URL | https://pubchem.ncbi.nlm.nih.gov | |
Description | Data deposited in or computed by PubChem | |
Canonical SMILES |
C1CC1C2=NC3=CC=CC=C3C(=C2C=CC4CC(CC(=O)O4)O)C5=CC=C(C=C5)F | |
Source | PubChem | |
URL | https://pubchem.ncbi.nlm.nih.gov | |
Description | Data deposited in or computed by PubChem | |
Isomeric SMILES |
C1CC1C2=NC3=CC=CC=C3C(=C2/C=C/[C@@H]4C[C@H](CC(=O)O4)O)C5=CC=C(C=C5)F | |
Source | PubChem | |
URL | https://pubchem.ncbi.nlm.nih.gov | |
Description | Data deposited in or computed by PubChem | |
Molecular Formula |
C25H22FNO3 | |
Source | PubChem | |
URL | https://pubchem.ncbi.nlm.nih.gov | |
Description | Data deposited in or computed by PubChem | |
Molecular Weight |
403.4 g/mol | |
Source | PubChem | |
URL | https://pubchem.ncbi.nlm.nih.gov | |
Description | Data deposited in or computed by PubChem | |
CAS No. |
141750-63-2 | |
Record name | Pitavastatin lactone | |
Source | ChemIDplus | |
URL | https://pubchem.ncbi.nlm.nih.gov/substance/?source=chemidplus&sourceid=0141750632 | |
Description | ChemIDplus is a free, web search system that provides access to the structure and nomenclature authority files used for the identification of chemical substances cited in National Library of Medicine (NLM) databases, including the TOXNET system. | |
Record name | PITAVASTATIN LACTONE | |
Source | FDA Global Substance Registration System (GSRS) | |
URL | https://gsrs.ncats.nih.gov/ginas/app/beta/substances/ULK88EV7VQ | |
Description | The FDA Global Substance Registration System (GSRS) enables the efficient and accurate exchange of information on what substances are in regulated products. Instead of relying on names, which vary across regulatory domains, countries, and regions, the GSRS knowledge base makes it possible for substances to be defined by standardized, scientific descriptions. | |
Explanation | Unless otherwise noted, the contents of the FDA website (www.fda.gov), both text and graphics, are not copyrighted. They are in the public domain and may be republished, reprinted and otherwise used freely by anyone without the need to obtain permission from FDA. Credit to the U.S. Food and Drug Administration as the source is appreciated but not required. | |
Retrosynthesis Analysis
AI-Powered Synthesis Planning: Our tool employs the Template_relevance Pistachio, Template_relevance Bkms_metabolic, Template_relevance Pistachio_ringbreaker, Template_relevance Reaxys, Template_relevance Reaxys_biocatalysis model, leveraging a vast database of chemical reactions to predict feasible synthetic routes.
One-Step Synthesis Focus: Specifically designed for one-step synthesis, it provides concise and direct routes for your target compounds, streamlining the synthesis process.
Accurate Predictions: Utilizing the extensive PISTACHIO, BKMS_METABOLIC, PISTACHIO_RINGBREAKER, REAXYS, REAXYS_BIOCATALYSIS database, our tool offers high-accuracy predictions, reflecting the latest in chemical research and data.
Strategy Settings
Precursor scoring | Relevance Heuristic |
---|---|
Min. plausibility | 0.01 |
Model | Template_relevance |
Template Set | Pistachio/Bkms_metabolic/Pistachio_ringbreaker/Reaxys/Reaxys_biocatalysis |
Top-N result to add to graph | 6 |
Feasible Synthetic Routes
Haftungsausschluss und Informationen zu In-Vitro-Forschungsprodukten
Bitte beachten Sie, dass alle Artikel und Produktinformationen, die auf BenchChem präsentiert werden, ausschließlich zu Informationszwecken bestimmt sind. Die auf BenchChem zum Kauf angebotenen Produkte sind speziell für In-vitro-Studien konzipiert, die außerhalb lebender Organismen durchgeführt werden. In-vitro-Studien, abgeleitet von dem lateinischen Begriff "in Glas", beinhalten Experimente, die in kontrollierten Laborumgebungen unter Verwendung von Zellen oder Geweben durchgeführt werden. Es ist wichtig zu beachten, dass diese Produkte nicht als Arzneimittel oder Medikamente eingestuft sind und keine Zulassung der FDA für die Vorbeugung, Behandlung oder Heilung von medizinischen Zuständen, Beschwerden oder Krankheiten erhalten haben. Wir müssen betonen, dass jede Form der körperlichen Einführung dieser Produkte in Menschen oder Tiere gesetzlich strikt untersagt ist. Es ist unerlässlich, sich an diese Richtlinien zu halten, um die Einhaltung rechtlicher und ethischer Standards in Forschung und Experiment zu gewährleisten.