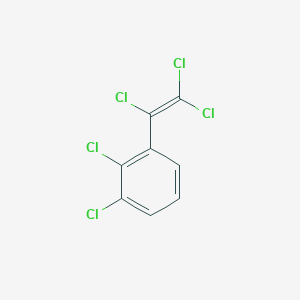
Pentachlorostyrene
Übersicht
Beschreibung
Pentachlorostyrene is a halogenated aromatic compound with the molecular formula C8H3Cl5. It is characterized by the presence of five chlorine atoms attached to a benzene ring, along with a vinyl group. This compound is known for its stability and resistance to degradation, making it a subject of interest in various scientific fields .
Vorbereitungsmethoden
Synthetic Routes and Reaction Conditions: Pentachlorostyrene can be synthesized through the dehydrohalogenation of betachloroethylpentachlorobenzene or by dehydrating methylpentachlorophenyl carbinol. The process involves heating the reactants in the presence of a catalyst such as benzoyl peroxide. The reaction typically takes place in a solvent like carbon tetrachloride, and the mixture is heated until reflux temperature is reached .
Industrial Production Methods: In industrial settings, the production of this compound follows similar synthetic routes but on a larger scale. The use of continuous reactors and optimized reaction conditions ensures higher yields and purity of the final product. The industrial process also involves rigorous purification steps to remove any impurities and by-products .
Analyse Chemischer Reaktionen
Types of Reactions: Pentachlorostyrene undergoes various chemical reactions, including:
Oxidation: This reaction typically involves the use of strong oxidizing agents, leading to the formation of chlorinated benzoic acids.
Reduction: Reduction reactions can be carried out using reducing agents like lithium aluminum hydride, resulting in the formation of less chlorinated derivatives.
Common Reagents and Conditions:
Oxidation: Potassium permanganate or chromium trioxide in acidic conditions.
Reduction: Lithium aluminum hydride in anhydrous ether.
Substitution: Sodium hydroxide or ammonia in aqueous or alcoholic solutions.
Major Products Formed:
Oxidation: Chlorinated benzoic acids.
Reduction: Less chlorinated styrene derivatives.
Substitution: Hydroxylated or aminated styrene derivatives.
Wissenschaftliche Forschungsanwendungen
Material Science Applications
Polymer Synthesis:
Pentachlorostyrene serves as a valuable monomer in the synthesis of high-performance polymers. Its incorporation into copolymers enhances thermal stability and chemical resistance. For instance, poly(pentafluorostyrene) has been studied for its dielectric properties in organic thin-film transistors (OTFTs). The copolymerization of pentafluorostyrene with other monomers such as methyl methacrylate has shown tunable surface properties, which are crucial for device performance in electronics .
Table 1: Properties of Poly(pentafluorostyrene) Copolymers
Property | Value |
---|---|
Glass Transition Temperature | 120 °C |
Dielectric Constant | 3.2 |
Field-Effect Mobility | Up to 0.5 cm²/V·s |
Thermal Stability | Stable up to 300 °C |
Electronics and Electrical Engineering
Dielectric Materials:
this compound-based polymers are explored as dielectric materials due to their low dielectric constants and high breakdown voltages. These materials are essential in the fabrication of capacitors and insulators for electronic devices. The copolymerization process allows for the fine-tuning of electrical properties, making them suitable for advanced electronic applications .
Case Study: Organic Thin-Film Transistors (OTFTs)
A study demonstrated that the incorporation of pentafluorostyrene into OTFTs significantly improved the field-effect mobility of the devices compared to traditional polymers. This enhancement is attributed to the optimized surface properties and reduced charge trapping in the dielectric layer .
Environmental Applications
Remediation Technologies:
this compound has been investigated for its potential role in environmental remediation technologies, particularly in addressing contamination from chlorinated compounds. Its chemical stability allows it to be used as a tracer or marker in soil and groundwater studies, aiding in the assessment of pollution sources and pathways .
Table 2: Environmental Impact Assessment of this compound
Parameter | Value |
---|---|
Solubility in Water | Immiscible |
Persistence | High |
Bioaccumulation Potential | Moderate |
Biomedical Applications
Fluorinated Nanoparticles:
Research indicates that this compound can be utilized in the synthesis of fluorinated nanoparticles for biomedical imaging applications, such as magnetic resonance imaging (MRI). These nanoparticles exhibit larger structural design potentials compared to traditional systems, enhancing imaging contrast and resolution .
Case Study: MRI Contrast Agents
Fluorinated nanoparticles synthesized from this compound have shown promise as contrast agents in MRI due to their unique magnetic properties and stability in biological environments. Studies have reported improved imaging quality when using these novel agents compared to conventional contrast materials .
Wirkmechanismus
The mechanism of action of pentachlorostyrene involves its interaction with cellular components, leading to various biochemical effects. The compound can disrupt cellular membranes and interfere with enzyme activities, particularly those involved in oxidative stress responses. Its high chlorine content contributes to its reactivity and ability to form stable complexes with biological molecules .
Vergleich Mit ähnlichen Verbindungen
Tetrachlorostyrene: Contains one less chlorine atom, leading to different reactivity and applications.
Hexachlorostyrene: Contains one more chlorine atom, making it more stable but less reactive.
Polychlorinated biphenyls (PCBs): Structurally similar but with different applications and environmental impacts.
Uniqueness: Pentachlorostyrene stands out due to its balance of stability and reactivity, making it suitable for a wide range of applications. Its unique structure allows for specific interactions with biological and chemical systems, distinguishing it from other chlorinated aromatic compounds .
Biologische Aktivität
Pentachlorostyrene (PCS) is a chlorinated aromatic compound that has garnered attention due to its potential biological activity and environmental impact. This article provides a comprehensive overview of the biological effects, toxicity, and environmental behavior of PCS, supported by data tables and case studies.
This compound is characterized by the presence of five chlorine atoms attached to a styrene backbone. Its chemical formula is C8HCl5, and it is known for its stability and persistence in the environment. The chlorination enhances its lipophilicity, which can lead to bioaccumulation in aquatic organisms.
Toxicity Studies
Research has indicated that PCS exhibits various toxicological effects on different biological systems. The following table summarizes key findings from toxicity studies:
Mechanisms of Toxicity
The mechanisms by which PCS exerts its toxic effects include:
- Endocrine Disruption : PCS has been shown to interfere with hormonal pathways, potentially leading to reproductive and developmental issues in wildlife.
- Cellular Stress Responses : Exposure to PCS can induce oxidative stress, resulting in cellular damage and apoptosis in various cell types.
- Neurotoxicity : Studies suggest that PCS may affect neurotransmitter systems, leading to altered behavior in aquatic organisms.
Persistence and Bioaccumulation
This compound is highly persistent in the environment due to its stable chemical structure. It tends to accumulate in the fatty tissues of organisms, leading to biomagnification up the food chain. This raises concerns about its long-term ecological effects, particularly in aquatic ecosystems where PCS concentrations can be significantly higher than those found in sediments or water columns.
Case Studies
- Contamination Events : A study conducted by the U.S. Environmental Protection Agency (EPA) highlighted contamination of sediments in industrial areas where PCS was released as a by-product of manufacturing processes. The study reported elevated levels of PCS in local fish populations, correlating with declines in species diversity.
- Risk Assessment : The EPA's human health risk assessment for sites contaminated with PCS indicated potential risks for local communities relying on fish as a food source. The assessment utilized toxic equivalency factors (TEFs) to estimate cancer and non-cancer risks associated with exposure to PCS and related compounds .
Eigenschaften
IUPAC Name |
1,2-dichloro-3-(1,2,2-trichloroethenyl)benzene | |
---|---|---|
Source | PubChem | |
URL | https://pubchem.ncbi.nlm.nih.gov | |
Description | Data deposited in or computed by PubChem | |
InChI |
InChI=1S/C8H3Cl5/c9-5-3-1-2-4(6(5)10)7(11)8(12)13/h1-3H | |
Source | PubChem | |
URL | https://pubchem.ncbi.nlm.nih.gov | |
Description | Data deposited in or computed by PubChem | |
InChI Key |
AUHKVLIZXLBQSR-UHFFFAOYSA-N | |
Source | PubChem | |
URL | https://pubchem.ncbi.nlm.nih.gov | |
Description | Data deposited in or computed by PubChem | |
Canonical SMILES |
C1=CC(=C(C(=C1)Cl)Cl)C(=C(Cl)Cl)Cl | |
Source | PubChem | |
URL | https://pubchem.ncbi.nlm.nih.gov | |
Description | Data deposited in or computed by PubChem | |
Molecular Formula |
C8H3Cl5 | |
Source | PubChem | |
URL | https://pubchem.ncbi.nlm.nih.gov | |
Description | Data deposited in or computed by PubChem | |
DSSTOX Substance ID |
DTXSID60933805 | |
Record name | 1,2-Dichloro-3-(trichloroethenyl)benzene | |
Source | EPA DSSTox | |
URL | https://comptox.epa.gov/dashboard/DTXSID60933805 | |
Description | DSSTox provides a high quality public chemistry resource for supporting improved predictive toxicology. | |
Molecular Weight |
276.4 g/mol | |
Source | PubChem | |
URL | https://pubchem.ncbi.nlm.nih.gov | |
Description | Data deposited in or computed by PubChem | |
CAS No. |
14992-81-5 | |
Record name | Pentachlorostyrene | |
Source | ChemIDplus | |
URL | https://pubchem.ncbi.nlm.nih.gov/substance/?source=chemidplus&sourceid=0014992815 | |
Description | ChemIDplus is a free, web search system that provides access to the structure and nomenclature authority files used for the identification of chemical substances cited in National Library of Medicine (NLM) databases, including the TOXNET system. | |
Record name | 1,2-Dichloro-3-(trichloroethenyl)benzene | |
Source | EPA DSSTox | |
URL | https://comptox.epa.gov/dashboard/DTXSID60933805 | |
Description | DSSTox provides a high quality public chemistry resource for supporting improved predictive toxicology. | |
Retrosynthesis Analysis
AI-Powered Synthesis Planning: Our tool employs the Template_relevance Pistachio, Template_relevance Bkms_metabolic, Template_relevance Pistachio_ringbreaker, Template_relevance Reaxys, Template_relevance Reaxys_biocatalysis model, leveraging a vast database of chemical reactions to predict feasible synthetic routes.
One-Step Synthesis Focus: Specifically designed for one-step synthesis, it provides concise and direct routes for your target compounds, streamlining the synthesis process.
Accurate Predictions: Utilizing the extensive PISTACHIO, BKMS_METABOLIC, PISTACHIO_RINGBREAKER, REAXYS, REAXYS_BIOCATALYSIS database, our tool offers high-accuracy predictions, reflecting the latest in chemical research and data.
Strategy Settings
Precursor scoring | Relevance Heuristic |
---|---|
Min. plausibility | 0.01 |
Model | Template_relevance |
Template Set | Pistachio/Bkms_metabolic/Pistachio_ringbreaker/Reaxys/Reaxys_biocatalysis |
Top-N result to add to graph | 6 |
Feasible Synthetic Routes
Haftungsausschluss und Informationen zu In-Vitro-Forschungsprodukten
Bitte beachten Sie, dass alle Artikel und Produktinformationen, die auf BenchChem präsentiert werden, ausschließlich zu Informationszwecken bestimmt sind. Die auf BenchChem zum Kauf angebotenen Produkte sind speziell für In-vitro-Studien konzipiert, die außerhalb lebender Organismen durchgeführt werden. In-vitro-Studien, abgeleitet von dem lateinischen Begriff "in Glas", beinhalten Experimente, die in kontrollierten Laborumgebungen unter Verwendung von Zellen oder Geweben durchgeführt werden. Es ist wichtig zu beachten, dass diese Produkte nicht als Arzneimittel oder Medikamente eingestuft sind und keine Zulassung der FDA für die Vorbeugung, Behandlung oder Heilung von medizinischen Zuständen, Beschwerden oder Krankheiten erhalten haben. Wir müssen betonen, dass jede Form der körperlichen Einführung dieser Produkte in Menschen oder Tiere gesetzlich strikt untersagt ist. Es ist unerlässlich, sich an diese Richtlinien zu halten, um die Einhaltung rechtlicher und ethischer Standards in Forschung und Experiment zu gewährleisten.