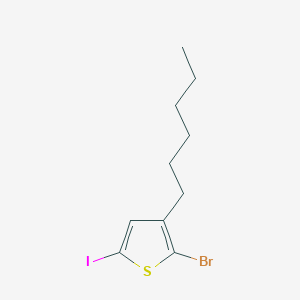
2-Bromo-3-hexyl-5-iodothiophene
Übersicht
Beschreibung
2-Bromo-3-hexyl-5-iodothiophene is an organohalide compound with the molecular formula C10H14BrIS. It is a derivative of thiophene, a five-membered aromatic ring containing sulfur. This compound is notable for its use in the synthesis of conjugated polymers, which are essential in the field of organic electronics.
Vorbereitungsmethoden
Synthetic Routes and Reaction Conditions
The synthesis of 2-Bromo-3-hexyl-5-iodothiophene typically involves halogenation reactions. One common method is the bromination of 3-hexylthiophene followed by iodination. The reaction conditions often include the use of solvents like tetrahydrofuran (THF) and catalysts such as nickel or palladium complexes .
Industrial Production Methods
In industrial settings, the production of this compound may involve large-scale halogenation processes. These processes are optimized for high yield and purity, often using continuous flow reactors and advanced purification techniques .
Analyse Chemischer Reaktionen
Types of Reactions
2-Bromo-3-hexyl-5-iodothiophene undergoes various chemical reactions, including:
Substitution Reactions: It can participate in nucleophilic substitution reactions where the bromine or iodine atoms are replaced by other nucleophiles.
Coupling Reactions: It is commonly used in cross-coupling reactions, such as Suzuki-Miyaura and Stille couplings, to form carbon-carbon bonds.
Common Reagents and Conditions
Nucleophilic Substitution: Reagents like sodium iodide in acetone can be used for substitution reactions.
Cross-Coupling: Palladium or nickel catalysts are often employed in the presence of ligands and bases such as triphenylphosphine and potassium carbonate.
Major Products
The major products formed from these reactions include various substituted thiophenes and conjugated polymers, which are valuable in electronic applications .
Wissenschaftliche Forschungsanwendungen
2-Bromo-3-hexyl-5-iodothiophene is extensively used in scientific research, particularly in the synthesis of conjugated polymers like poly(3-hexylthiophene). These polymers are crucial in the development of organic photovoltaic cells, organic field-effect transistors, and other electronic devices . Additionally, it is used in the study of polymerization mechanisms and the development of new materials with tailored electronic properties .
Wirkmechanismus
The mechanism by which 2-Bromo-3-hexyl-5-iodothiophene exerts its effects is primarily through its role as a building block in polymer synthesis. The bromine and iodine atoms facilitate cross-coupling reactions, allowing for the formation of extended conjugated systems. These systems exhibit unique electronic properties due to the delocalization of π-electrons along the polymer backbone .
Vergleich Mit ähnlichen Verbindungen
Similar Compounds
- 2,5-Dibromo-3-hexylthiophene
- 3-Hexylthiophene
- 2-Bromo-3-hexylthiophene
Uniqueness
Compared to similar compounds, 2-Bromo-3-hexyl-5-iodothiophene offers a unique combination of reactivity and stability, making it particularly useful in the synthesis of high-performance conjugated polymers. Its dual halogenation allows for versatile functionalization and polymerization strategies .
Eigenschaften
IUPAC Name |
2-bromo-3-hexyl-5-iodothiophene | |
---|---|---|
Source | PubChem | |
URL | https://pubchem.ncbi.nlm.nih.gov | |
Description | Data deposited in or computed by PubChem | |
InChI |
InChI=1S/C10H14BrIS/c1-2-3-4-5-6-8-7-9(12)13-10(8)11/h7H,2-6H2,1H3 | |
Source | PubChem | |
URL | https://pubchem.ncbi.nlm.nih.gov | |
Description | Data deposited in or computed by PubChem | |
InChI Key |
SDMKEQYHISDGKT-UHFFFAOYSA-N | |
Source | PubChem | |
URL | https://pubchem.ncbi.nlm.nih.gov | |
Description | Data deposited in or computed by PubChem | |
Canonical SMILES |
CCCCCCC1=C(SC(=C1)I)Br | |
Source | PubChem | |
URL | https://pubchem.ncbi.nlm.nih.gov | |
Description | Data deposited in or computed by PubChem | |
Molecular Formula |
C10H14BrIS | |
Source | PubChem | |
URL | https://pubchem.ncbi.nlm.nih.gov | |
Description | Data deposited in or computed by PubChem | |
Related CAS |
160096-82-2 | |
Record name | Thiophene, 2-bromo-3-hexyl-5-iodo-, homopolymer | |
Source | CAS Common Chemistry | |
URL | https://commonchemistry.cas.org/detail?cas_rn=160096-82-2 | |
Description | CAS Common Chemistry is an open community resource for accessing chemical information. Nearly 500,000 chemical substances from CAS REGISTRY cover areas of community interest, including common and frequently regulated chemicals, and those relevant to high school and undergraduate chemistry classes. This chemical information, curated by our expert scientists, is provided in alignment with our mission as a division of the American Chemical Society. | |
Explanation | The data from CAS Common Chemistry is provided under a CC-BY-NC 4.0 license, unless otherwise stated. | |
DSSTOX Substance ID |
DTXSID80467091 | |
Record name | 2-Bromo-5-iodo-3-hexylthiophene | |
Source | EPA DSSTox | |
URL | https://comptox.epa.gov/dashboard/DTXSID80467091 | |
Description | DSSTox provides a high quality public chemistry resource for supporting improved predictive toxicology. | |
Molecular Weight |
373.09 g/mol | |
Source | PubChem | |
URL | https://pubchem.ncbi.nlm.nih.gov | |
Description | Data deposited in or computed by PubChem | |
CAS No. |
160096-76-4 | |
Record name | 2-Bromo-5-iodo-3-hexylthiophene | |
Source | EPA DSSTox | |
URL | https://comptox.epa.gov/dashboard/DTXSID80467091 | |
Description | DSSTox provides a high quality public chemistry resource for supporting improved predictive toxicology. | |
Record name | 2-BROMO-3-HEXYL-5-IODOTHIOPHENE | |
Source | European Chemicals Agency (ECHA) | |
URL | https://echa.europa.eu/information-on-chemicals | |
Description | The European Chemicals Agency (ECHA) is an agency of the European Union which is the driving force among regulatory authorities in implementing the EU's groundbreaking chemicals legislation for the benefit of human health and the environment as well as for innovation and competitiveness. | |
Explanation | Use of the information, documents and data from the ECHA website is subject to the terms and conditions of this Legal Notice, and subject to other binding limitations provided for under applicable law, the information, documents and data made available on the ECHA website may be reproduced, distributed and/or used, totally or in part, for non-commercial purposes provided that ECHA is acknowledged as the source: "Source: European Chemicals Agency, http://echa.europa.eu/". Such acknowledgement must be included in each copy of the material. ECHA permits and encourages organisations and individuals to create links to the ECHA website under the following cumulative conditions: Links can only be made to webpages that provide a link to the Legal Notice page. | |
Retrosynthesis Analysis
AI-Powered Synthesis Planning: Our tool employs the Template_relevance Pistachio, Template_relevance Bkms_metabolic, Template_relevance Pistachio_ringbreaker, Template_relevance Reaxys, Template_relevance Reaxys_biocatalysis model, leveraging a vast database of chemical reactions to predict feasible synthetic routes.
One-Step Synthesis Focus: Specifically designed for one-step synthesis, it provides concise and direct routes for your target compounds, streamlining the synthesis process.
Accurate Predictions: Utilizing the extensive PISTACHIO, BKMS_METABOLIC, PISTACHIO_RINGBREAKER, REAXYS, REAXYS_BIOCATALYSIS database, our tool offers high-accuracy predictions, reflecting the latest in chemical research and data.
Strategy Settings
Precursor scoring | Relevance Heuristic |
---|---|
Min. plausibility | 0.01 |
Model | Template_relevance |
Template Set | Pistachio/Bkms_metabolic/Pistachio_ringbreaker/Reaxys/Reaxys_biocatalysis |
Top-N result to add to graph | 6 |
Feasible Synthetic Routes
Q1: What are the advantages of using 2-bromo-3-hexyl-5-iodothiophene over 2,5-dibromo-3-hexylthiophene in the synthesis of regioregular P3HT?
A: While both monomers can be used to synthesize P3HT, this compound offers higher regioselectivity during polymerization. This is due to the difference in reactivity between the bromine and iodine substituents. The iodine atom is preferentially activated by the nickel catalyst, leading to a more controlled and regioselective polymerization. [, ]
Q2: How does the addition of lithium chloride (LiCl) affect the polymerization of this compound?
A: Research shows that LiCl significantly enhances the polymerization rate of this compound when using a Ni(dppp)Cl2 catalyst. The propagation rate constant (kp) increases substantially, leading to faster polymerization. This effect is attributed to LiCl increasing the reactivity of the Grignard form of the monomer and potentially modifying the active nickel catalyst. [, ]
Q3: Can the molecular weight of P3HT be controlled during polymerization using this compound?
A: Yes, studies demonstrate that the molecular weight of P3HT can be controlled by adjusting the molar ratio of this compound to the Ni(dppp)Cl2 catalyst. This control over molecular weight is crucial for tuning the properties of the resulting P3HT polymer. []
Q4: Are there alternative synthetic routes to synthesize P3HT besides using this compound?
A: Yes, researchers have explored alternative methods for P3HT synthesis. One approach involves using 2,5-dibromo-3-hexylthiophene with a trialkylzincate complex and a nickel catalyst. This method, while potentially requiring less regio-selective halogenation steps, might necessitate optimization for large-scale production. []
Q5: What is the role of the nickel catalyst in the polymerization of this compound?
A: Nickel catalysts, particularly Ni(dppp)Cl2, are crucial for the controlled polymerization of this compound. The nickel catalyst facilitates the Kumada coupling reaction, enabling the formation of the carbon-carbon bonds that make up the P3HT backbone. [, , ]
Q6: What analytical techniques are used to characterize the synthesized P3HT?
A6: Various techniques are employed to analyze P3HT, including:
- Nuclear Magnetic Resonance (NMR) Spectroscopy: Provides structural information and can be used to assess the regioregularity of the polymer. []
- Gel Permeation Chromatography (GPC): Determines the molecular weight distribution of the synthesized P3HT. []
- UV-Vis Spectroscopy: Monitors the polymerization reaction in real-time and can provide information about the polymer's optical properties. [, ]
- Atomic Force Microscopy (AFM): Allows visualization of the morphology and phase separation behavior of P3HT thin films. []
Q7: What are the potential applications of regioregular P3HT?
A7: Regioregular P3HT, due to its unique electrical and optical properties, holds promise for various applications:
Haftungsausschluss und Informationen zu In-Vitro-Forschungsprodukten
Bitte beachten Sie, dass alle Artikel und Produktinformationen, die auf BenchChem präsentiert werden, ausschließlich zu Informationszwecken bestimmt sind. Die auf BenchChem zum Kauf angebotenen Produkte sind speziell für In-vitro-Studien konzipiert, die außerhalb lebender Organismen durchgeführt werden. In-vitro-Studien, abgeleitet von dem lateinischen Begriff "in Glas", beinhalten Experimente, die in kontrollierten Laborumgebungen unter Verwendung von Zellen oder Geweben durchgeführt werden. Es ist wichtig zu beachten, dass diese Produkte nicht als Arzneimittel oder Medikamente eingestuft sind und keine Zulassung der FDA für die Vorbeugung, Behandlung oder Heilung von medizinischen Zuständen, Beschwerden oder Krankheiten erhalten haben. Wir müssen betonen, dass jede Form der körperlichen Einführung dieser Produkte in Menschen oder Tiere gesetzlich strikt untersagt ist. Es ist unerlässlich, sich an diese Richtlinien zu halten, um die Einhaltung rechtlicher und ethischer Standards in Forschung und Experiment zu gewährleisten.