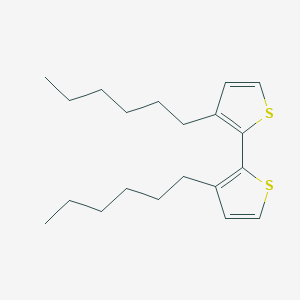
3,3'-Dihexyl-2,2'-Bithiophen
Übersicht
Beschreibung
3,3’-Dihexyl-2,2’-bithiophene: is an organic compound belonging to the class of bithiophenes. It is characterized by the presence of two thiophene rings connected by a single bond, with hexyl groups attached to the 3-position of each thiophene ring. This compound is known for its applications in organic electronics, particularly in the development of organic semiconductors and photovoltaic devices .
Wissenschaftliche Forschungsanwendungen
Chemistry: 3,3’-Dihexyl-2,2’-bithiophene is widely used in the synthesis of conjugated polymers and oligomers, which are essential components in organic electronic devices .
Biology and Medicine: While its primary applications are in materials science, research is ongoing to explore its potential in biological systems, particularly in the development of biosensors .
Industry: In the industrial sector, 3,3’-Dihexyl-2,2’-bithiophene is used in the fabrication of organic field-effect transistors (OFETs), organic photovoltaics (OPVs), and organic light-emitting diodes (OLEDs) .
Wirkmechanismus
Target of Action
3,3’-Dihexyl-2,2’-bithiophene is primarily used in the field of organic electronics . Its primary targets are the electronic devices where it is used as a monomer for synthesizing polymers .
Mode of Action
The compound interacts with its targets by contributing to the formation of regioregular-P3HT-regiosymmetric-P3HT, a type of diblock polymer . This interaction results in the creation of organic electronic devices with specific properties.
Biochemical Pathways
It is known that the compound plays a crucial role in the synthesis of polymers used in organic electronics .
Result of Action
The molecular and cellular effects of 3,3’-Dihexyl-2,2’-bithiophene’s action are best observed in the performance of the electronic devices it helps create. For instance, it contributes to the development of organic solar cells, thin film transistors, chemical sensors, and photovoltaic cells .
Action Environment
Environmental factors such as temperature, humidity, and light exposure can influence the action, efficacy, and stability of 3,3’-Dihexyl-2,2’-bithiophene. For instance, it should be stored under inert gas at a temperature between 0-10°C to maintain its stability .
Vorbereitungsmethoden
Synthetic Routes and Reaction Conditions: The synthesis of 3,3’-Dihexyl-2,2’-bithiophene typically involves the following steps:
Bromination: The starting material, 3-hexylthiophene, is brominated using bromine or N-bromosuccinimide to yield 2,5-dibromo-3-hexylthiophene.
Coupling Reaction: The dibromo compound undergoes a coupling reaction, such as the Stille coupling, with a suitable organotin reagent to form 3,3’-Dihexyl-2,2’-bithiophene.
Industrial Production Methods: Industrial production of 3,3’-Dihexyl-2,2’-bithiophene follows similar synthetic routes but on a larger scale. The reaction conditions are optimized to ensure high yield and purity. The compound is typically purified using column chromatography or recrystallization techniques .
Analyse Chemischer Reaktionen
Types of Reactions:
Oxidation: 3,3’-Dihexyl-2,2’-bithiophene can undergo oxidation reactions, often using reagents like hydrogen peroxide or m-chloroperbenzoic acid, leading to the formation of sulfoxides or sulfones.
Reduction: Reduction reactions can be carried out using reducing agents such as lithium aluminum hydride, resulting in the formation of dihydro derivatives.
Common Reagents and Conditions:
Oxidation: Hydrogen peroxide, m-chloroperbenzoic acid.
Reduction: Lithium aluminum hydride.
Substitution: Electrophiles like bromine, iodine, or nitronium ions.
Major Products Formed:
Oxidation: Sulfoxides, sulfones.
Reduction: Dihydro derivatives.
Substitution: Halogenated or nitrated derivatives.
Vergleich Mit ähnlichen Verbindungen
3,3’-Dihexyl-2,2’-quaterthiophene: This compound has an additional thiophene ring, providing extended conjugation and potentially higher charge mobility.
2,2’-Bithiophene: Lacks the hexyl groups, resulting in lower solubility and processability.
Poly(3-hexylthiophene): A polymeric form with similar electronic properties but different mechanical and processing characteristics.
Uniqueness: 3,3’-Dihexyl-2,2’-bithiophene stands out due to its balanced properties of solubility, processability, and electronic performance. The presence of hexyl groups enhances its applicability in solution-based processing techniques, making it a versatile material for various organic electronic applications .
Eigenschaften
IUPAC Name |
3-hexyl-2-(3-hexylthiophen-2-yl)thiophene | |
---|---|---|
Source | PubChem | |
URL | https://pubchem.ncbi.nlm.nih.gov | |
Description | Data deposited in or computed by PubChem | |
InChI |
InChI=1S/C20H30S2/c1-3-5-7-9-11-17-13-15-21-19(17)20-18(14-16-22-20)12-10-8-6-4-2/h13-16H,3-12H2,1-2H3 | |
Source | PubChem | |
URL | https://pubchem.ncbi.nlm.nih.gov | |
Description | Data deposited in or computed by PubChem | |
InChI Key |
RQHMOXZEVWCNMG-UHFFFAOYSA-N | |
Source | PubChem | |
URL | https://pubchem.ncbi.nlm.nih.gov | |
Description | Data deposited in or computed by PubChem | |
Canonical SMILES |
CCCCCCC1=C(SC=C1)C2=C(C=CS2)CCCCCC | |
Source | PubChem | |
URL | https://pubchem.ncbi.nlm.nih.gov | |
Description | Data deposited in or computed by PubChem | |
Molecular Formula |
C20H30S2 | |
Source | PubChem | |
URL | https://pubchem.ncbi.nlm.nih.gov | |
Description | Data deposited in or computed by PubChem | |
DSSTOX Substance ID |
DTXSID10467423 | |
Record name | 3,3'-Dihexyl-2,2'-bithiophene | |
Source | EPA DSSTox | |
URL | https://comptox.epa.gov/dashboard/DTXSID10467423 | |
Description | DSSTox provides a high quality public chemistry resource for supporting improved predictive toxicology. | |
Molecular Weight |
334.6 g/mol | |
Source | PubChem | |
URL | https://pubchem.ncbi.nlm.nih.gov | |
Description | Data deposited in or computed by PubChem | |
CAS No. |
125607-30-9 | |
Record name | 3,3'-Dihexyl-2,2'-bithiophene | |
Source | EPA DSSTox | |
URL | https://comptox.epa.gov/dashboard/DTXSID10467423 | |
Description | DSSTox provides a high quality public chemistry resource for supporting improved predictive toxicology. | |
Synthesis routes and methods I
Procedure details
Synthesis routes and methods II
Procedure details
Retrosynthesis Analysis
AI-Powered Synthesis Planning: Our tool employs the Template_relevance Pistachio, Template_relevance Bkms_metabolic, Template_relevance Pistachio_ringbreaker, Template_relevance Reaxys, Template_relevance Reaxys_biocatalysis model, leveraging a vast database of chemical reactions to predict feasible synthetic routes.
One-Step Synthesis Focus: Specifically designed for one-step synthesis, it provides concise and direct routes for your target compounds, streamlining the synthesis process.
Accurate Predictions: Utilizing the extensive PISTACHIO, BKMS_METABOLIC, PISTACHIO_RINGBREAKER, REAXYS, REAXYS_BIOCATALYSIS database, our tool offers high-accuracy predictions, reflecting the latest in chemical research and data.
Strategy Settings
Precursor scoring | Relevance Heuristic |
---|---|
Min. plausibility | 0.01 |
Model | Template_relevance |
Template Set | Pistachio/Bkms_metabolic/Pistachio_ringbreaker/Reaxys/Reaxys_biocatalysis |
Top-N result to add to graph | 6 |
Feasible Synthetic Routes
Q1: What is the structural significance of 3,3'-Dihexyl-2,2'-bithiophene in polymer synthesis?
A1: 3,3'-Dihexyl-2,2'-bithiophene serves as a crucial building block in synthesizing donor-acceptor (D-A) polymers. [] These polymers often exhibit interesting optoelectronic properties and find applications in organic electronics. The dihexyl side chains attached to the bithiophene core influence the solubility and self-assembly of the resulting polymers. []
Q2: How does the structure of 3,3'-Dihexyl-2,2'-bithiophene affect its fluorescence properties?
A2: Research indicates that incorporating 3,3'-Dihexyl-2,2'-bithiophene into a polymer with 1,2,4,5-tetrafluorophenylene results in a material capable of red-colored fluorescence. [] Interestingly, the choice of solvent significantly impacts the polymer's self-assembly, leading to different macrostructures (spherical vs. fibrous) and fluorescence colors (red vs. yellow). [] This highlights the influence of structural organization on the optical properties of materials containing 3,3'-Dihexyl-2,2'-bithiophene.
Q3: Can you elaborate on the role of 3,3'-Dihexyl-2,2'-bithiophene in metal-organic frameworks (MOFs)?
A3: 3,3'-Dihexyl-2,2'-bithiophene acts as a linker in the synthesis of manganese-based MOFs. [] This specific linker influences the dimensionality of the resulting MOF structure, demonstrating its importance in designing materials with tailored properties. [] Moreover, research using 3,3'-Dihexyl-2,2'-bithiophene and other functionalized bithiophene dicarboxylic acids as linkers in zinc-based MOFs has shed light on how structural variations impact the MOFs' luminescent properties. []
Q4: How does 3,3'-Dihexyl-2,2'-bithiophene behave in palladium-catalyzed reactions?
A4: Studies have shown that during a palladium/silver co-catalyzed cross-dehydrogenative coupling polymerization utilizing 3,3'-Dihexyl-2,2'-bithiophene, an interesting phenomenon occurs. [] The second chain extension cross-coupling, involving the bithiophene unit, proceeds significantly faster than the initial cross-coupling and homocoupling reactions. [] This accelerated chain extension, attributed to strong palladium-thiophene interactions, results in polymers with high molecular weight and minimal homocoupling defects. []
Q5: Is there evidence of 3,3'-Dihexyl-2,2'-bithiophene contributing to thermochromic behavior in polymers?
A5: While 3,3'-Dihexyl-2,2'-bithiophene itself doesn't exhibit thermochromism, research shows that polymers incorporating similar structures, like poly(3-dodecylthiophene), display temperature-dependent color changes. [] This effect is attributed to conformational transitions within the polymer chain driven by temperature changes. [] While poly(3,3′-dihexyl-2,2′-bithiophene) might not adopt a co-planar conformation due to strong steric interactions, this highlights the potential impact of structural modifications on the thermochromic properties of related polymers. []
Haftungsausschluss und Informationen zu In-Vitro-Forschungsprodukten
Bitte beachten Sie, dass alle Artikel und Produktinformationen, die auf BenchChem präsentiert werden, ausschließlich zu Informationszwecken bestimmt sind. Die auf BenchChem zum Kauf angebotenen Produkte sind speziell für In-vitro-Studien konzipiert, die außerhalb lebender Organismen durchgeführt werden. In-vitro-Studien, abgeleitet von dem lateinischen Begriff "in Glas", beinhalten Experimente, die in kontrollierten Laborumgebungen unter Verwendung von Zellen oder Geweben durchgeführt werden. Es ist wichtig zu beachten, dass diese Produkte nicht als Arzneimittel oder Medikamente eingestuft sind und keine Zulassung der FDA für die Vorbeugung, Behandlung oder Heilung von medizinischen Zuständen, Beschwerden oder Krankheiten erhalten haben. Wir müssen betonen, dass jede Form der körperlichen Einführung dieser Produkte in Menschen oder Tiere gesetzlich strikt untersagt ist. Es ist unerlässlich, sich an diese Richtlinien zu halten, um die Einhaltung rechtlicher und ethischer Standards in Forschung und Experiment zu gewährleisten.