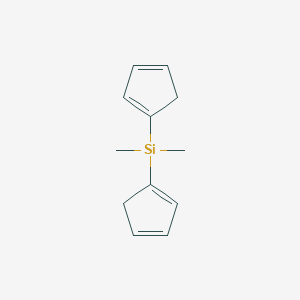
DI(Cyclopentadienyl)dimethylsilane
Übersicht
Beschreibung
DI(Cyclopentadienyl)dimethylsilane is an organosilicon compound with the molecular formula C12H16Si. It is characterized by the presence of two cyclopentadienyl rings attached to a silicon atom, which is also bonded to two methyl groups. This compound is of significant interest in organometallic chemistry due to its unique structure and reactivity.
Vorbereitungsmethoden
Synthetic Routes and Reaction Conditions
The synthesis of DI(Cyclopentadienyl)dimethylsilane typically involves the reaction of cyclopentadienyl sodium with dimethyldichlorosilane. The reaction is carried out in an inert atmosphere, often using solvents like tetrahydrofuran (THF) to facilitate the reaction. The general reaction scheme is as follows:
2C5H5Na+SiCl2(CH3)2→(C5H5)2Si(CH3)2+2NaCl
Industrial Production Methods
Industrial production methods for this compound are similar to laboratory synthesis but are scaled up to accommodate larger quantities. The reaction conditions are optimized for higher yields and purity, often involving continuous flow reactors and advanced purification techniques.
Analyse Chemischer Reaktionen
Types of Reactions
DI(Cyclopentadienyl)dimethylsilane undergoes various types of chemical reactions, including:
Oxidation: The compound can be oxidized to form silanols or siloxanes.
Reduction: Reduction reactions can lead to the formation of silyl anions.
Substitution: The methyl groups or cyclopentadienyl rings can be substituted with other functional groups.
Common Reagents and Conditions
Oxidation: Common oxidizing agents include hydrogen peroxide (H2O2) and potassium permanganate (KMnO4).
Reduction: Reducing agents such as lithium aluminum hydride (LiAlH4) are often used.
Substitution: Substitution reactions may involve reagents like Grignard reagents or organolithium compounds.
Major Products
The major products formed from these reactions depend on the specific conditions and reagents used. For example, oxidation can yield silanols, while substitution reactions can produce a variety of organosilicon compounds.
Wissenschaftliche Forschungsanwendungen
DI(Cyclopentadienyl)dimethylsilane has a wide range of applications in scientific research:
Chemistry: It is used as a precursor for the synthesis of various organosilicon compounds and as a ligand in transition metal complexes.
Biology: Research is ongoing into its potential use in drug delivery systems due to its ability to form stable complexes with metals.
Medicine: Its derivatives are being explored for their potential use in medical imaging and as therapeutic agents.
Industry: It is used in the production of high-performance materials, including polymers and coatings.
Wirkmechanismus
The mechanism by which DI(Cyclopentadienyl)dimethylsilane exerts its effects is primarily through its ability to form stable complexes with transition metals. The cyclopentadienyl rings act as ligands, coordinating with metal centers to form metallocenes. These complexes are highly stable and exhibit unique reactivity, making them valuable in catalysis and other applications.
Vergleich Mit ähnlichen Verbindungen
Similar Compounds
Ferrocene: (C5H5)2Fe
Ruthenocene: (C5H5)2Ru
Zirconocene: (C5H5)2ZrCl2
Uniqueness
DI(Cyclopentadienyl)dimethylsilane is unique due to the presence of silicon, which imparts different chemical properties compared to its carbon-based analogs. The silicon atom allows for greater flexibility in the types of reactions the compound can undergo, making it a versatile building block in organometallic chemistry.
Eigenschaften
IUPAC Name |
di(cyclopenta-1,3-dien-1-yl)-dimethylsilane | |
---|---|---|
Source | PubChem | |
URL | https://pubchem.ncbi.nlm.nih.gov | |
Description | Data deposited in or computed by PubChem | |
InChI |
InChI=1S/C12H16Si/c1-13(2,11-7-3-4-8-11)12-9-5-6-10-12/h3-7,9H,8,10H2,1-2H3 | |
Source | PubChem | |
URL | https://pubchem.ncbi.nlm.nih.gov | |
Description | Data deposited in or computed by PubChem | |
InChI Key |
FPWYHHNBOYUKSS-UHFFFAOYSA-N | |
Source | PubChem | |
URL | https://pubchem.ncbi.nlm.nih.gov | |
Description | Data deposited in or computed by PubChem | |
Canonical SMILES |
C[Si](C)(C1=CC=CC1)C2=CC=CC2 | |
Source | PubChem | |
URL | https://pubchem.ncbi.nlm.nih.gov | |
Description | Data deposited in or computed by PubChem | |
Molecular Formula |
C12H16Si | |
Source | PubChem | |
URL | https://pubchem.ncbi.nlm.nih.gov | |
Description | Data deposited in or computed by PubChem | |
DSSTOX Substance ID |
DTXSID30478233 | |
Record name | Silane, dicyclopentadienyldimethyl- | |
Source | EPA DSSTox | |
URL | https://comptox.epa.gov/dashboard/DTXSID30478233 | |
Description | DSSTox provides a high quality public chemistry resource for supporting improved predictive toxicology. | |
Molecular Weight |
188.34 g/mol | |
Source | PubChem | |
URL | https://pubchem.ncbi.nlm.nih.gov | |
Description | Data deposited in or computed by PubChem | |
CAS No. |
107241-50-9 | |
Record name | Silane, dicyclopentadienyldimethyl- | |
Source | EPA DSSTox | |
URL | https://comptox.epa.gov/dashboard/DTXSID30478233 | |
Description | DSSTox provides a high quality public chemistry resource for supporting improved predictive toxicology. | |
Retrosynthesis Analysis
AI-Powered Synthesis Planning: Our tool employs the Template_relevance Pistachio, Template_relevance Bkms_metabolic, Template_relevance Pistachio_ringbreaker, Template_relevance Reaxys, Template_relevance Reaxys_biocatalysis model, leveraging a vast database of chemical reactions to predict feasible synthetic routes.
One-Step Synthesis Focus: Specifically designed for one-step synthesis, it provides concise and direct routes for your target compounds, streamlining the synthesis process.
Accurate Predictions: Utilizing the extensive PISTACHIO, BKMS_METABOLIC, PISTACHIO_RINGBREAKER, REAXYS, REAXYS_BIOCATALYSIS database, our tool offers high-accuracy predictions, reflecting the latest in chemical research and data.
Strategy Settings
Precursor scoring | Relevance Heuristic |
---|---|
Min. plausibility | 0.01 |
Model | Template_relevance |
Template Set | Pistachio/Bkms_metabolic/Pistachio_ringbreaker/Reaxys/Reaxys_biocatalysis |
Top-N result to add to graph | 6 |
Feasible Synthetic Routes
Q1: What is the role of di(cyclopentadienyl)dimethylsilane in synthesizing ruthenocenophanes?
A1: this compound serves as a crucial precursor in the synthesis of [1.1]ruthenocenophanes, specifically bis(dimethylsila)-[1.1]ruthenocenophane []. The synthesis involves reacting dilithium this compound with RuCl2·4 dmso in a tetrahydrofuran solution []. This reaction highlights the compound's ability to act as a ligand source, contributing both cyclopentadienyl rings and the bridging dimethylsilyl group to the final ruthenocenophane structure.
Haftungsausschluss und Informationen zu In-Vitro-Forschungsprodukten
Bitte beachten Sie, dass alle Artikel und Produktinformationen, die auf BenchChem präsentiert werden, ausschließlich zu Informationszwecken bestimmt sind. Die auf BenchChem zum Kauf angebotenen Produkte sind speziell für In-vitro-Studien konzipiert, die außerhalb lebender Organismen durchgeführt werden. In-vitro-Studien, abgeleitet von dem lateinischen Begriff "in Glas", beinhalten Experimente, die in kontrollierten Laborumgebungen unter Verwendung von Zellen oder Geweben durchgeführt werden. Es ist wichtig zu beachten, dass diese Produkte nicht als Arzneimittel oder Medikamente eingestuft sind und keine Zulassung der FDA für die Vorbeugung, Behandlung oder Heilung von medizinischen Zuständen, Beschwerden oder Krankheiten erhalten haben. Wir müssen betonen, dass jede Form der körperlichen Einführung dieser Produkte in Menschen oder Tiere gesetzlich strikt untersagt ist. Es ist unerlässlich, sich an diese Richtlinien zu halten, um die Einhaltung rechtlicher und ethischer Standards in Forschung und Experiment zu gewährleisten.