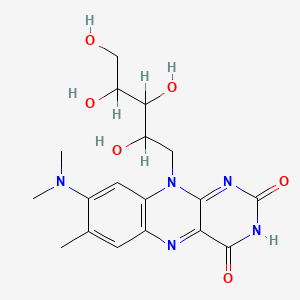
Roseoflavin
Übersicht
Beschreibung
Roseoflavin is a naturally occurring riboflavin (vitamin B2) analogue with significant antibiotic properties. It is produced by the bacteria Streptomyces davaonensis and Streptomyces cinnabarinus. This compound is unique as it is the only known natural riboflavin analogue with antibiotic activity, making it a promising candidate for various scientific and medical applications .
Wissenschaftliche Forschungsanwendungen
Roseoflavin has a wide range of scientific research applications:
Chemistry: this compound is used as a model compound to study the properties and reactions of riboflavin analogues.
Biology: It serves as a tool to investigate the metabolic pathways involving riboflavin and its analogues.
Medicine: this compound has shown potential as an antimicrobial agent against various bacteria, including Staphylococcus aureus, Enterococcus faecalis, and Streptococcus pyogenes.
Wirkmechanismus
Target of Action
Roseoflavin, a natural riboflavin (vitamin B2) analogue, primarily targets the metabolism of riboflavin and the utilization of riboflavin metabolites . The key enzymes responsible for this compound biosynthesis have been identified . These include the enzyme encoded by the gene rosB
, which converts flavin mononucleotide (FMN) to 8-demethyl-8-aminoriboflavin-5’-phosphate .
Mode of Action
This compound interacts with its targets by inhibiting the production of flavin mononucleotide (FMN) and flavin adenine dinucleotide (FAD), essential cofactors in various biochemical reactions . It binds to FMN riboswitches, thereby regulating gene expression .
Biochemical Pathways
The biochemical pathway of this compound biosynthesis starts from flavin mononucleotide (FMN) and proceeds through an immediate precursor, aminoriboflavin . The enzyme encoded by the gene rosB
converts FMN to 8-demethyl-8-aminoriboflavin-5’-phosphate (aminoriboflavin-5’-phosphate, AFP). AFP is then dephosphorylated to aminoriboflavin (AF) by a specific phosphatase, the product of the gene rosC
. Finally, AF is dimethylated to this compound using an enzyme encoded by rosA
.
Pharmacokinetics
It is known that this compound is naturally produced by certain bacteria
Result of Action
The molecular and cellular effects of this compound’s action include the inhibition of growth in various bacteria and protozoal parasites . For instance, this compound has been found to inhibit the growth of Staphylococcus aureus, Enterococcus faecalis, Streptococcus pyogenes, Listeria monocytogenes, and parasites like Trypanosoma cruzi, Trypanosoma brucei, and Leishmania mexicana .
Action Environment
It is known that this compound is used in the food industry to select this compound-resistant strains , suggesting that its action can be influenced by the presence of other microbial species
Biochemische Analyse
Biochemical Properties
Roseoflavin plays a significant role in biochemical reactions due to its antibiotic properties. It interacts with various enzymes, proteins, and other biomolecules. The biosynthesis of this compound involves several key enzymes, including the enzyme encoded by the gene rosB, which converts flavin mononucleotide to 8-demethyl-8-aminoriboflavin-5’-phosphate . This intermediate is then dephosphorylated to aminoriboflavin by a specific phosphatase encoded by the gene rosC . Finally, aminoriboflavin is dimethylated to this compound using an enzyme encoded by the gene rosA . These interactions highlight the complex biochemical pathways involved in the production of this compound.
Cellular Effects
This compound exerts various effects on different types of cells and cellular processes. It has been shown to inhibit the growth of several bacterial strains, including Staphylococcus aureus and Listeria monocytogenes . This compound influences cell function by interfering with cell signaling pathways, gene expression, and cellular metabolism. For instance, it can disrupt the normal functioning of riboflavin-dependent enzymes, leading to altered metabolic processes and impaired bacterial growth .
Molecular Mechanism
The molecular mechanism of action of this compound involves its interaction with riboflavin-dependent enzymes. This compound acts as an antimetabolite by mimicking riboflavin and competitively inhibiting riboflavin-dependent enzymes . This inhibition disrupts the normal catalytic activities of these enzymes, leading to a cascade of effects on cellular metabolism and gene expression. Additionally, this compound can bind to riboflavin transporters, further inhibiting the uptake of riboflavin by bacterial cells .
Temporal Effects in Laboratory Settings
In laboratory settings, the effects of this compound have been observed to change over time. This compound is relatively stable under standard laboratory conditions, but it can degrade over extended periods . Long-term studies have shown that this compound can have sustained inhibitory effects on bacterial growth, although the extent of inhibition may decrease over time due to degradation . These temporal effects highlight the importance of considering the stability and degradation of this compound in experimental designs.
Dosage Effects in Animal Models
The effects of this compound vary with different dosages in animal models. At lower doses, this compound has been shown to effectively inhibit bacterial growth without causing significant toxic effects . At higher doses, this compound can exhibit toxic or adverse effects, including potential damage to host tissues . These dosage-dependent effects underscore the need for careful optimization of this compound dosages in therapeutic applications.
Metabolic Pathways
This compound is involved in several metabolic pathways, primarily related to its role as an antimetabolite. It interacts with enzymes involved in riboflavin metabolism, such as riboflavin kinase and flavokinase . By inhibiting these enzymes, this compound disrupts the normal metabolic flux of riboflavin and its derivatives, leading to altered levels of metabolites and impaired cellular functions . These interactions highlight the intricate metabolic pathways influenced by this compound.
Transport and Distribution
Within cells and tissues, this compound is transported and distributed through specific transporters and binding proteins. It can interact with riboflavin transporters, affecting its localization and accumulation within cells . The distribution of this compound is influenced by its binding affinity to these transporters, which can impact its overall efficacy and bioavailability . Understanding the transport and distribution mechanisms of this compound is crucial for optimizing its therapeutic potential.
Subcellular Localization
This compound exhibits specific subcellular localization patterns that influence its activity and function. It can be targeted to specific compartments or organelles through post-translational modifications and targeting signals . These localization patterns are essential for understanding the precise mechanisms by which this compound exerts its effects within cells. By studying the subcellular localization of this compound, researchers can gain insights into its mode of action and potential therapeutic applications.
Vorbereitungsmethoden
Synthetic Routes and Reaction Conditions: Roseoflavin is primarily produced through chemical synthesis. The synthesis begins with the nitration of N,N-dimethyl-o-toluidine to form 2-dimethylamino-4-nitrotoluene. This compound is then reduced using hydrazine in the presence of Raney nickel to yield 2-dimethylamino-4-aminotoluene. The next step involves the condensation of this compound with D-ribose, followed by pressure hydrogenation in the presence of Raney nickel to produce 2-dimethylamino-4-o-ribitylaminotoluene. This intermediate is then condensed with violuric acid to form this compound. The crude product is acetylated to form this compound-tetraacetate, which is then recrystallized from methanol. Finally, the purified acetate is hydrolyzed with sodium hydroxide to yield this compound .
Industrial Production Methods: Industrial production of this compound involves the use of genetically engineered microorganisms. For instance, the genes responsible for this compound biosynthesis have been successfully expressed in Corynebacterium glutamicum and Streptomyces davaonensis, leading to enhanced production yields. These methods provide a more economical and scalable approach to producing this compound .
Analyse Chemischer Reaktionen
Types of Reactions: Roseoflavin undergoes various chemical reactions, including oxidation, reduction, and substitution reactions.
Common Reagents and Conditions:
Oxidation: this compound can be oxidized using common oxidizing agents such as hydrogen peroxide or potassium permanganate.
Reduction: Reduction of this compound can be achieved using reducing agents like sodium borohydride or lithium aluminum hydride.
Substitution: Substitution reactions involving this compound typically require nucleophilic reagents under basic conditions.
Major Products: The major products formed from these reactions depend on the specific reagents and conditions used. For example, oxidation of this compound can yield various oxidized derivatives, while reduction can produce reduced forms of the compound .
Vergleich Mit ähnlichen Verbindungen
Riboflavin: The parent compound of roseoflavin, riboflavin, is a vital nutrient involved in various cellular processes.
8-Aminoriboflavin: Another riboflavin analogue with similar properties to this compound, but with different biological activities.
Uniqueness of this compound: this compound is unique due to its antibiotic properties, which are not observed in other riboflavin analogues. Its ability to bind to flavin mononucleotide riboswitches and inhibit essential bacterial processes makes it a valuable compound for antimicrobial research and potential therapeutic applications .
Eigenschaften
IUPAC Name |
8-(dimethylamino)-7-methyl-10-(2,3,4,5-tetrahydroxypentyl)benzo[g]pteridine-2,4-dione | |
---|---|---|
Source | PubChem | |
URL | https://pubchem.ncbi.nlm.nih.gov | |
Description | Data deposited in or computed by PubChem | |
InChI |
InChI=1S/C18H23N5O6/c1-8-4-9-11(5-10(8)22(2)3)23(6-12(25)15(27)13(26)7-24)16-14(19-9)17(28)21-18(29)20-16/h4-5,12-13,15,24-27H,6-7H2,1-3H3,(H,21,28,29) | |
Source | PubChem | |
URL | https://pubchem.ncbi.nlm.nih.gov | |
Description | Data deposited in or computed by PubChem | |
InChI Key |
IGQLDUYTWDABFK-UHFFFAOYSA-N | |
Source | PubChem | |
URL | https://pubchem.ncbi.nlm.nih.gov | |
Description | Data deposited in or computed by PubChem | |
Canonical SMILES |
CC1=CC2=C(C=C1N(C)C)N(C3=NC(=O)NC(=O)C3=N2)CC(C(C(CO)O)O)O | |
Source | PubChem | |
URL | https://pubchem.ncbi.nlm.nih.gov | |
Description | Data deposited in or computed by PubChem | |
Molecular Formula |
C18H23N5O6 | |
Source | PubChem | |
URL | https://pubchem.ncbi.nlm.nih.gov | |
Description | Data deposited in or computed by PubChem | |
DSSTOX Substance ID |
DTXSID60965368 | |
Record name | 1-Deoxy-1-[8-(dimethylamino)-4-hydroxy-7-methyl-2-oxobenzo[g]pteridin-10(2H)-yl]pentitol | |
Source | EPA DSSTox | |
URL | https://comptox.epa.gov/dashboard/DTXSID60965368 | |
Description | DSSTox provides a high quality public chemistry resource for supporting improved predictive toxicology. | |
Molecular Weight |
405.4 g/mol | |
Source | PubChem | |
URL | https://pubchem.ncbi.nlm.nih.gov | |
Description | Data deposited in or computed by PubChem | |
CAS No. |
51093-55-1 | |
Record name | Roseoflavin | |
Source | ChemIDplus | |
URL | https://pubchem.ncbi.nlm.nih.gov/substance/?source=chemidplus&sourceid=0051093551 | |
Description | ChemIDplus is a free, web search system that provides access to the structure and nomenclature authority files used for the identification of chemical substances cited in National Library of Medicine (NLM) databases, including the TOXNET system. | |
Record name | 1-Deoxy-1-[8-(dimethylamino)-4-hydroxy-7-methyl-2-oxobenzo[g]pteridin-10(2H)-yl]pentitol | |
Source | EPA DSSTox | |
URL | https://comptox.epa.gov/dashboard/DTXSID60965368 | |
Description | DSSTox provides a high quality public chemistry resource for supporting improved predictive toxicology. | |
Retrosynthesis Analysis
AI-Powered Synthesis Planning: Our tool employs the Template_relevance Pistachio, Template_relevance Bkms_metabolic, Template_relevance Pistachio_ringbreaker, Template_relevance Reaxys, Template_relevance Reaxys_biocatalysis model, leveraging a vast database of chemical reactions to predict feasible synthetic routes.
One-Step Synthesis Focus: Specifically designed for one-step synthesis, it provides concise and direct routes for your target compounds, streamlining the synthesis process.
Accurate Predictions: Utilizing the extensive PISTACHIO, BKMS_METABOLIC, PISTACHIO_RINGBREAKER, REAXYS, REAXYS_BIOCATALYSIS database, our tool offers high-accuracy predictions, reflecting the latest in chemical research and data.
Strategy Settings
Precursor scoring | Relevance Heuristic |
---|---|
Min. plausibility | 0.01 |
Model | Template_relevance |
Template Set | Pistachio/Bkms_metabolic/Pistachio_ringbreaker/Reaxys/Reaxys_biocatalysis |
Top-N result to add to graph | 6 |
Feasible Synthetic Routes
Haftungsausschluss und Informationen zu In-Vitro-Forschungsprodukten
Bitte beachten Sie, dass alle Artikel und Produktinformationen, die auf BenchChem präsentiert werden, ausschließlich zu Informationszwecken bestimmt sind. Die auf BenchChem zum Kauf angebotenen Produkte sind speziell für In-vitro-Studien konzipiert, die außerhalb lebender Organismen durchgeführt werden. In-vitro-Studien, abgeleitet von dem lateinischen Begriff "in Glas", beinhalten Experimente, die in kontrollierten Laborumgebungen unter Verwendung von Zellen oder Geweben durchgeführt werden. Es ist wichtig zu beachten, dass diese Produkte nicht als Arzneimittel oder Medikamente eingestuft sind und keine Zulassung der FDA für die Vorbeugung, Behandlung oder Heilung von medizinischen Zuständen, Beschwerden oder Krankheiten erhalten haben. Wir müssen betonen, dass jede Form der körperlichen Einführung dieser Produkte in Menschen oder Tiere gesetzlich strikt untersagt ist. Es ist unerlässlich, sich an diese Richtlinien zu halten, um die Einhaltung rechtlicher und ethischer Standards in Forschung und Experiment zu gewährleisten.