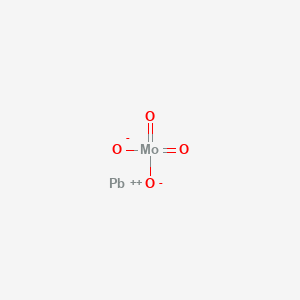
Blei-Molybdat
Übersicht
Beschreibung
Lead molybdate, with the chemical formula PbMoO₄, is a compound that occurs naturally as the mineral wulfenite. It is known for its vibrant colors, ranging from yellow to orange-red, and its tetragonal crystal structure. Lead molybdate is a significant material in various scientific and industrial applications due to its unique optical and electronic properties .
Wissenschaftliche Forschungsanwendungen
Lead molybdate has a wide range of applications in scientific research, including:
Photocatalysis: Lead molybdate is an effective photocatalyst for the degradation of organic pollutants and the reduction of heavy metals in water treatment processes.
Material Science: It is used in the synthesis of advanced materials with tailored properties for specific applications, such as sensors and catalysts.
Environmental Chemistry: Lead molybdate’s photocatalytic properties make it valuable in environmental remediation, particularly in the reduction of toxic heavy metals.
Wirkmechanismus
Target of Action
Lead molybdate (PbMoO4) is a wide band gap semiconductor . It primarily targets photoelectrochemical properties and is utilized in the construction of simple logic devices such as logic gates or switches, and more complex optoelectronic circuits . It also targets the Ce4+/Ce3+ redox couple, exhibiting the photoelectrochemical photocurrent switching effect (the PEPS effect) in their presence .
Mode of Action
Lead molybdate interacts with its targets through photoelectrochemical processes. In the presence of the Ce4+/Ce3+ redox couple, it exhibits the PEPS effect . This can be explained in terms of oxidation/reduction processes of cerium (III)/cerium (IV) species . The dye alizarin, when adsorbed onto PbMoO4, binds to Mo VI centres which are exposed on the surface .
Biochemical Pathways
Molybdenum, a component of lead molybdate, is incorporated into the molybdenum cofactor (Moco) in cells, functioning as the active site of several molybdenum-requiring enzymes . These enzymes participate in crucial biological processes such as nitrate assimilation, phytohormone biosynthesis, purine metabolism, and sulfite detoxification .
Pharmacokinetics
It is known that the composition of the reaction mixture, ie, the ratio between lead and molybdate ions, influences the morphology and the electronic structure of the semiconductor .
Result of Action
The interaction of lead molybdate with its targets results in changes in photoelectrochemical properties. Alizarin adsorbed onto PbMoO4 strongly enhances the cathodic photocurrent generation . The anodic photocurrent diminishes . This intense cathodic photocurrent generation may be utilized in photocatalysis, e.g., during the photoreduction of metal ions or the generation of reactive oxygen species and/or solar fuels .
Action Environment
The action of lead molybdate is influenced by environmental factors. Furthermore, the use of a lead complex in the synthesis of lead molybdate can lead to the creation of very tiny structures, which can be attributed to its steric effect .
Biochemische Analyse
Biochemical Properties
Lead molybdate plays a role in various biochemical reactions. It has been found to have high photocatalytic activity, which can be attributed to its nanostructure . This property allows it to interact with various enzymes, proteins, and other biomolecules, influencing their function and behavior .
Cellular Effects
The effects of lead molybdate on cells and cellular processes are still being explored. Its photocatalytic activity suggests that it could influence cell function, potentially impacting cell signaling pathways, gene expression, and cellular metabolism .
Molecular Mechanism
At the molecular level, lead molybdate exerts its effects through its photocatalytic activity. This involves binding interactions with biomolecules, potential enzyme inhibition or activation, and changes in gene expression .
Metabolic Pathways
Lead molybdate’s involvement in metabolic pathways is another area of active research. It could potentially interact with various enzymes or cofactors, and may influence metabolic flux or metabolite levels .
Vorbereitungsmethoden
Synthetic Routes and Reaction Conditions: Lead molybdate can be synthesized through several methods, including:
Co-precipitation Method: This involves reacting lead acetate trihydrate with ammonium heptamolybdate tetrahydrate in an aqueous solution.
Hydrothermal Method: This method involves the reaction of lead nitrate and sodium molybdate in a hydrothermal reactor at elevated temperatures and pressures.
Microwave-Assisted Hydrothermal Route: This method uses microwave radiation to heat the reaction mixture, leading to the formation of lead molybdate with controlled particle size and morphology.
Industrial Production Methods: In industrial settings, lead molybdate is often produced using large-scale hydrothermal synthesis due to its efficiency and ability to produce high-purity crystals. The reaction conditions, such as temperature, pressure, and reactant concentrations, are carefully controlled to optimize yield and product quality .
Analyse Chemischer Reaktionen
Lead molybdate undergoes various chemical reactions, including:
Oxidation and Reduction: Lead molybdate can participate in redox reactions, particularly in the presence of cerium ions.
Photocatalytic Reactions: Lead molybdate exhibits photocatalytic activity under ultraviolet light.
Substitution Reactions: Lead molybdate can undergo substitution reactions where lead ions are replaced by other metal ions, altering its properties and applications.
Common Reagents and Conditions:
Oxidizing Agents: Cerium (IV) ions are commonly used in redox reactions involving lead molybdate.
Reducing Agents: Hydrogen peroxide and other reducing agents can be used in photocatalytic reduction reactions.
Reaction Conditions: These reactions typically occur under controlled pH and temperature conditions to ensure optimal performance and product formation.
Major Products Formed:
Photocatalytic Reduction Products: The reduction of hexavalent chromium results in the formation of trivalent chromium, which is less toxic and more environmentally friendly.
Substitution Products: Substitution reactions can lead to the formation of various lead molybdate derivatives with different metal ions, each exhibiting unique properties.
Vergleich Mit ähnlichen Verbindungen
Lead molybdate can be compared with other molybdate compounds, such as:
Calcium Molybdate (CaMoO₄): Similar to lead molybdate, calcium molybdate is used in photocatalysis and optoelectronics.
Sodium Molybdate (Na₂MoO₄): Sodium molybdate is more soluble in water and is commonly used in industrial applications as a corrosion inhibitor and in agriculture as a micronutrient.
Zinc Molybdate (ZnMoO₄): Zinc molybdate is used in flame retardants and pigments.
Uniqueness of Lead Molybdate: Lead molybdate’s unique combination of optical, electronic, and photocatalytic properties makes it a versatile material for various applications. Its ability to undergo redox reactions and its high stability under different conditions further enhance its utility in scientific research and industrial applications .
Biologische Aktivität
Lead molybdate () is an inorganic compound that has garnered attention due to its unique properties and potential biological activities. This compound is primarily studied in the context of its role as a lead salt in various applications, including its use in phosphors and as a reagent in analytical chemistry. However, understanding its biological activity is crucial for assessing its environmental and health implications.
The biological activity of lead molybdate is closely tied to the behavior of molybdenum (Mo) in biological systems. Molybdenum is an essential trace element that plays a critical role in various enzymatic processes. In organisms, molybdenum must be complexed with pterin compounds to form the molybdenum cofactor (Moco), which is vital for the activity of several enzymes, including:
- Sulfite oxidase : Involved in sulfur metabolism.
- Xanthine oxidase : Plays a role in purine degradation.
- Aldehyde oxidase : Involved in the oxidation of aldehydes.
Lead molybdate, when introduced into biological systems, may influence these enzymatic pathways either positively or negatively depending on concentration and exposure duration.
Toxicological Profile
The toxicological profile of lead molybdate is significant due to the presence of lead, a well-known toxic heavy metal. Studies indicate that exposure to lead compounds can result in various health issues, including neurotoxicity, reproductive toxicity, and developmental disorders. The relationship between lead exposure and metabolic disorders has been documented, highlighting the need for careful consideration of lead molybdate's biological effects.
Key Findings from Toxicological Studies
- Metabolic Disruption : Exposure to molybdate has been shown to disrupt metabolic processes in organisms. A study noted significant changes in serum metabolites, indicating potential metabolic disorders linked to molybdate exposure .
- Enzymatic Activity : The presence of lead may inhibit the activity of molybdoenzymes, potentially leading to deficiencies in critical metabolic pathways .
Case Studies
- Plant Growth Inhibition : Research involving Arabidopsis thaliana demonstrated that molybdate deprivation resulted in reduced growth and impaired enzyme activity associated with nitrogen metabolism . This highlights the dual role of molybdate as both an essential nutrient and a potential inhibitor when present as lead molybdate.
- Animal Studies : In rodent models, exposure to sodium molybdate resulted in alterations in copper metabolism without significant acute toxicity at low concentrations . These findings suggest that while molybdate itself can be beneficial at trace levels, its interaction with other metals like lead complicates its biological activity.
Summary of Biological Effects
Eigenschaften
IUPAC Name |
dioxido(dioxo)molybdenum;lead(2+) | |
---|---|---|
Source | PubChem | |
URL | https://pubchem.ncbi.nlm.nih.gov | |
Description | Data deposited in or computed by PubChem | |
InChI |
InChI=1S/Mo.4O.Pb/q;;;2*-1;+2 | |
Source | PubChem | |
URL | https://pubchem.ncbi.nlm.nih.gov | |
Description | Data deposited in or computed by PubChem | |
InChI Key |
XJUNRGGMKUAPAP-UHFFFAOYSA-N | |
Source | PubChem | |
URL | https://pubchem.ncbi.nlm.nih.gov | |
Description | Data deposited in or computed by PubChem | |
Canonical SMILES |
[O-][Mo](=O)(=O)[O-].[Pb+2] | |
Source | PubChem | |
URL | https://pubchem.ncbi.nlm.nih.gov | |
Description | Data deposited in or computed by PubChem | |
Molecular Formula |
MoO4Pb | |
Source | PubChem | |
URL | https://pubchem.ncbi.nlm.nih.gov | |
Description | Data deposited in or computed by PubChem | |
Molecular Weight |
367 g/mol | |
Source | PubChem | |
URL | https://pubchem.ncbi.nlm.nih.gov | |
Description | Data deposited in or computed by PubChem | |
Physical Description |
Yellow solid; [Merck Index] | |
Record name | Lead molybdate | |
Source | Haz-Map, Information on Hazardous Chemicals and Occupational Diseases | |
URL | https://haz-map.com/Agents/2538 | |
Description | Haz-Map® is an occupational health database designed for health and safety professionals and for consumers seeking information about the adverse effects of workplace exposures to chemical and biological agents. | |
Explanation | Copyright (c) 2022 Haz-Map(R). All rights reserved. Unless otherwise indicated, all materials from Haz-Map are copyrighted by Haz-Map(R). No part of these materials, either text or image may be used for any purpose other than for personal use. Therefore, reproduction, modification, storage in a retrieval system or retransmission, in any form or by any means, electronic, mechanical or otherwise, for reasons other than personal use, is strictly prohibited without prior written permission. | |
CAS No. |
10190-55-3 | |
Record name | Lead molybdate(VI) | |
Source | ChemIDplus | |
URL | https://pubchem.ncbi.nlm.nih.gov/substance/?source=chemidplus&sourceid=0010190553 | |
Description | ChemIDplus is a free, web search system that provides access to the structure and nomenclature authority files used for the identification of chemical substances cited in National Library of Medicine (NLM) databases, including the TOXNET system. | |
Record name | Lead molybdenum oxide (PbMoO4) | |
Source | EPA Chemicals under the TSCA | |
URL | https://www.epa.gov/chemicals-under-tsca | |
Description | EPA Chemicals under the Toxic Substances Control Act (TSCA) collection contains information on chemicals and their regulations under TSCA, including non-confidential content from the TSCA Chemical Substance Inventory and Chemical Data Reporting. | |
Record name | Lead molybdate | |
Source | European Chemicals Agency (ECHA) | |
URL | https://echa.europa.eu/substance-information/-/substanceinfo/100.030.405 | |
Description | The European Chemicals Agency (ECHA) is an agency of the European Union which is the driving force among regulatory authorities in implementing the EU's groundbreaking chemicals legislation for the benefit of human health and the environment as well as for innovation and competitiveness. | |
Explanation | Use of the information, documents and data from the ECHA website is subject to the terms and conditions of this Legal Notice, and subject to other binding limitations provided for under applicable law, the information, documents and data made available on the ECHA website may be reproduced, distributed and/or used, totally or in part, for non-commercial purposes provided that ECHA is acknowledged as the source: "Source: European Chemicals Agency, http://echa.europa.eu/". Such acknowledgement must be included in each copy of the material. ECHA permits and encourages organisations and individuals to create links to the ECHA website under the following cumulative conditions: Links can only be made to webpages that provide a link to the Legal Notice page. | |
Record name | LEAD MOLYBDATE | |
Source | FDA Global Substance Registration System (GSRS) | |
URL | https://gsrs.ncats.nih.gov/ginas/app/beta/substances/WGP849K028 | |
Description | The FDA Global Substance Registration System (GSRS) enables the efficient and accurate exchange of information on what substances are in regulated products. Instead of relying on names, which vary across regulatory domains, countries, and regions, the GSRS knowledge base makes it possible for substances to be defined by standardized, scientific descriptions. | |
Explanation | Unless otherwise noted, the contents of the FDA website (www.fda.gov), both text and graphics, are not copyrighted. They are in the public domain and may be republished, reprinted and otherwise used freely by anyone without the need to obtain permission from FDA. Credit to the U.S. Food and Drug Administration as the source is appreciated but not required. | |
Haftungsausschluss und Informationen zu In-Vitro-Forschungsprodukten
Bitte beachten Sie, dass alle Artikel und Produktinformationen, die auf BenchChem präsentiert werden, ausschließlich zu Informationszwecken bestimmt sind. Die auf BenchChem zum Kauf angebotenen Produkte sind speziell für In-vitro-Studien konzipiert, die außerhalb lebender Organismen durchgeführt werden. In-vitro-Studien, abgeleitet von dem lateinischen Begriff "in Glas", beinhalten Experimente, die in kontrollierten Laborumgebungen unter Verwendung von Zellen oder Geweben durchgeführt werden. Es ist wichtig zu beachten, dass diese Produkte nicht als Arzneimittel oder Medikamente eingestuft sind und keine Zulassung der FDA für die Vorbeugung, Behandlung oder Heilung von medizinischen Zuständen, Beschwerden oder Krankheiten erhalten haben. Wir müssen betonen, dass jede Form der körperlichen Einführung dieser Produkte in Menschen oder Tiere gesetzlich strikt untersagt ist. Es ist unerlässlich, sich an diese Richtlinien zu halten, um die Einhaltung rechtlicher und ethischer Standards in Forschung und Experiment zu gewährleisten.