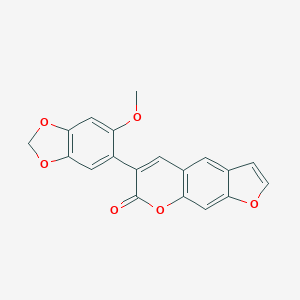
Pachyrrhizin
Übersicht
Beschreibung
Pachyrrhizin ist eine Isoflavonoidverbindung, die zur Klasse der organischen Verbindungen gehört, die als Isoflav-3-enone bekannt sind. Diese Verbindungen zeichnen sich durch eine Struktur aus, die auf einem Isoflav-3-en-Gerüst basiert, das an Position C2 eine Oxogruppe trägt.
Vorbereitungsmethoden
Synthetische Routen und Reaktionsbedingungen
Die Synthese von Pachyrrhizin umfasst mehrere Schritte, beginnend mit leicht verfügbaren Vorläufern. Die synthetische Route beinhaltet typischerweise die Bildung des Isoflav-3-enon-Gerüsts durch Cyclisierungsreaktionen, gefolgt von der Einführung von funktionellen Gruppen an bestimmten Positionen. Die Reaktionsbedingungen beinhalten oft die Verwendung von Katalysatoren, Lösungsmitteln und kontrollierten Temperaturen, um das gewünschte Produkt zu erhalten.
Industrielle Produktionsverfahren
Die industrielle Produktion von this compound kann die Extraktion der Verbindung aus natürlichen Quellen wie den Samen von Pachyrhizus erosus beinhalten. Das Extraktionsverfahren umfasst die Lösungsmittelextraktion, Reinigung und Kristallisation, um reines this compound zu erhalten. Fortschrittliche Techniken wie Chromatographie und Spektroskopie werden verwendet, um die Reinheit und Qualität der Verbindung zu gewährleisten.
Chemische Reaktionsanalyse
Arten von Reaktionen
This compound unterliegt verschiedenen chemischen Reaktionen, darunter:
Oxidation: this compound kann oxidiert werden, um verschiedene Oxidationsprodukte zu bilden.
Reduktion: Reduktionsreaktionen können this compound in reduzierte Formen mit unterschiedlichen biologischen Aktivitäten umwandeln.
Substitution: Substitutionsreaktionen beinhalten den Austausch bestimmter funktioneller Gruppen im this compound-Molekül.
Häufige Reagenzien und Bedingungen
Häufige Reagenzien, die in den Reaktionen von this compound verwendet werden, umfassen Oxidationsmittel, Reduktionsmittel und Katalysatoren. Die Reaktionsbedingungen variieren je nach dem gewünschten Produkt und können bestimmte Temperaturen, Drücke und Lösungsmittel umfassen.
Hauptprodukte, die gebildet werden
Die Hauptprodukte, die aus den Reaktionen von this compound gebildet werden, umfassen verschiedene Derivate mit veränderten biologischen Aktivitäten
Wissenschaftliche Forschungsanwendungen
This compound hat in verschiedenen wissenschaftlichen Forschungsanwendungen bemerkenswerte Vielseitigkeit gezeigt, darunter:
Chemie: this compound wird als Vorläufer für die Synthese anderer Isoflavonoidverbindungen verwendet.
Biologie: Es wird wegen seiner antioxidativen, immunmodulatorischen und Antikrebsaktivitäten untersucht.
Industrie: Die Verbindung wird bei der Entwicklung von funktionellen Lebensmitteln und Nutrazeutika verwendet
Wirkmechanismus
Der Wirkmechanismus von this compound beinhaltet seine Wechselwirkung mit spezifischen molekularen Zielen und Signalwegen. This compound übt seine Wirkungen durch die Modulation von Signalwegen aus, die mit oxidativem Stress, Entzündungen und Zellproliferation zusammenhängen. Die antioxidativen Eigenschaften der Verbindung tragen dazu bei, freie Radikale abzufangen, während ihre immunmodulatorischen Wirkungen die Abwehrmechanismen des Körpers verbessern .
Analyse Chemischer Reaktionen
Types of Reactions
Pachyrrhizin undergoes various chemical reactions, including:
Oxidation: this compound can be oxidized to form different oxidation products.
Reduction: Reduction reactions can convert this compound into reduced forms with different biological activities.
Substitution: Substitution reactions involve the replacement of specific functional groups in the this compound molecule.
Common Reagents and Conditions
Common reagents used in the reactions of this compound include oxidizing agents, reducing agents, and catalysts. The reaction conditions vary depending on the desired product and may include specific temperatures, pressures, and solvents.
Major Products Formed
The major products formed from the reactions of this compound include various derivatives with altered biological activities
Wissenschaftliche Forschungsanwendungen
Pachyrrhizin has shown remarkable versatility in various scientific research applications, including:
Chemistry: this compound is used as a precursor for the synthesis of other isoflavonoid compounds.
Biology: It is studied for its antioxidant, immune modulation, and anticancer properties.
Industry: The compound is used in the development of functional foods and nutraceuticals
Wirkmechanismus
The mechanism of action of pachyrrhizin involves its interaction with specific molecular targets and pathways. This compound exerts its effects through the modulation of signaling pathways related to oxidative stress, inflammation, and cell proliferation. The compound’s antioxidant properties help in scavenging free radicals, while its immune modulation effects enhance the body’s defense mechanisms .
Vergleich Mit ähnlichen Verbindungen
Pachyrrhizin ist unter den Isoflavonoidverbindungen einzigartig aufgrund seiner spezifischen Struktur und biologischen Aktivitäten. Ähnliche Verbindungen umfassen:
Rotenon: Bekannt für seine insektiziden Eigenschaften.
Munduseron: Wird wegen seiner Antikrebsaktivitäten untersucht.
Biologische Aktivität
Pachyrrhizin is a naturally occurring compound primarily extracted from the seeds of the yam bean (Pachyrhizus erosus), a member of the Fabaceae family. This compound has garnered attention due to its diverse biological activities, including potential applications in medicinal and agricultural fields. This article aims to provide a comprehensive overview of the biological activity of this compound, supported by case studies, data tables, and relevant research findings.
Chemical Structure and Properties
This compound is characterized by its complex chemical structure, which includes various functional groups that contribute to its bioactivity. The molecular formula for this compound is . Its structural similarity to other bioactive compounds, such as isoflavones and coumarins, suggests a potential for diverse pharmacological effects .
Antimicrobial Activity
This compound exhibits significant antimicrobial properties against various pathogens. A study demonstrated that extracts containing this compound effectively inhibited the growth of Sclerotium rolfsii and Ralstonia solanacearum, two significant agricultural pathogens. At a concentration of 1:1000, this compound reduced the mycelial growth of S. rolfsii by 73% after five days . In another instance, it reduced bacterial multiplication of R. solanacearum by 57% within 48 hours .
Antioxidant Properties
The antioxidant capacity of this compound has been highlighted in various studies, indicating its potential role in protecting cells from oxidative stress. The presence of flavonoids and other phenolic compounds in yam bean extracts contributes to this antioxidant activity . The ability to scavenge free radicals makes this compound a candidate for further research in the context of chronic diseases linked to oxidative damage.
Anticancer Effects
Research indicates that this compound may possess anticancer properties. A study noted its efficacy against human cancer cell lines, suggesting that it could inhibit cancer cell proliferation through mechanisms involving apoptosis induction . However, detailed clinical studies are still needed to establish these effects conclusively.
Immunomodulatory Effects
This compound has shown potential in modulating immune responses. In vivo studies have indicated that it can enhance immune function, making it a candidate for further exploration as an immunotherapeutic agent .
Data Table: Biological Activities of this compound
Case Study 1: Toxicity Assessment
A notable case involved the toxicity assessment of yam bean seeds containing this compound. The study revealed that mature seeds exhibited high lethality rates (100%) compared to controls (27%) when tested on various organisms, highlighting the necessity for caution in their use . This underscores the dual nature of this compound as both beneficial and potentially harmful.
Case Study 2: Agricultural Applications
In agricultural research, extracts from yam bean seeds have been utilized as biopesticides due to their effectiveness against plant pathogens. The findings support the development of natural pest control methods that could reduce reliance on synthetic pesticides .
Eigenschaften
CAS-Nummer |
10091-01-7 |
---|---|
Molekularformel |
C19H12O6 |
Molekulargewicht |
336.3 g/mol |
IUPAC-Name |
6-(6-methoxy-1,3-benzodioxol-5-yl)furo[3,2-g]chromen-7-one |
InChI |
InChI=1S/C19H12O6/c1-21-16-8-18-17(23-9-24-18)6-12(16)13-5-11-4-10-2-3-22-14(10)7-15(11)25-19(13)20/h2-8H,9H2,1H3 |
InChI-Schlüssel |
PENSQRMNZZWMGV-UHFFFAOYSA-N |
SMILES |
COC1=CC2=C(C=C1C3=CC4=C(C=C5C(=C4)C=CO5)OC3=O)OCO2 |
Kanonische SMILES |
COC1=CC2=C(C=C1C3=CC4=C(C=C5C(=C4)C=CO5)OC3=O)OCO2 |
melting_point |
207 - 209 °C |
Key on ui other cas no. |
10091-01-7 |
Physikalische Beschreibung |
Solid |
Herkunft des Produkts |
United States |
Retrosynthesis Analysis
AI-Powered Synthesis Planning: Our tool employs the Template_relevance Pistachio, Template_relevance Bkms_metabolic, Template_relevance Pistachio_ringbreaker, Template_relevance Reaxys, Template_relevance Reaxys_biocatalysis model, leveraging a vast database of chemical reactions to predict feasible synthetic routes.
One-Step Synthesis Focus: Specifically designed for one-step synthesis, it provides concise and direct routes for your target compounds, streamlining the synthesis process.
Accurate Predictions: Utilizing the extensive PISTACHIO, BKMS_METABOLIC, PISTACHIO_RINGBREAKER, REAXYS, REAXYS_BIOCATALYSIS database, our tool offers high-accuracy predictions, reflecting the latest in chemical research and data.
Strategy Settings
Precursor scoring | Relevance Heuristic |
---|---|
Min. plausibility | 0.01 |
Model | Template_relevance |
Template Set | Pistachio/Bkms_metabolic/Pistachio_ringbreaker/Reaxys/Reaxys_biocatalysis |
Top-N result to add to graph | 6 |
Feasible Synthetic Routes
Haftungsausschluss und Informationen zu In-Vitro-Forschungsprodukten
Bitte beachten Sie, dass alle Artikel und Produktinformationen, die auf BenchChem präsentiert werden, ausschließlich zu Informationszwecken bestimmt sind. Die auf BenchChem zum Kauf angebotenen Produkte sind speziell für In-vitro-Studien konzipiert, die außerhalb lebender Organismen durchgeführt werden. In-vitro-Studien, abgeleitet von dem lateinischen Begriff "in Glas", beinhalten Experimente, die in kontrollierten Laborumgebungen unter Verwendung von Zellen oder Geweben durchgeführt werden. Es ist wichtig zu beachten, dass diese Produkte nicht als Arzneimittel oder Medikamente eingestuft sind und keine Zulassung der FDA für die Vorbeugung, Behandlung oder Heilung von medizinischen Zuständen, Beschwerden oder Krankheiten erhalten haben. Wir müssen betonen, dass jede Form der körperlichen Einführung dieser Produkte in Menschen oder Tiere gesetzlich strikt untersagt ist. Es ist unerlässlich, sich an diese Richtlinien zu halten, um die Einhaltung rechtlicher und ethischer Standards in Forschung und Experiment zu gewährleisten.