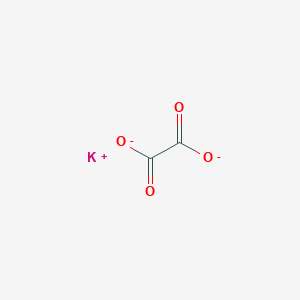
Ethanedioic acid,potassium salt (1:?)
Übersicht
Beschreibung
Ethanedioic acid,potassium salt (1:?), also known as potassium oxalate, is a chemical compound with the formula K2C2O4. It is the potassium salt of oxalic acid, a dicarboxylic acid. This compound is commonly found in its monohydrate form, which means it contains one molecule of water per molecule of potassium oxalate. Potassium oxalate is a white crystalline solid that is soluble in water and has various applications in different fields.
Vorbereitungsmethoden
Synthetic Routes and Reaction Conditions
Potassium oxalate can be synthesized by reacting oxalic acid with potassium hydroxide. The reaction is as follows:
H2C2O4+2KOH→K2C2O4+2H2O
This reaction typically occurs in an aqueous solution, and the resulting potassium oxalate can be crystallized out by evaporating the water.
Industrial Production Methods
Industrially, potassium oxalate is produced by neutralizing oxalic acid with potassium carbonate or potassium hydroxide. The reaction with potassium carbonate is as follows:
H2C2O4+K2CO3→K2C2O4+H2O+CO2
The product is then purified and crystallized to obtain the desired compound.
Analyse Chemischer Reaktionen
Types of Reactions
Potassium oxalate undergoes various chemical reactions, including:
Oxidation: Potassium oxalate can be oxidized by strong oxidizing agents such as potassium permanganate in acidic conditions to produce carbon dioxide and water.
Reduction: It can be reduced to formic acid and carbon monoxide under specific conditions.
Substitution: Potassium oxalate can participate in substitution reactions where the oxalate ion is replaced by other anions.
Common Reagents and Conditions
Oxidation: Potassium permanganate in acidic medium.
Reduction: Catalysts such as palladium or platinum under high pressure and temperature.
Substitution: Various metal salts in aqueous solutions.
Major Products Formed
Oxidation: Carbon dioxide and water.
Reduction: Formic acid and carbon monoxide.
Substitution: Metal oxalates and other salts.
Wissenschaftliche Forschungsanwendungen
Potassium oxalate has numerous applications in scientific research:
Chemistry: Used as a reagent in redox titrations and as a standard for calibrating solutions.
Biology: Employed in studies involving calcium oxalate crystals, which are relevant to kidney stone research.
Medicine: Investigated for its potential role in reducing calcium oxalate kidney stones.
Industry: Utilized in the preparation of catalysts, cleaning agents, and as a mordant in dyeing processes.
Wirkmechanismus
Potassium oxalate exerts its effects primarily through its ability to form complexes with metal ions. The oxalate ion can chelate metal ions, making it useful in various applications such as metal cleaning and as a reagent in analytical chemistry. In biological systems, oxalate can bind to calcium ions, forming insoluble calcium oxalate, which is a major component of kidney stones.
Vergleich Mit ähnlichen Verbindungen
Similar Compounds
Sodium oxalate: Similar in structure but contains sodium instead of potassium.
Calcium oxalate: Insoluble in water and forms kidney stones.
Ammonium oxalate: Used in similar applications but contains ammonium ions.
Uniqueness
Potassium oxalate is unique due to its high solubility in water compared to calcium oxalate, making it more suitable for applications requiring soluble oxalate salts. Its ability to form stable complexes with various metal ions also distinguishes it from other oxalate salts.
Biologische Aktivität
Ethanedioic acid, commonly known as oxalic acid, and its potassium salt forms have garnered significant attention in various fields, including biochemistry, medicine, and agriculture. This article delves into the biological activities associated with Ethanedioic acid, potassium salt (1:?) , focusing on its biochemical interactions, physiological effects, and potential applications.
Chemical Overview
Ethanedioic acid, potassium salt (2:1), dihydrate has the chemical formula and is also referred to as potassium tetraoxalate dihydrate. It is a crystalline compound used primarily as a pH buffer in analytical chemistry and various industrial applications .
Target of Action: The primary biological activity of potassium tetraoxalate involves its role as a pH buffer. By maintaining stable pH levels in biological systems, it facilitates optimal conditions for enzymatic reactions and metabolic processes.
Mode of Action: The compound acts by neutralizing excess acids or bases in a solution. This buffering capacity is crucial in biological systems where pH fluctuations can lead to impaired cellular functions.
Result of Action: The effective buffering action helps maintain homeostasis in biological environments, which is essential for the proper functioning of cells and metabolic pathways.
Toxicological Profile
Ethanedioic acid and its salts, including potassium oxalate, exhibit varying degrees of toxicity. The primary concern arises from the formation of calcium oxalate crystals in the kidneys, which can lead to renal dysfunction and kidney stones .
- Acute Toxicity: Potassium oxalate has been classified as harmful if ingested or if it comes into contact with skin . The lethal dose for oxalic acid is reported to be around 15-30 grams for humans .
- Chronic Effects: Long-term exposure may lead to cumulative toxic effects, particularly affecting renal function due to calcium oxalate precipitation.
Metabolic Pathways
The oxalate anion produced from the dissociation of ethanedioic acid plays a significant role in various metabolic processes. It can act as a competitive inhibitor of lactate dehydrogenase (LDH), an enzyme critical for anaerobic metabolism. Inhibition of LDH has been linked to reduced tumor growth in cancer cells that rely on anaerobic metabolism for energy .
Applications in Research and Medicine
Ethanedioic acid, potassium salt has several applications across different fields:
- Biochemistry: Used as a standard for calibrating pH measurements and studying metal ion complexation.
- Medicine: Investigated for its potential role in chelation therapy for heavy metal poisoning. Its ability to bind with metal ions makes it useful in detoxification processes .
- Agriculture: Plays a role in plant defense mechanisms against pathogens. Small amounts can enhance plant resistance to fungal infections .
Case Studies
-
Kidney Stone Formation:
A study demonstrated that high dietary intake of oxalic acid correlates with increased risk of calcium oxalate kidney stones. The study analyzed dietary habits and urine composition among participants, revealing that individuals consuming high levels of oxalates had significantly higher urinary oxalate concentrations . -
Cancer Metabolism:
Research explored the effects of oxalic acid on cancer cell metabolism. It was found that inhibiting LDH with oxalic acid derivatives could reduce lactate production and impede tumor growth, suggesting a potential therapeutic avenue for cancer treatment .
Comparative Analysis
Compound | Chemical Formula | Biological Activity | Toxicity Level |
---|---|---|---|
Ethanedioic Acid | Inhibits LDH; forms kidney stones | Moderate to High | |
Potassium Oxalate | Chelation therapy; pH buffering | Moderate | |
Sodium Tetraoxalate | Similar to potassium salt; used in analytical chemistry | Moderate |
Eigenschaften
IUPAC Name |
potassium;oxalate | |
---|---|---|
Source | PubChem | |
URL | https://pubchem.ncbi.nlm.nih.gov | |
Description | Data deposited in or computed by PubChem | |
InChI |
InChI=1S/C2H2O4.K/c3-1(4)2(5)6;/h(H,3,4)(H,5,6);/q;+1/p-2 | |
Source | PubChem | |
URL | https://pubchem.ncbi.nlm.nih.gov | |
Description | Data deposited in or computed by PubChem | |
InChI Key |
JMTCDHVHZSGGJA-UHFFFAOYSA-L | |
Source | PubChem | |
URL | https://pubchem.ncbi.nlm.nih.gov | |
Description | Data deposited in or computed by PubChem | |
Canonical SMILES |
C(=O)(C(=O)[O-])[O-].[K+] | |
Source | PubChem | |
URL | https://pubchem.ncbi.nlm.nih.gov | |
Description | Data deposited in or computed by PubChem | |
Molecular Formula |
C2KO4- | |
Source | PubChem | |
URL | https://pubchem.ncbi.nlm.nih.gov | |
Description | Data deposited in or computed by PubChem | |
Molecular Weight |
127.12 g/mol | |
Source | PubChem | |
URL | https://pubchem.ncbi.nlm.nih.gov | |
Description | Data deposited in or computed by PubChem | |
CAS No. |
10043-22-8 | |
Record name | Ethanedioic acid, potassium salt (1:?) | |
Source | ChemIDplus | |
URL | https://pubchem.ncbi.nlm.nih.gov/substance/?source=chemidplus&sourceid=0010043228 | |
Description | ChemIDplus is a free, web search system that provides access to the structure and nomenclature authority files used for the identification of chemical substances cited in National Library of Medicine (NLM) databases, including the TOXNET system. | |
Record name | Ethanedioic acid, potassium salt (1:?) | |
Source | EPA Chemicals under the TSCA | |
URL | https://www.epa.gov/chemicals-under-tsca | |
Description | EPA Chemicals under the Toxic Substances Control Act (TSCA) collection contains information on chemicals and their regulations under TSCA, including non-confidential content from the TSCA Chemical Substance Inventory and Chemical Data Reporting. | |
Record name | Oxalic acid, potassium salt | |
Source | European Chemicals Agency (ECHA) | |
URL | https://echa.europa.eu/substance-information/-/substanceinfo/100.030.112 | |
Description | The European Chemicals Agency (ECHA) is an agency of the European Union which is the driving force among regulatory authorities in implementing the EU's groundbreaking chemicals legislation for the benefit of human health and the environment as well as for innovation and competitiveness. | |
Explanation | Use of the information, documents and data from the ECHA website is subject to the terms and conditions of this Legal Notice, and subject to other binding limitations provided for under applicable law, the information, documents and data made available on the ECHA website may be reproduced, distributed and/or used, totally or in part, for non-commercial purposes provided that ECHA is acknowledged as the source: "Source: European Chemicals Agency, http://echa.europa.eu/". Such acknowledgement must be included in each copy of the material. ECHA permits and encourages organisations and individuals to create links to the ECHA website under the following cumulative conditions: Links can only be made to webpages that provide a link to the Legal Notice page. | |
Retrosynthesis Analysis
AI-Powered Synthesis Planning: Our tool employs the Template_relevance Pistachio, Template_relevance Bkms_metabolic, Template_relevance Pistachio_ringbreaker, Template_relevance Reaxys, Template_relevance Reaxys_biocatalysis model, leveraging a vast database of chemical reactions to predict feasible synthetic routes.
One-Step Synthesis Focus: Specifically designed for one-step synthesis, it provides concise and direct routes for your target compounds, streamlining the synthesis process.
Accurate Predictions: Utilizing the extensive PISTACHIO, BKMS_METABOLIC, PISTACHIO_RINGBREAKER, REAXYS, REAXYS_BIOCATALYSIS database, our tool offers high-accuracy predictions, reflecting the latest in chemical research and data.
Strategy Settings
Precursor scoring | Relevance Heuristic |
---|---|
Min. plausibility | 0.01 |
Model | Template_relevance |
Template Set | Pistachio/Bkms_metabolic/Pistachio_ringbreaker/Reaxys/Reaxys_biocatalysis |
Top-N result to add to graph | 6 |
Feasible Synthetic Routes
Haftungsausschluss und Informationen zu In-Vitro-Forschungsprodukten
Bitte beachten Sie, dass alle Artikel und Produktinformationen, die auf BenchChem präsentiert werden, ausschließlich zu Informationszwecken bestimmt sind. Die auf BenchChem zum Kauf angebotenen Produkte sind speziell für In-vitro-Studien konzipiert, die außerhalb lebender Organismen durchgeführt werden. In-vitro-Studien, abgeleitet von dem lateinischen Begriff "in Glas", beinhalten Experimente, die in kontrollierten Laborumgebungen unter Verwendung von Zellen oder Geweben durchgeführt werden. Es ist wichtig zu beachten, dass diese Produkte nicht als Arzneimittel oder Medikamente eingestuft sind und keine Zulassung der FDA für die Vorbeugung, Behandlung oder Heilung von medizinischen Zuständen, Beschwerden oder Krankheiten erhalten haben. Wir müssen betonen, dass jede Form der körperlichen Einführung dieser Produkte in Menschen oder Tiere gesetzlich strikt untersagt ist. Es ist unerlässlich, sich an diese Richtlinien zu halten, um die Einhaltung rechtlicher und ethischer Standards in Forschung und Experiment zu gewährleisten.