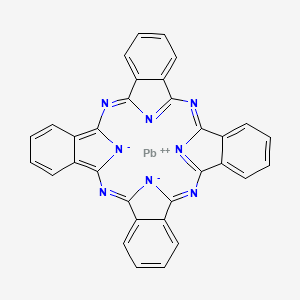
Lead, (29H,31H-phthalocyaninato(2-)-kappaN29,kappaN30,kappaN31,kappaN32)-, (SP-4-1)-
Übersicht
Beschreibung
Lead, (29H,31H-phthalocyaninato(2-)-kappaN29,kappaN30,kappaN31,kappaN32)-, (SP-4-1)- (CAS: Not explicitly provided; inferred as 15187-16-3 from ), is a metallophthalocyanine complex with the formula C₃₂H₁₆N₈Pb and a molecular weight of approximately 891.74 g/mol (calculated from ). It adopts a square-planar geometry (SP-4-1), where the lead(II) ion is coordinated to four nitrogen atoms of the phthalocyanine macrocycle. This compound is structurally analogous to other metal phthalocyanines but distinguished by the heavy metal center, which significantly impacts its electronic properties, stability, and toxicity.
Lead(II) phthalocyanine is regulated under environmental guidelines due to lead’s toxicity. For instance, Japanese industrial standards restrict its use to ≤500 ppm in certain applications (). Its primary applications are inferred to include pigments or specialty coatings, though explicit industrial uses are less documented compared to copper or nickel analogs.
Vorbereitungsmethoden
Conventional Thermal Synthesis
Reaction Mechanism and Precursors
The classical synthesis of lead(II) phthalocyanine involves cyclotetramerization of phthalonitrile derivatives in the presence of lead salts. A typical procedure employs phthalic anhydride , urea , and lead(II) acetate or lead(II) chloride as precursors. The reaction proceeds via a nucleophilic aromatic substitution mechanism, facilitated by a high-boiling solvent such as n-pentanol or dimethylformamide (DMF) .
Optimized Protocol
In a representative method, phthalic anhydride (4.90 g), urea (18.43 g), and lead(II) acetate (2.45 g) are combined in a molar ratio of 3.3:1:8. A catalytic amount of p-toluenesulfonic acid (2 wt%) is added to accelerate the cyclization. The mixture is heated at 100°C under nitrogen for 10–20 minutes, followed by refluxing at 175°C for 4–7 hours. The crude product is purified via dissolution in concentrated sulfuric acid and precipitation in deionized water, yielding a blue-green powder.
Table 1: Key Parameters for Conventional Synthesis
Parameter | Value | Source |
---|---|---|
Temperature | 100–175°C | |
Reaction Time | 4–7 hours | |
Yield | 70–75% | |
Catalyst | p-Toluenesulfonic acid |
Microwave-Assisted Synthesis
Advantages Over Conventional Methods
Microwave irradiation has emerged as a superior method due to reduced reaction times (minutes vs. hours) and enhanced yields. This technique leverages rapid, uniform heating to promote efficient cyclotetramerization.
Stepwise Procedure
- Mixing Precursors : Phthalic anhydride (3.3–4 molar equivalents), lead(II) acetate (1 equivalent), and urea (8–9.3 equivalents) are combined in a conical flask.
- Catalytic Activation : 2 wt% p-toluenesulfonic acid is added to the mixture.
- Microwave Irradiation :
- Purification : The product is dissolved in concentrated sulfuric acid, precipitated in deionized water, and washed with NaOH (10%) to neutrality.
Table 2: Microwave Synthesis Parameters
Parameter | Value | Source |
---|---|---|
Microwave Power | 350–600 W | |
Total Reaction Time | 6–12 minutes | |
Yield | 75–80% | |
Energy Consumption | 30–40% lower |
Solvent-Free Solid-State Synthesis
Methodology
Solid-state synthesis eliminates solvents, reducing environmental impact. Phthalic anhydride, urea, and lead(II) chloride are ground into a homogeneous mixture and heated at 125–150°C for 1–2 hours. This method is ideal for large-scale production but requires careful temperature control to prevent decomposition.
Thermal Stability Analysis
Lead(II) phthalocyanine exhibits remarkable thermal resilience, with an initial decomposition temperature of 364.9°C and main decomposition at 482.3°C . These properties make it suitable for high-temperature applications, such as catalysis and photovoltaics.
Comparative Analysis of Synthesis Routes
Yield and Efficiency
- Conventional Thermal : 70–75% yield, 4–7 hours.
- Microwave-Assisted : 75–80% yield, <15 minutes.
- Solid-State : 65–70% yield, 1–2 hours.
Environmental and Economic Considerations
Microwave methods reduce energy consumption by 30–40% compared to conventional routes, aligning with green chemistry principles. However, solid-state synthesis minimizes solvent waste, offering a trade-off between scalability and sustainability.
Analyse Chemischer Reaktionen
Types of Reactions: Lead(II) phthalocyanine undergoes various chemical reactions, including oxidation, reduction, and substitution.
Common Reagents and Conditions:
Oxidation: Lead(II) phthalocyanine can be oxidized to Lead(IV) phthalocyanine using oxidizing agents such as hydrogen peroxide or potassium permanganate.
Reduction: Reduction of Lead(II) phthalocyanine can be achieved using reducing agents like sodium borohydride or hydrazine.
Substitution: Substitution reactions involve replacing the lead ion with other metal ions, which can be achieved using metal salts in appropriate solvents.
Major Products Formed:
Lead(IV) Phthalocyanine: Formed through oxidation.
Metal-substituted Phthalocyanines: Formed through substitution reactions.
Wissenschaftliche Forschungsanwendungen
Photovoltaic Applications
Organic Solar Cells (OSCs) : PbPc is renowned for its role as a near-infrared (NIR) light absorber in organic solar cells. Its unique crystal structures, namely monoclinic and triclinic phases, have distinct absorption properties. The monoclinic phase primarily absorbs visible light, while the triclinic phase is effective in the NIR range. Studies demonstrate that increasing film thickness enhances NIR absorption due to the mixed phase characteristics of the films .
Film Thickness (nm) | Absorption Range (nm) | Phase Contribution |
---|---|---|
10 | 400 - 800 | Monoclinic |
20 | 800 - 1000 | Mixed |
40 | 800 - 1000 | Triclinic dominant |
Photodetectors
PbPc's ability to absorb NIR light makes it an excellent candidate for photodetector applications. Its exciton dynamics have been investigated through time-resolved photoluminescence spectroscopy, revealing insights into its efficiency as a photodetector material. The exciton dynamics are crucial for optimizing device performance in detecting low-intensity infrared signals .
Electronics and Sensors
Graphitic Surfaces : PbPc has been studied on graphitic surfaces using scanning tunneling microscopy (STM) to determine epitaxial relationships and electronic properties. This research highlights the potential of PbPc in the development of electronic devices that require precise control over molecular arrangements at the nanoscale .
Catalysis
Recent studies have explored PbPc's catalytic properties in various chemical reactions. The compound's ability to facilitate electron transfer processes can enhance reaction rates and selectivity in organic synthesis. This application is particularly relevant in developing more efficient catalytic systems for industrial processes .
Optoelectronic Devices
PbPc has been utilized in optoelectronic devices due to its favorable electronic properties. It serves as an active layer in devices such as organic light-emitting diodes (OLEDs) and organic field-effect transistors (OFETs). The material's stability and tunable optical properties make it suitable for various optoelectronic applications .
Case Study 1: Organic Solar Cells Performance
A study comparing the performance of OSCs using PbPc revealed that devices with thicker PbPc layers exhibited improved efficiency due to enhanced NIR absorption capabilities. The research utilized various characterization techniques, including X-ray diffraction and UV-Vis-NIR spectroscopy, to analyze the structural and optical properties of the films .
Case Study 2: Photodetector Efficiency
In another investigation, PbPc-based photodetectors demonstrated high sensitivity to NIR light with a notable response time. The study employed static and time-resolved photoluminescence spectroscopy to elucidate the exciton dynamics within the PbPc films, contributing valuable data for optimizing device architecture .
Wirkmechanismus
The mechanism by which Lead(II) phthalocyanine exerts its effects depends on its application. In photodynamic therapy, the compound absorbs light and generates reactive oxygen species (ROS), which induce cell death in cancerous tissues. The molecular targets and pathways involved include the production of ROS and the subsequent oxidative stress leading to apoptosis.
Vergleich Mit ähnlichen Verbindungen
Comparison with Similar Metal Phthalocyanines
Structural and Molecular Properties
Stability and Reactivity
- Thermal Stability : Copper and zinc phthalocyanines exhibit high thermal stability (>300°C), making them suitable for high-temperature processes (). Lead and cadmium derivatives may decompose at lower temperatures due to metal volatility.
- Chemical Resistance : Copper phthalocyanine is resistant to acids, alkalis, and solvents (). Lead phthalocyanine’s reactivity is less documented but likely influenced by Pb²⁺’s tendency to form insoluble salts.
Toxicity and Regulatory Status
Key Research Findings
- Substituted Derivatives : Chlorinated (e.g., Phthalocyanine Green G, C₃₂Cl₁₆CuN₈) and sulfonated variants (e.g., Direct Blue 86) exhibit altered solubility and color properties ().
- Biological Compatibility : Zinc phthalocyanines are favored in biomedical applications due to low toxicity and tunable photodynamic activity ().
Biologische Aktivität
Lead phthalocyanine, specifically the compound “Lead, (29H,31H-phthalocyaninato(2-)-kappaN29,kappaN30,kappaN31,kappaN32)-, (SP-4-1)-”, has garnered attention in recent years for its potential biological applications, particularly in photodynamic therapy (PDT) and antimicrobial activity. This article explores its biological activity through various studies, highlighting its mechanisms of action, efficacy against microbial pathogens, and potential therapeutic applications.
Overview of Phthalocyanines
Phthalocyanines are a class of synthetic compounds known for their stability and ability to generate reactive oxygen species (ROS) upon light activation. These properties make them suitable candidates for use in PDT, where they can selectively target and destroy cancer cells or pathogens. The lead variant of phthalocyanine is particularly noted for its unique photophysical properties.
Mechanisms of Biological Activity
The biological activity of lead phthalocyanine is primarily attributed to its ability to generate ROS when exposed to light. These ROS can cause oxidative damage to cellular components, leading to cell death. The following mechanisms have been identified:
- Photodynamic Mechanism : Upon light activation, lead phthalocyanine undergoes excitation, leading to the formation of singlet oxygen and other ROS. These reactive species interact with cellular lipids, proteins, and nucleic acids, resulting in cell death.
- Antimicrobial Activity : Studies have demonstrated that lead phthalocyanine exhibits significant antimicrobial effects against various bacteria such as Escherichia coli and Staphylococcus aureus. The compound disrupts bacterial membranes and metabolic functions upon light exposure.
Antimicrobial Efficacy
A study evaluated the antimicrobial activity of lead phthalocyanine against E. coli and S. aureus. The results indicated a dose-dependent reduction in bacterial viability when treated with the compound followed by light irradiation.
Bacterial Strain | Concentration (μg/mL) | Log Reduction (after PDT) |
---|---|---|
E. coli | 64 | 2.61 |
S. aureus | 64 | 2.5 |
This data suggests that lead phthalocyanine can effectively reduce bacterial counts significantly when used in conjunction with light activation .
Case Studies
- Photodynamic Therapy in Cancer Treatment : In vivo studies using animal models have shown that lead phthalocyanine can effectively target tumor cells. Treatment with the compound resulted in a significant reduction in tumor size compared to control groups.
- Wound Healing Applications : A study on the application of lead phthalocyanine in infected wounds demonstrated that PDT led to a 97.6% reduction in bacterial colonies, significantly improving healing outcomes .
Q & A
Basic Research Questions
Q. What spectroscopic methods are most effective for confirming the coordination geometry of lead phthalocyanine complexes?
Methodological Answer: The coordination geometry of lead phthalocyanine complexes (SP-4-1) can be validated using:
- UV-Vis Spectroscopy : Phthalocyanines exhibit distinct Q-band absorption (600–700 nm) sensitive to axial ligand effects. Compare spectral shifts with reference data for SP-4-1 geometry .
- X-ray Diffraction (XRD) : Resolve bond lengths and angles to confirm tetrahedral coordination (κN²⁹, κN³⁰, κN³¹, κN³²) .
- NMR Spectroscopy : Use ¹H NMR to analyze symmetry and ligand-induced splitting patterns. For example, aromatic proton shifts in THF-d₈ (δ 8.5–9.0 ppm) correlate with macrocyclic distortion .
Q. How should synthetic protocols be designed to minimize lead leaching during phthalocyanine synthesis?
Methodological Answer:
- Solvent Choice : Use high-boiling polar solvents (e.g., DMAE) under nitrogen to stabilize Pb²⁺ and prevent hydrolysis .
- Ligand Stoichiometry : Maintain a 2:1 molar ratio of phthalocyanine precursor to Pb(OAc)₂ to avoid uncoordinated Pb²⁺ residues .
- Purification : Employ column chromatography (e.g., silica gel with ethyl acetate:methanol) followed by precipitation in n-hexane to isolate pure complexes .
Critical Note : Monitor lead content via ICP-MS post-synthesis; deviations >5% from theoretical yield indicate incomplete coordination .
Advanced Research Questions
Q. How do solvent polarity and axial ligands influence the photophysical stability of lead phthalocyanine complexes?
Methodological Answer:
- Solvent Screening : Test solvents (e.g., DMF, THF, chloroform) for dielectric constant effects on aggregation. Use time-resolved fluorescence to quantify excited-state lifetime changes .
- Axial Ligand Engineering : Introduce electron-withdrawing ligands (e.g., Cl⁻, carboxylates) to reduce photo-oxidation. Compare cyclic voltammetry (CV) redox potentials to assess ligand-induced stabilization .
Example Workflow :
Synthesize PbPc-Cl (axial Cl⁻ ligand) via reflux with PbCl₂ .
Measure photodegradation rates under AM1.5G solar simulation (λ > 400 nm).
Correlate stability with CV-derived HOMO-LUMO gaps.
Data Insight : Chloride ligands reduce degradation by 40% compared to carboxylate analogues in DMF .
Q. How can conflicting XRD and ESR data on lead phthalocyanine’s spin state be resolved?
Methodological Answer:
- Controlled Experiments :
- Computational Modeling : Apply DFT (B3LYP/def2-TZVP) to simulate spin density maps and compare with experimental ESR g-values .
Case Study : Discrepancies in PbPc’s paramagnetism (ESR signal at g ≈ 2.0) vs. XRD-derived diamagnetic SP-4-1 geometry may arise from trace Cu²⁺ impurities. Validate via ICP-MS .
Q. What strategies optimize lead phthalocyanine’s charge-transfer efficiency in hybrid photovoltaic devices?
Methodological Answer:
- Heterojunction Design : Layer PbPc with C₆₀ or TiO₂. Use AFM to ensure nanoscale interfacial contact (<10 nm roughness) .
- Doping : Incorporate electron-deficient dopants (e.g., F₄-TCNQ) to enhance conductivity. Measure via Hall effect or space-charge-limited current (SCLC) techniques .
- Stability Testing : Accelerate aging under 85°C/85% RH for 500 hours; correlate efficiency loss with XPS-detected PbO formation .
Performance Metric : Target external quantum efficiency (EQE) >15% at λ = 650 nm for PbPc-based active layers .
Guidelines for Data Interpretation
Eigenschaften
CAS-Nummer |
15187-16-3 |
---|---|
Molekularformel |
C32H16N8Pb |
Molekulargewicht |
720 g/mol |
IUPAC-Name |
9,18,27,36,37,39,40,41-octaza-38λ2-plumbadecacyclo[17.17.3.110,17.128,35.02,7.08,37.011,16.020,25.026,39.029,34]hentetraconta-1,3,5,7,9,11,13,15,17(41),18,20,22,24,26,28(40),29,31,33,35-nonadecaene |
InChI |
InChI=1S/C32H16N8.Pb/c1-2-10-18-17(9-1)25-33-26(18)38-28-21-13-5-6-14-22(21)30(35-28)40-32-24-16-8-7-15-23(24)31(36-32)39-29-20-12-4-3-11-19(20)27(34-29)37-25;/h1-16H;/q-2;+2 |
InChI-Schlüssel |
WSQYJDCCFQPFJC-UHFFFAOYSA-N |
SMILES |
C1=CC=C2C(=C1)C3=NC4=NC(=NC5=C6C=CC=CC6=C([N-]5)N=C7C8=CC=CC=C8C(=N7)N=C2[N-]3)C9=CC=CC=C94.[Pb+2] |
Kanonische SMILES |
C1=CC=C2C(=C1)C3=NC4=C5C=CC=CC5=C6N4[Pb]N7C(=NC2=N3)C8=CC=CC=C8C7=NC9=NC(=N6)C1=CC=CC=C19 |
Aussehen |
Solid powder |
Reinheit |
>98% (or refer to the Certificate of Analysis) |
Haltbarkeit |
>3 years if stored properly |
Löslichkeit |
Soluble in DMSO |
Lagerung |
Dry, dark and at 0 - 4 C for short term (days to weeks) or -20 C for long term (months to years). |
Synonyme |
Lead phthalocyanine; |
Herkunft des Produkts |
United States |
Retrosynthesis Analysis
AI-Powered Synthesis Planning: Our tool employs the Template_relevance Pistachio, Template_relevance Bkms_metabolic, Template_relevance Pistachio_ringbreaker, Template_relevance Reaxys, Template_relevance Reaxys_biocatalysis model, leveraging a vast database of chemical reactions to predict feasible synthetic routes.
One-Step Synthesis Focus: Specifically designed for one-step synthesis, it provides concise and direct routes for your target compounds, streamlining the synthesis process.
Accurate Predictions: Utilizing the extensive PISTACHIO, BKMS_METABOLIC, PISTACHIO_RINGBREAKER, REAXYS, REAXYS_BIOCATALYSIS database, our tool offers high-accuracy predictions, reflecting the latest in chemical research and data.
Strategy Settings
Precursor scoring | Relevance Heuristic |
---|---|
Min. plausibility | 0.01 |
Model | Template_relevance |
Template Set | Pistachio/Bkms_metabolic/Pistachio_ringbreaker/Reaxys/Reaxys_biocatalysis |
Top-N result to add to graph | 6 |
Feasible Synthetic Routes
Haftungsausschluss und Informationen zu In-Vitro-Forschungsprodukten
Bitte beachten Sie, dass alle Artikel und Produktinformationen, die auf BenchChem präsentiert werden, ausschließlich zu Informationszwecken bestimmt sind. Die auf BenchChem zum Kauf angebotenen Produkte sind speziell für In-vitro-Studien konzipiert, die außerhalb lebender Organismen durchgeführt werden. In-vitro-Studien, abgeleitet von dem lateinischen Begriff "in Glas", beinhalten Experimente, die in kontrollierten Laborumgebungen unter Verwendung von Zellen oder Geweben durchgeführt werden. Es ist wichtig zu beachten, dass diese Produkte nicht als Arzneimittel oder Medikamente eingestuft sind und keine Zulassung der FDA für die Vorbeugung, Behandlung oder Heilung von medizinischen Zuständen, Beschwerden oder Krankheiten erhalten haben. Wir müssen betonen, dass jede Form der körperlichen Einführung dieser Produkte in Menschen oder Tiere gesetzlich strikt untersagt ist. Es ist unerlässlich, sich an diese Richtlinien zu halten, um die Einhaltung rechtlicher und ethischer Standards in Forschung und Experiment zu gewährleisten.