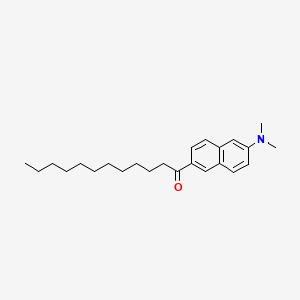
Laurdan
Übersicht
Beschreibung
Laurdan is an organic compound used as a fluorescent dye in fluorescence microscopy . It is used to investigate the qualities of the phospholipid bilayers of cell membranes . One of its most important characteristics is its sensitivity to membrane phase transitions and other alterations to membrane fluidity such as the penetration of water . Laurdan was first synthesized in 1979 by the Argentinian scientist Gregorio Weber .
Synthesis Analysis
A convenient and versatile synthesis of Laurdan-like fluorescent membrane probes has been reported . A simple and versatile two-step synthetic scheme leads to Laurdan analogs differing by their polar head .Molecular Structure Analysis
Laurdan is composed of a chain of lauric fatty acid (hydrophobic) linked to a naphthalene molecule . Because of a partial charge separation between the 2-dimethylamino and the 6-carbonyl residues, the naphthalene moiety has a dipole moment, which increases upon excitation and causes the reorientation of the surrounding solvent dipoles .Chemical Reactions Analysis
The fluorescent properties of Laurdan are sensitive to the physical state of the lipid bilayer . Laurdan signal intensity and shape of the emission spectrum change with temperature .Physical And Chemical Properties Analysis
Laurdan has a molecular weight of 353.55 and appears as a colorless solid . It is soluble in DMF, acetonitrile, and methanol . Its melting point is 88 °C .Wissenschaftliche Forschungsanwendungen
Imaging Lipid Order in Cell Plasma Membranes
Laurdan has been used as a solvatochromic probe for imaging lipid order selectively in cell plasma membranes . This application provides information about the organization of lipids, their ordering, and uneven distribution . A key drawback of Laurdan linked to its rapid internalization and subsequent labeling of internal membranes was addressed by redesigning it with a membrane anchor group based on negatively charged sulfonate and dodecyl chain .
Measurement of Cell Membrane Fluidity
Laurdan has been utilized for the measurement of cell membrane fluidity through fluorescence spectroscopy and microscopy . The fluorescence emission spectrum of Laurdan is sensitive to the presence of H2O close to its chromophore . This allows for straightforward measurement of antibiotic compound-triggered changes in membrane fluidity both in vivo and in vitro .
Analysis of Lipid Domains
Laurdan can be used to analyze lipid domains and lipid packing . Changes in the overall membrane fluidity or triggering the formation of abnormal lipid domains by an antimicrobial compound can inhibit cell growth . Laurdan can help in analyzing these important cellular parameters .
Study of Lipid Adaptation
Laurdan can be used to study lipid adaptation . Numerous antimicrobial compounds target bacterial cytoplasmic membranes and disrupt the normal function of this essential cellular structure . Laurdan can help in understanding the mechanisms of action of this category of antimicrobials .
Analysis of Membrane Viscosity
Laurdan can be used to analyze membrane viscosity . A correct fluidity state of the membrane is equally important in order to support the multitude of membrane-associated cellular processes . Laurdan can help in analyzing these important cellular parameters .
Study of Membrane Targeting Antimicrobials
Laurdan can be used to study membrane targeting antimicrobials . Not all membrane targeting antimicrobials trigger permeabilization of cellular membranes . Laurdan can help in understanding the mechanisms of action of this category of antimicrobials .
Characterization of Cell Organelles
Cell organelles feature characteristic lipid compositions that lead to differences in membrane properties . Laurdan, whose fluorescence is sensitive to membrane packing, is commonly used to measure membrane ordering and fluidity in living cells .
Analysis of Heterogeneous Embedding in Lipid Bilayers
Laurdan is incorporated in a heterogeneous fashion in both DOPC and DPPC bilayers, and its fluorescence depends on the details of this embedding . This allows for the simulation of absorption and emission spectra of Laurdan in both DOPC and DPPC bilayers with quantum chemical and classical molecular dynamics methods .
Wirkmechanismus
Target of Action
Laurdan, or 6-dodecanoyl-2-dimethylaminonaphthalene, is a synthetic, environmentally sensitive dye . Its primary target is the cellular membrane , specifically the lipid bilayer . The functional group of Laurdan is physically located at the carbonyl region of the bilayer . Due to its amphiphilic nature, laurdan can insert into lipid bilayers at different depths and orientations .
Mode of Action
Laurdan’s interaction with its target results in changes in membrane fluidity . Membrane fluidity is a critical parameter of cellular membranes, which cells continuously strive to maintain within a viable range . An interference with the correct membrane fluidity state can strongly inhibit cell function . Laurdan’s fluorescence emission spectrum is sensitive to the presence of H2O close to its chromophore , allowing it to detect changes in membrane fluidity.
Biochemical Pathways
Laurdan’s action primarily affects the biochemical pathways related to membrane fluidity and permeability . By interacting with the lipid bilayer, Laurdan can influence the diffusion barrier function of the cytoplasmic membrane . This can lead to changes in the overall membrane fluidity or trigger the formation of abnormal lipid domains , potentially inhibiting cell growth .
Pharmacokinetics
It is known that laurdan is essentially nonfluorescent in water but becomes fluorescent when incorporated into a lipid bilayer .
Result of Action
The primary result of Laurdan’s action is the alteration of membrane fluidity . This can lead to changes in the overall membrane fluidity or the formation of abnormal lipid domains . These changes can strongly inhibit cell function , affecting a multitude of membrane-associated cellular processes .
Action Environment
The action of Laurdan can be influenced by various environmental factors. For instance, cholesterol levels in lipid vesicles can alter membrane hydration and viscosity , affecting Laurdan’s action. Moreover, Laurdan is designed to stain exclusively the outer leaflet of lipid bilayers , making it more sensitive to changes in the external environment.
Zukünftige Richtungen
Laurdan has been extensively used in biological relevant systems . Its value in the study of membrane structure and dynamics is reflected in more than 330 papers reported in the existing literature . Future research may continue to leverage Laurdan’s unique properties to gain further insights into membrane dynamics .
Eigenschaften
IUPAC Name |
1-[6-(dimethylamino)naphthalen-2-yl]dodecan-1-one | |
---|---|---|
Source | PubChem | |
URL | https://pubchem.ncbi.nlm.nih.gov | |
Description | Data deposited in or computed by PubChem | |
InChI |
InChI=1S/C24H35NO/c1-4-5-6-7-8-9-10-11-12-13-24(26)22-15-14-21-19-23(25(2)3)17-16-20(21)18-22/h14-19H,4-13H2,1-3H3 | |
Source | PubChem | |
URL | https://pubchem.ncbi.nlm.nih.gov | |
Description | Data deposited in or computed by PubChem | |
InChI Key |
JHDGGIDITFLRJY-UHFFFAOYSA-N | |
Source | PubChem | |
URL | https://pubchem.ncbi.nlm.nih.gov | |
Description | Data deposited in or computed by PubChem | |
Canonical SMILES |
CCCCCCCCCCCC(=O)C1=CC2=C(C=C1)C=C(C=C2)N(C)C | |
Source | PubChem | |
URL | https://pubchem.ncbi.nlm.nih.gov | |
Description | Data deposited in or computed by PubChem | |
Molecular Formula |
C24H35NO | |
Source | PubChem | |
URL | https://pubchem.ncbi.nlm.nih.gov | |
Description | Data deposited in or computed by PubChem | |
DSSTOX Substance ID |
DTXSID80225517 | |
Record name | Laurdan | |
Source | EPA DSSTox | |
URL | https://comptox.epa.gov/dashboard/DTXSID80225517 | |
Description | DSSTox provides a high quality public chemistry resource for supporting improved predictive toxicology. | |
Molecular Weight |
353.5 g/mol | |
Source | PubChem | |
URL | https://pubchem.ncbi.nlm.nih.gov | |
Description | Data deposited in or computed by PubChem | |
Product Name |
Laurdan | |
CAS RN |
74515-25-6 | |
Record name | 1-[6-(Dimethylamino)-2-naphthalenyl]-1-dodecanone | |
Source | CAS Common Chemistry | |
URL | https://commonchemistry.cas.org/detail?cas_rn=74515-25-6 | |
Description | CAS Common Chemistry is an open community resource for accessing chemical information. Nearly 500,000 chemical substances from CAS REGISTRY cover areas of community interest, including common and frequently regulated chemicals, and those relevant to high school and undergraduate chemistry classes. This chemical information, curated by our expert scientists, is provided in alignment with our mission as a division of the American Chemical Society. | |
Explanation | The data from CAS Common Chemistry is provided under a CC-BY-NC 4.0 license, unless otherwise stated. | |
Record name | Laurdan | |
Source | ChemIDplus | |
URL | https://pubchem.ncbi.nlm.nih.gov/substance/?source=chemidplus&sourceid=0074515256 | |
Description | ChemIDplus is a free, web search system that provides access to the structure and nomenclature authority files used for the identification of chemical substances cited in National Library of Medicine (NLM) databases, including the TOXNET system. | |
Record name | Laurdan | |
Source | EPA DSSTox | |
URL | https://comptox.epa.gov/dashboard/DTXSID80225517 | |
Description | DSSTox provides a high quality public chemistry resource for supporting improved predictive toxicology. | |
Record name | LAURDAN | |
Source | FDA Global Substance Registration System (GSRS) | |
URL | https://gsrs.ncats.nih.gov/ginas/app/beta/substances/Y97FBL93VW | |
Description | The FDA Global Substance Registration System (GSRS) enables the efficient and accurate exchange of information on what substances are in regulated products. Instead of relying on names, which vary across regulatory domains, countries, and regions, the GSRS knowledge base makes it possible for substances to be defined by standardized, scientific descriptions. | |
Explanation | Unless otherwise noted, the contents of the FDA website (www.fda.gov), both text and graphics, are not copyrighted. They are in the public domain and may be republished, reprinted and otherwise used freely by anyone without the need to obtain permission from FDA. Credit to the U.S. Food and Drug Administration as the source is appreciated but not required. | |
Synthesis routes and methods
Procedure details
Retrosynthesis Analysis
AI-Powered Synthesis Planning: Our tool employs the Template_relevance Pistachio, Template_relevance Bkms_metabolic, Template_relevance Pistachio_ringbreaker, Template_relevance Reaxys, Template_relevance Reaxys_biocatalysis model, leveraging a vast database of chemical reactions to predict feasible synthetic routes.
One-Step Synthesis Focus: Specifically designed for one-step synthesis, it provides concise and direct routes for your target compounds, streamlining the synthesis process.
Accurate Predictions: Utilizing the extensive PISTACHIO, BKMS_METABOLIC, PISTACHIO_RINGBREAKER, REAXYS, REAXYS_BIOCATALYSIS database, our tool offers high-accuracy predictions, reflecting the latest in chemical research and data.
Strategy Settings
Precursor scoring | Relevance Heuristic |
---|---|
Min. plausibility | 0.01 |
Model | Template_relevance |
Template Set | Pistachio/Bkms_metabolic/Pistachio_ringbreaker/Reaxys/Reaxys_biocatalysis |
Top-N result to add to graph | 6 |
Feasible Synthetic Routes
Haftungsausschluss und Informationen zu In-Vitro-Forschungsprodukten
Bitte beachten Sie, dass alle Artikel und Produktinformationen, die auf BenchChem präsentiert werden, ausschließlich zu Informationszwecken bestimmt sind. Die auf BenchChem zum Kauf angebotenen Produkte sind speziell für In-vitro-Studien konzipiert, die außerhalb lebender Organismen durchgeführt werden. In-vitro-Studien, abgeleitet von dem lateinischen Begriff "in Glas", beinhalten Experimente, die in kontrollierten Laborumgebungen unter Verwendung von Zellen oder Geweben durchgeführt werden. Es ist wichtig zu beachten, dass diese Produkte nicht als Arzneimittel oder Medikamente eingestuft sind und keine Zulassung der FDA für die Vorbeugung, Behandlung oder Heilung von medizinischen Zuständen, Beschwerden oder Krankheiten erhalten haben. Wir müssen betonen, dass jede Form der körperlichen Einführung dieser Produkte in Menschen oder Tiere gesetzlich strikt untersagt ist. Es ist unerlässlich, sich an diese Richtlinien zu halten, um die Einhaltung rechtlicher und ethischer Standards in Forschung und Experiment zu gewährleisten.