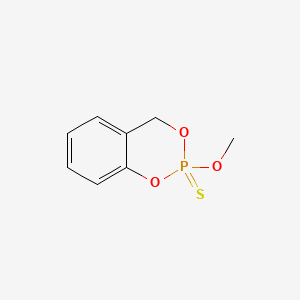
Dioxabenzofos
Übersicht
Beschreibung
Wissenschaftliche Forschungsanwendungen
Dioxabenzofos has been used in various scientific research applications, including:
Wirkmechanismus
Target of Action
Dioxabenzofos, also known as Salithion, is an organophosphate insecticide . Its primary target is the enzyme acetylcholinesterase . Acetylcholinesterase plays a crucial role in nerve function by breaking down the neurotransmitter acetylcholine, which is essential for transmitting signals in the nervous system .
Mode of Action
This compound acts as a cholinesterase inhibitor . It binds to acetylcholinesterase, preventing it from breaking down acetylcholine . This leads to an accumulation of acetylcholine in the synaptic cleft, causing overstimulation of the nervous system . The compound is non-systemic, exerting its effects through contact, ingestion, and respiratory pathways .
Biochemical Pathways
As a cholinesterase inhibitor, it disrupts the normal functioning of the cholinergic nervous system . This disruption can lead to a range of downstream effects, including overstimulation of muscles and glands, and potential neurotoxicity .
Pharmacokinetics
Like other organophosphates, it is likely to be absorbed through the skin, lungs, and gastrointestinal tract, distributed throughout the body, metabolized in the liver, and excreted via the kidneys .
Result of Action
The primary result of this compound action is the overstimulation of the nervous system due to the accumulation of acetylcholine . This can lead to a range of symptoms, including muscle twitching, weakness, and potentially paralysis . At high enough doses, it can cause respiratory failure and death .
Action Environment
The action of this compound can be influenced by various environmental factors. For example, its efficacy as an insecticide can be affected by temperature, humidity, and the presence of other chemicals . Its stability can also be influenced by factors such as pH and light exposure . .
Biochemische Analyse
Biochemical Properties
Dioxabenzofos plays a significant role in biochemical reactions as an acetylcholinesterase inhibitor . This compound interacts with the enzyme acetylcholinesterase, which is crucial for the breakdown of the neurotransmitter acetylcholine in synaptic clefts. By inhibiting this enzyme, this compound causes an accumulation of acetylcholine, leading to continuous nerve signal transmission, which can be lethal to insects . Additionally, this compound may interact with other proteins and biomolecules involved in nerve signal transmission, although specific interactions beyond acetylcholinesterase inhibition are less well-documented.
Cellular Effects
This compound affects various types of cells, particularly nerve cells, by disrupting normal cellular processes. The compound’s inhibition of acetylcholinesterase leads to excessive accumulation of acetylcholine, which overstimulates nerve cells and disrupts normal cell signaling pathways . This overstimulation can result in symptoms such as muscle twitching, paralysis, and eventually death in insects. In mammalian cells, similar effects can occur, leading to neurotoxicity and potential long-term damage to the nervous system .
Molecular Mechanism
At the molecular level, this compound exerts its effects primarily through the inhibition of acetylcholinesterase . The compound binds to the active site of the enzyme, preventing it from hydrolyzing acetylcholine into acetate and choline. This binding is typically irreversible, leading to prolonged inhibition of the enzyme and sustained accumulation of acetylcholine . This mechanism of action is similar to other organophosphate insecticides, which also target acetylcholinesterase to exert their toxic effects.
Temporal Effects in Laboratory Settings
In laboratory settings, the effects of this compound can change over time due to factors such as stability and degradation. The compound is known to have a limited shelf life and can degrade under certain conditions, which may reduce its effectiveness . Long-term exposure to this compound in in vitro and in vivo studies has shown that the compound can cause persistent neurotoxic effects, including prolonged inhibition of acetylcholinesterase and continuous overstimulation of nerve cells . These effects can lead to long-term damage to the nervous system and other cellular functions.
Dosage Effects in Animal Models
The effects of this compound vary with different dosages in animal models. At low doses, the compound may cause mild symptoms such as muscle twitching and slight behavioral changes . At higher doses, this compound can cause severe neurotoxic effects, including paralysis, convulsions, and death . Threshold effects have been observed, where a certain dosage level leads to a significant increase in toxicity. Additionally, high doses of this compound can cause toxic effects on other organ systems, including the liver and kidneys .
Metabolic Pathways
This compound is involved in several metabolic pathways, primarily related to its detoxification and elimination from the body. The compound is metabolized by liver enzymes, including cytochrome P450 enzymes, which convert it into less toxic metabolites . These metabolites are then further processed and excreted from the body through urine and feces. The metabolic pathways of this compound also involve interactions with various cofactors and enzymes that facilitate its breakdown and elimination .
Transport and Distribution
Within cells and tissues, this compound is transported and distributed through various mechanisms. The compound can cross cell membranes and accumulate in nerve tissues, where it exerts its toxic effects The distribution of this compound within the body can affect its localization and accumulation in target tissues, influencing its overall toxicity .
Subcellular Localization
This compound is primarily localized in nerve cells, where it targets acetylcholinesterase in synaptic clefts . The compound’s activity is influenced by its subcellular localization, as it needs to reach the active site of acetylcholinesterase to exert its inhibitory effects.
Vorbereitungsmethoden
Synthetic Routes and Reaction Conditions
Dioxabenzofos can be synthesized through a series of chemical reactions involving the formation of a benzodioxaphosphinine ring.
Industrial Production Methods
Industrial production of this compound involves similar synthetic routes but on a larger scale. The process requires stringent control of reaction conditions such as temperature, pressure, and pH to ensure high yield and purity of the final product .
Analyse Chemischer Reaktionen
Types of Reactions
Dioxabenzofos undergoes several types of chemical reactions, including:
Oxidation: The compound can be oxidized to form various oxidation products.
Reduction: Reduction reactions can convert this compound into its corresponding reduced forms.
Common Reagents and Conditions
Oxidation: Common oxidizing agents include hydrogen peroxide and potassium permanganate.
Reduction: Reducing agents such as sodium borohydride and lithium aluminum hydride are used.
Substitution: Nucleophiles like amines and thiols are commonly used in substitution reactions.
Major Products Formed
The major products formed from these reactions depend on the specific reagents and conditions used. For example, oxidation can lead to the formation of sulfoxides and sulfones, while substitution reactions can yield various substituted benzodioxaphosphinine derivatives .
Vergleich Mit ähnlichen Verbindungen
Similar Compounds
Methoxyfenozide: Another insecticide with a similar mode of action but different chemical structure.
Pyriproxyfen: An insect growth regulator that affects the development of insects.
Triazophos: An organophosphate insecticide with a similar mechanism of action but different chemical properties.
Uniqueness
Dioxabenzofos is unique due to its specific chemical structure, which includes a benzodioxaphosphinine ring. This structure contributes to its specific mode of action and its effectiveness as an insecticide. Additionally, its chiral nature and the presence of stereoisomers add to its complexity and potential for varied biological activity .
Eigenschaften
IUPAC Name |
2-methoxy-2-sulfanylidene-4H-1,3,2λ5-benzodioxaphosphinine | |
---|---|---|
Source | PubChem | |
URL | https://pubchem.ncbi.nlm.nih.gov | |
Description | Data deposited in or computed by PubChem | |
InChI |
InChI=1S/C8H9O3PS/c1-9-12(13)10-6-7-4-2-3-5-8(7)11-12/h2-5H,6H2,1H3 | |
Source | PubChem | |
URL | https://pubchem.ncbi.nlm.nih.gov | |
Description | Data deposited in or computed by PubChem | |
InChI Key |
OUNSASXJZHBGAI-UHFFFAOYSA-N | |
Source | PubChem | |
URL | https://pubchem.ncbi.nlm.nih.gov | |
Description | Data deposited in or computed by PubChem | |
Canonical SMILES |
COP1(=S)OCC2=CC=CC=C2O1 | |
Source | PubChem | |
URL | https://pubchem.ncbi.nlm.nih.gov | |
Description | Data deposited in or computed by PubChem | |
Molecular Formula |
C8H9O3PS | |
Source | PubChem | |
URL | https://pubchem.ncbi.nlm.nih.gov | |
Description | Data deposited in or computed by PubChem | |
DSSTOX Substance ID |
DTXSID7041885 | |
Record name | Dioxabenzofos | |
Source | EPA DSSTox | |
URL | https://comptox.epa.gov/dashboard/DTXSID7041885 | |
Description | DSSTox provides a high quality public chemistry resource for supporting improved predictive toxicology. | |
Molecular Weight |
216.20 g/mol | |
Source | PubChem | |
URL | https://pubchem.ncbi.nlm.nih.gov | |
Description | Data deposited in or computed by PubChem | |
CAS No. |
3811-49-2, 90293-10-0, 90293-16-6 | |
Record name | Salithion | |
Source | CAS Common Chemistry | |
URL | https://commonchemistry.cas.org/detail?cas_rn=3811-49-2 | |
Description | CAS Common Chemistry is an open community resource for accessing chemical information. Nearly 500,000 chemical substances from CAS REGISTRY cover areas of community interest, including common and frequently regulated chemicals, and those relevant to high school and undergraduate chemistry classes. This chemical information, curated by our expert scientists, is provided in alignment with our mission as a division of the American Chemical Society. | |
Explanation | The data from CAS Common Chemistry is provided under a CC-BY-NC 4.0 license, unless otherwise stated. | |
Record name | Dioxabenzofos [BSI:ISO] | |
Source | ChemIDplus | |
URL | https://pubchem.ncbi.nlm.nih.gov/substance/?source=chemidplus&sourceid=0003811492 | |
Description | ChemIDplus is a free, web search system that provides access to the structure and nomenclature authority files used for the identification of chemical substances cited in National Library of Medicine (NLM) databases, including the TOXNET system. | |
Record name | (S)-Salithion | |
Source | ChemIDplus | |
URL | https://pubchem.ncbi.nlm.nih.gov/substance/?source=chemidplus&sourceid=0090293100 | |
Description | ChemIDplus is a free, web search system that provides access to the structure and nomenclature authority files used for the identification of chemical substances cited in National Library of Medicine (NLM) databases, including the TOXNET system. | |
Record name | (R)-Salithion | |
Source | ChemIDplus | |
URL | https://pubchem.ncbi.nlm.nih.gov/substance/?source=chemidplus&sourceid=0090293166 | |
Description | ChemIDplus is a free, web search system that provides access to the structure and nomenclature authority files used for the identification of chemical substances cited in National Library of Medicine (NLM) databases, including the TOXNET system. | |
Record name | Dioxabenzofos | |
Source | EPA DSSTox | |
URL | https://comptox.epa.gov/dashboard/DTXSID7041885 | |
Description | DSSTox provides a high quality public chemistry resource for supporting improved predictive toxicology. | |
Record name | 2-methoxy-4H-1,3,2-benzodioxaphosphorin 2-sulphide | |
Source | European Chemicals Agency (ECHA) | |
URL | https://echa.europa.eu/substance-information/-/substanceinfo/100.021.176 | |
Description | The European Chemicals Agency (ECHA) is an agency of the European Union which is the driving force among regulatory authorities in implementing the EU's groundbreaking chemicals legislation for the benefit of human health and the environment as well as for innovation and competitiveness. | |
Explanation | Use of the information, documents and data from the ECHA website is subject to the terms and conditions of this Legal Notice, and subject to other binding limitations provided for under applicable law, the information, documents and data made available on the ECHA website may be reproduced, distributed and/or used, totally or in part, for non-commercial purposes provided that ECHA is acknowledged as the source: "Source: European Chemicals Agency, http://echa.europa.eu/". Such acknowledgement must be included in each copy of the material. ECHA permits and encourages organisations and individuals to create links to the ECHA website under the following cumulative conditions: Links can only be made to webpages that provide a link to the Legal Notice page. | |
Record name | DIOXABENZOFOS | |
Source | FDA Global Substance Registration System (GSRS) | |
URL | https://gsrs.ncats.nih.gov/ginas/app/beta/substances/6ZWK96BZW6 | |
Description | The FDA Global Substance Registration System (GSRS) enables the efficient and accurate exchange of information on what substances are in regulated products. Instead of relying on names, which vary across regulatory domains, countries, and regions, the GSRS knowledge base makes it possible for substances to be defined by standardized, scientific descriptions. | |
Explanation | Unless otherwise noted, the contents of the FDA website (www.fda.gov), both text and graphics, are not copyrighted. They are in the public domain and may be republished, reprinted and otherwise used freely by anyone without the need to obtain permission from FDA. Credit to the U.S. Food and Drug Administration as the source is appreciated but not required. | |
Synthesis routes and methods
Procedure details
Retrosynthesis Analysis
AI-Powered Synthesis Planning: Our tool employs the Template_relevance Pistachio, Template_relevance Bkms_metabolic, Template_relevance Pistachio_ringbreaker, Template_relevance Reaxys, Template_relevance Reaxys_biocatalysis model, leveraging a vast database of chemical reactions to predict feasible synthetic routes.
One-Step Synthesis Focus: Specifically designed for one-step synthesis, it provides concise and direct routes for your target compounds, streamlining the synthesis process.
Accurate Predictions: Utilizing the extensive PISTACHIO, BKMS_METABOLIC, PISTACHIO_RINGBREAKER, REAXYS, REAXYS_BIOCATALYSIS database, our tool offers high-accuracy predictions, reflecting the latest in chemical research and data.
Strategy Settings
Precursor scoring | Relevance Heuristic |
---|---|
Min. plausibility | 0.01 |
Model | Template_relevance |
Template Set | Pistachio/Bkms_metabolic/Pistachio_ringbreaker/Reaxys/Reaxys_biocatalysis |
Top-N result to add to graph | 6 |
Feasible Synthetic Routes
Q1: What is Salithion's primary mode of action as an insecticide?
A1: Salithion exerts its insecticidal activity by inhibiting acetylcholinesterase (AChE) []. This enzyme is crucial for nerve impulse transmission in insects.
Q2: How does the inhibition of acetylcholinesterase impact insects?
A2: Inhibiting AChE leads to the accumulation of acetylcholine at nerve synapses. This overstimulation disrupts normal nerve function, ultimately leading to paralysis and death of the insect [].
Q3: What is the molecular formula and weight of Salithion?
A3: The molecular formula of Salithion is C8H9O3PS, and its molecular weight is 216.2 g/mol [].
Q4: What is known about the structural conformation of Salithion?
A4: X-ray crystallography and NMR spectroscopy have shown that the dioxaphosphorin ring in Salithion adopts a distorted half-chair conformation, with the P=S bond oriented equatorially [].
Q5: Does the stereochemistry of Salithion affect its insecticidal activity?
A5: Yes, Salithion exhibits enantioselectivity. The (S)-enantiomer of Salithion has been found to be more potent than the (R)-enantiomer in terms of both insecticidal activity and AChE inhibition in houseflies [, , ].
Q6: How does the structure of Salithion contribute to its selective toxicity towards insects compared to mammals?
A6: While the exact mechanism is not fully understood, research suggests that the selective toxicity might be attributed to differences in the target enzyme (AChE) structure and sensitivity between insects and mammals []. Additionally, mammals might have more efficient detoxification pathways for Salithion [].
Q7: What is the relationship between Salithion's structure and its degradation in soil?
A7: Studies have demonstrated stereoselective degradation of Salithion in soil. The (S)-enantiomer degrades faster than the (R)-enantiomer, suggesting that soil microorganisms preferentially degrade the (S)-form [].
Q8: How is Salithion metabolized in living organisms?
A8: In rats, Salithion is rapidly metabolized and primarily excreted in urine. The metabolic pathways involve demethylation, oxidation to salioxon (its oxon analog), and cleavage of the P-O-aryl bond [].
Q9: Are there insects resistant to Salithion?
A9: Yes, resistance to Salithion has been observed in some insect populations, such as houseflies and bulb mites. Resistance mechanisms can involve insensitive AChE, enhanced detoxification by glutathione S-transferases (GSTs), or a combination of both [, ].
Q10: What is the role of glutathione S-transferases in Salithion resistance?
A10: GSTs catalyze the conjugation of glutathione to Salithion, leading to its detoxification and excretion. Overexpression of GSTs in resistant insects can significantly reduce Salithion's effectiveness [, ].
Q11: What is the environmental fate of Salithion?
A11: Salithion can persist in the environment, particularly in soil. Its degradation is influenced by factors like temperature, pH, and microbial activity. It can also potentially contaminate water sources [].
Q12: What are the potential ecological risks associated with Salithion use?
A12: As an insecticide, Salithion can negatively impact non-target organisms, including beneficial insects and aquatic life. Its persistence in the environment raises concerns about bioaccumulation and long-term ecological effects [].
Q13: Are there safer alternatives to Salithion for pest control?
A13: Research is ongoing to develop and evaluate safer and more environmentally friendly alternatives to Salithion. This includes exploring integrated pest management strategies, biopesticides, and insecticides with more favorable toxicological profiles [].
Q14: What analytical techniques are used to detect and quantify Salithion?
A14: Common methods for Salithion analysis include gas chromatography (GC) [], high-performance liquid chromatography (HPLC) [], and mass spectrometry (MS) [].
Q15: Have computational methods been used in Salithion research?
A15: Yes, computational chemistry tools like molecular dynamics (MD) simulations have been employed to study the interaction of Salithion enantiomers with cyclodextrins, potentially aiding in the development of novel formulations [].
Haftungsausschluss und Informationen zu In-Vitro-Forschungsprodukten
Bitte beachten Sie, dass alle Artikel und Produktinformationen, die auf BenchChem präsentiert werden, ausschließlich zu Informationszwecken bestimmt sind. Die auf BenchChem zum Kauf angebotenen Produkte sind speziell für In-vitro-Studien konzipiert, die außerhalb lebender Organismen durchgeführt werden. In-vitro-Studien, abgeleitet von dem lateinischen Begriff "in Glas", beinhalten Experimente, die in kontrollierten Laborumgebungen unter Verwendung von Zellen oder Geweben durchgeführt werden. Es ist wichtig zu beachten, dass diese Produkte nicht als Arzneimittel oder Medikamente eingestuft sind und keine Zulassung der FDA für die Vorbeugung, Behandlung oder Heilung von medizinischen Zuständen, Beschwerden oder Krankheiten erhalten haben. Wir müssen betonen, dass jede Form der körperlichen Einführung dieser Produkte in Menschen oder Tiere gesetzlich strikt untersagt ist. Es ist unerlässlich, sich an diese Richtlinien zu halten, um die Einhaltung rechtlicher und ethischer Standards in Forschung und Experiment zu gewährleisten.