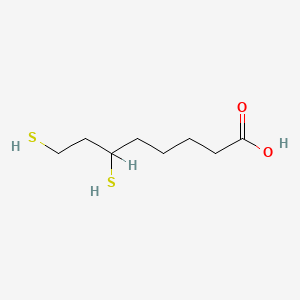
Dihydrolipoic acid
Übersicht
Beschreibung
Dihydroli-poesäure ist eine organische Verbindung, die die reduzierte Form von Li-poesäure darstellt. Diese Carbonsäure enthält ein Paar Thiolgruppen, was sie zu einem Dithiol macht. Sie ist optisch aktiv, aber nur das R-Enantiomer ist biochemisch bedeutsam. Das Li-poesäure/Dihydroli-poesäure-Paar ist an einer Vielzahl von biochemischen Transformationen beteiligt .
Herstellungsmethoden
Synthesewege und Reaktionsbedingungen
Dihydroli-poesäure kann durch Reduktionsprozesse aus Li-poesäure synthetisiert werden. Eine gängige Methode beinhaltet die Verwendung von Palladium auf Kohlenstoff (Pd/C) als Katalysator in Gegenwart von Wasserstoffgas. Die Reaktion findet typischerweise in einer Ethanol-Lösung unter kontrollierten Temperatur- und Druckbedingungen statt .
Industrielle Produktionsmethoden
Die industrielle Produktion von Dihydroli-poesäure beinhaltet häufig die Destillation von Dihydroli-poesäure oder die Extraktion von Dihydroli-poesäure aus einer protischen Lösung mit organischen Lösungsmitteln bei bestimmten pH-Werten. Diese Verfahren gewährleisten die Reinheit und Ausbeute der Verbindung .
Vorbereitungsmethoden
Synthetic Routes and Reaction Conditions
Dihydrolipoic acid can be synthesized from lipoic acid through reduction processes. One common method involves the use of palladium on carbon (Pd/C) as a catalyst in the presence of hydrogen gas. The reaction typically takes place in an ethanol solution under controlled temperature and pressure conditions .
Industrial Production Methods
Industrial production of this compound often involves the distillation of this compound or the extraction of this compound from a protic solution using organic solvents at specific pH levels. These processes ensure the purity and yield of the compound .
Analyse Chemischer Reaktionen
Reaktionstypen
Dihydroli-poesäure unterliegt verschiedenen Arten von Reaktionen, darunter:
Oxidation: Dihydroli-poesäure kann zurück zu Li-poesäure oxidiert werden.
Reduktion: Sie kann als Reduktionsmittel wirken und endogene Antioxidantien wie Vitamin C und E regenerieren.
Substitution: Die Thiolgruppen können an Substitutionsreaktionen mit verschiedenen Elektrophilen teilnehmen.
Gängige Reagenzien und Bedingungen
Oxidation: Gängige Oxidationsmittel sind Wasserstoffperoxid und molekularer Sauerstoff.
Reduktion: Wasserstoffgas in Gegenwart eines Palladiumkatalysators wird üblicherweise verwendet.
Substitution: Elektrophile wie Alkylhalogenide können unter milden Bedingungen mit den Thiolgruppen reagieren.
Hauptprodukte
Oxidation: Li-poesäure ist das Hauptprodukt.
Reduktion: Regenerierte Antioxidantien wie Vitamin C und E.
Substitution: Verschiedene substituierte Thiol-Derivate, abhängig von dem verwendeten Elektrophil.
Wissenschaftliche Forschungsanwendungen
Dihydroli-poesäure hat eine breite Palette von wissenschaftlichen Forschungsanwendungen:
Chemie: Sie wird als Reduktionsmittel und bei der Synthese verschiedener Thiol-haltiger Verbindungen verwendet.
Biologie: Sie spielt eine Rolle im Zellstoffwechsel und wirkt als Coenzym in mehreren enzymatischen Reaktionen.
Wirkmechanismus
Dihydroli-poesäure übt ihre Wirkungen hauptsächlich durch ihre antioxidativen Eigenschaften aus. Sie kann reaktive Sauerstoffspezies abfangen und andere Antioxidantien regenerieren. Die Verbindung interagiert mit verschiedenen molekularen Zielstrukturen, darunter Enzyme, die am mitochondrialen Energiestoffwechsel beteiligt sind, wie z. B. der Pyruvat-Dehydrogenase-Komplex . Sie moduliert auch Proteinspiegel und fördert die Aktivität von Neurotransmittern und Antioxidantien .
Wissenschaftliche Forschungsanwendungen
Antioxidant Properties
Mechanism of Action:
Dihydrolipoic acid acts as a strong reductant, effectively regenerating oxidized antioxidants and reducing oxidative stress in biological systems. It has been shown to decrease levels of reactive oxygen species (ROS) and inhibit inflammatory pathways, making it a valuable compound in the treatment of diseases associated with oxidative stress.
Case Study: Neuroinflammation and Depression
A study demonstrated that DHLA could mitigate sickness behavior induced by lipopolysaccharides (LPS) in rats. The treatment with DHLA increased the expression of key proteins involved in antioxidant defense (Nrf2 and HO-1) while decreasing pro-inflammatory markers such as NLRP3 and IL-1β. This suggests DHLA's potential as a therapeutic strategy for conditions like depression that are linked to neuroinflammation .
Cancer Chemoprevention
Inhibition of Tumor Promotion:
Research indicates that DHLA may serve as a chemopreventive agent against inflammation-associated tumorigenesis. In a study involving skin tumor models, DHLA significantly reduced tumor incidence and multiplicity induced by chemical carcinogens. It achieved this by inhibiting nitric oxide production and cyclooxygenase-2 activity, both critical mediators in cancer promotion .
Table 1: Summary of DHLA's Effects on Cancer Models
Metabolic Disorders
Diabetes Management:
this compound has been studied for its role in managing diabetes-related complications. Its antioxidant properties help reduce oxidative damage associated with diabetic neuropathy. In clinical trials, DHLA supplementation has shown promise in improving nerve conduction velocity and reducing symptoms of diabetic polyneuropathy .
Cardiovascular Health
Protection Against Oxidative Stress:
DHLA's ability to reduce oxidative stress extends to cardiovascular applications as well. It has been shown to protect against oxidative damage induced by aluminum exposure, suggesting potential benefits in preventing cardiovascular diseases linked to oxidative stress .
Summary of Applications
This compound is emerging as a versatile compound with significant potential across various domains:
- Antioxidant Therapy: Reduces oxidative stress and inflammation.
- Cancer Prevention: Inhibits tumor promotion through anti-inflammatory mechanisms.
- Neuroprotection: Offers therapeutic potential in neurodegenerative diseases and depression.
- Diabetes Management: Alleviates symptoms of diabetic neuropathy.
- Cardiovascular Protection: Shields against oxidative damage.
Wirkmechanismus
Dihydrolipoic acid exerts its effects primarily through its antioxidant properties. It can scavenge reactive oxygen species and regenerate other antioxidants. The compound interacts with various molecular targets, including enzymes involved in the mitochondrial energy metabolism, such as the pyruvate dehydrogenase complex . It also modulates protein levels and promotes the activity of neurotransmitters and antioxidants .
Vergleich Mit ähnlichen Verbindungen
Ähnliche Verbindungen
Li-poesäure: Die oxidierte Form von Dihydroli-poesäure, ebenfalls bekannt für ihre antioxidativen Eigenschaften.
Glutathion: Ein weiteres Thiol-haltiges Antioxidans, das eine entscheidende Rolle bei der zellulären Abwehr gegen oxidativen Stress spielt.
Cystein: Eine Aminosäure mit einer Thiolgruppe, die an der Synthese von Proteinen und anderen Biomolekülen beteiligt ist.
Einzigartigkeit
Dihydroli-poesäure ist einzigartig aufgrund ihrer Fähigkeit, endogene Antioxidantien zu regenerieren, und ihrer Doppelfunktion als sowohl Antioxidans als auch Coenzym. Im Gegensatz zu Glutathion und Cystein kann Dihydroli-poesäure leicht die Blut-Hirn-Schranke überwinden, was sie besonders wertvoll in neuroprotektiven Anwendungen macht .
Biologische Aktivität
Dihydrolipoic acid (DHLA), the reduced form of alpha-lipoic acid (LA), is recognized for its significant biological activities, particularly its antioxidant and anti-inflammatory properties. This article delves into the biological activity of DHLA, supported by various studies and findings.
Antioxidant Properties
DHLA is a potent antioxidant that plays a crucial role in reducing oxidative stress. It can regenerate other antioxidants such as vitamins C and E and increase intracellular glutathione levels, which are vital for cellular defense against oxidative damage . The compound acts by scavenging free radicals and chelating metal ions, thus preventing oxidative damage to lipids, proteins, and DNA .
Table 1: Antioxidant Mechanisms of DHLA
Mechanism | Description |
---|---|
Free Radical Scavenging | Directly neutralizes reactive oxygen species (ROS) |
Metal Ion Chelation | Binds to metal ions, reducing their availability to catalyze oxidative reactions |
Regeneration of Antioxidants | Restores oxidized forms of vitamins C and E |
Glutathione Synthesis | Enhances the production of glutathione, a key cellular antioxidant |
Anti-inflammatory Effects
Research indicates that DHLA exhibits significant anti-inflammatory effects. In a study involving lipopolysaccharide (LPS)-induced sickness behavior in rats, DHLA treatment resulted in decreased levels of inflammatory markers such as NLRP3, caspase-1, and IL-1β. This suggests that DHLA may modulate inflammatory pathways through the ERK/Nrf2/HO-1 signaling pathway .
Case Study: LPS-Induced Sickness Behavior
- Objective : To evaluate the preventive effects of DHLA on LPS-induced sickness behavior.
- Method : Rats were treated with DHLA prior to LPS administration.
- Results :
- Increased expression of ERK, Nrf2, and HO-1.
- Decreased ROS generation.
- Reduced expression of pro-inflammatory cytokines.
This study highlights DHLA's potential as a therapeutic agent for conditions characterized by neuroinflammation and depression .
Chemopreventive Potential
DHLA has been investigated for its chemopreventive properties in cancer. A study demonstrated that it inhibited skin tumor promotion in mice by reducing nitric oxide (NO) and prostaglandin E2 (PGE2) production, both of which are associated with inflammation and tumorigenesis. The compound effectively suppressed inducible nitric oxide synthase (iNOS) expression while not significantly affecting cyclooxygenase-2 (COX-2) levels .
Table 2: Effects of DHLA on Tumor Promotion
Parameter | Control Group | DHLA Treatment |
---|---|---|
Tumor Incidence | High | Low |
Tumor Multiplicity | High | Low |
iNOS Expression | High | Significantly Reduced |
COX-2 Activity | Unchanged | Inhibited |
Pro-oxidant Activity
Interestingly, while DHLA is primarily known for its antioxidant properties, it can also exhibit pro-oxidant effects under certain conditions. In vitro studies have shown that DHLA can enhance the toxicity of certain compounds like 4-aminophenol by increasing methaemoglobin formation without depleting thiol levels . This duality in function highlights the importance of context in evaluating the biological activity of DHLA.
Eigenschaften
IUPAC Name |
6,8-bis(sulfanyl)octanoic acid | |
---|---|---|
Source | PubChem | |
URL | https://pubchem.ncbi.nlm.nih.gov | |
Description | Data deposited in or computed by PubChem | |
InChI |
InChI=1S/C8H16O2S2/c9-8(10)4-2-1-3-7(12)5-6-11/h7,11-12H,1-6H2,(H,9,10) | |
Source | PubChem | |
URL | https://pubchem.ncbi.nlm.nih.gov | |
Description | Data deposited in or computed by PubChem | |
InChI Key |
IZFHEQBZOYJLPK-UHFFFAOYSA-N | |
Source | PubChem | |
URL | https://pubchem.ncbi.nlm.nih.gov | |
Description | Data deposited in or computed by PubChem | |
Canonical SMILES |
C(CCC(=O)O)CC(CCS)S | |
Source | PubChem | |
URL | https://pubchem.ncbi.nlm.nih.gov | |
Description | Data deposited in or computed by PubChem | |
Molecular Formula |
C8H16O2S2 | |
Source | PubChem | |
URL | https://pubchem.ncbi.nlm.nih.gov | |
Description | Data deposited in or computed by PubChem | |
DSSTOX Substance ID |
DTXSID50861946 | |
Record name | 6,8-Dihydrothioctic acid | |
Source | EPA DSSTox | |
URL | https://comptox.epa.gov/dashboard/DTXSID50861946 | |
Description | DSSTox provides a high quality public chemistry resource for supporting improved predictive toxicology. | |
Molecular Weight |
208.3 g/mol | |
Source | PubChem | |
URL | https://pubchem.ncbi.nlm.nih.gov | |
Description | Data deposited in or computed by PubChem | |
Physical Description |
Solid | |
Record name | Dihydrolipoate | |
Source | Human Metabolome Database (HMDB) | |
URL | http://www.hmdb.ca/metabolites/HMDB0012210 | |
Description | The Human Metabolome Database (HMDB) is a freely available electronic database containing detailed information about small molecule metabolites found in the human body. | |
Explanation | HMDB is offered to the public as a freely available resource. Use and re-distribution of the data, in whole or in part, for commercial purposes requires explicit permission of the authors and explicit acknowledgment of the source material (HMDB) and the original publication (see the HMDB citing page). We ask that users who download significant portions of the database cite the HMDB paper in any resulting publications. | |
CAS No. |
462-20-4 | |
Record name | Dihydrolipoic acid | |
Source | CAS Common Chemistry | |
URL | https://commonchemistry.cas.org/detail?cas_rn=462-20-4 | |
Description | CAS Common Chemistry is an open community resource for accessing chemical information. Nearly 500,000 chemical substances from CAS REGISTRY cover areas of community interest, including common and frequently regulated chemicals, and those relevant to high school and undergraduate chemistry classes. This chemical information, curated by our expert scientists, is provided in alignment with our mission as a division of the American Chemical Society. | |
Explanation | The data from CAS Common Chemistry is provided under a CC-BY-NC 4.0 license, unless otherwise stated. | |
Record name | Dihydrolipoic acid | |
Source | ChemIDplus | |
URL | https://pubchem.ncbi.nlm.nih.gov/substance/?source=chemidplus&sourceid=0000462204 | |
Description | ChemIDplus is a free, web search system that provides access to the structure and nomenclature authority files used for the identification of chemical substances cited in National Library of Medicine (NLM) databases, including the TOXNET system. | |
Record name | 6,8-Dihydrothioctic acid | |
Source | EPA DSSTox | |
URL | https://comptox.epa.gov/dashboard/DTXSID50861946 | |
Description | DSSTox provides a high quality public chemistry resource for supporting improved predictive toxicology. | |
Record name | 6,8-disulfanyloctanoic acid | |
Source | European Chemicals Agency (ECHA) | |
URL | https://echa.europa.eu/substance-information/-/substanceinfo/100.120.390 | |
Description | The European Chemicals Agency (ECHA) is an agency of the European Union which is the driving force among regulatory authorities in implementing the EU's groundbreaking chemicals legislation for the benefit of human health and the environment as well as for innovation and competitiveness. | |
Explanation | Use of the information, documents and data from the ECHA website is subject to the terms and conditions of this Legal Notice, and subject to other binding limitations provided for under applicable law, the information, documents and data made available on the ECHA website may be reproduced, distributed and/or used, totally or in part, for non-commercial purposes provided that ECHA is acknowledged as the source: "Source: European Chemicals Agency, http://echa.europa.eu/". Such acknowledgement must be included in each copy of the material. ECHA permits and encourages organisations and individuals to create links to the ECHA website under the following cumulative conditions: Links can only be made to webpages that provide a link to the Legal Notice page. | |
Record name | DIHYDROTHIOCTIC ACID | |
Source | FDA Global Substance Registration System (GSRS) | |
URL | https://gsrs.ncats.nih.gov/ginas/app/beta/substances/7NV2KHU5JA | |
Description | The FDA Global Substance Registration System (GSRS) enables the efficient and accurate exchange of information on what substances are in regulated products. Instead of relying on names, which vary across regulatory domains, countries, and regions, the GSRS knowledge base makes it possible for substances to be defined by standardized, scientific descriptions. | |
Explanation | Unless otherwise noted, the contents of the FDA website (www.fda.gov), both text and graphics, are not copyrighted. They are in the public domain and may be republished, reprinted and otherwise used freely by anyone without the need to obtain permission from FDA. Credit to the U.S. Food and Drug Administration as the source is appreciated but not required. | |
Record name | Dihydrolipoate | |
Source | Human Metabolome Database (HMDB) | |
URL | http://www.hmdb.ca/metabolites/HMDB0012210 | |
Description | The Human Metabolome Database (HMDB) is a freely available electronic database containing detailed information about small molecule metabolites found in the human body. | |
Explanation | HMDB is offered to the public as a freely available resource. Use and re-distribution of the data, in whole or in part, for commercial purposes requires explicit permission of the authors and explicit acknowledgment of the source material (HMDB) and the original publication (see the HMDB citing page). We ask that users who download significant portions of the database cite the HMDB paper in any resulting publications. | |
Synthesis routes and methods I
Procedure details
Synthesis routes and methods II
Procedure details
Retrosynthesis Analysis
AI-Powered Synthesis Planning: Our tool employs the Template_relevance Pistachio, Template_relevance Bkms_metabolic, Template_relevance Pistachio_ringbreaker, Template_relevance Reaxys, Template_relevance Reaxys_biocatalysis model, leveraging a vast database of chemical reactions to predict feasible synthetic routes.
One-Step Synthesis Focus: Specifically designed for one-step synthesis, it provides concise and direct routes for your target compounds, streamlining the synthesis process.
Accurate Predictions: Utilizing the extensive PISTACHIO, BKMS_METABOLIC, PISTACHIO_RINGBREAKER, REAXYS, REAXYS_BIOCATALYSIS database, our tool offers high-accuracy predictions, reflecting the latest in chemical research and data.
Strategy Settings
Precursor scoring | Relevance Heuristic |
---|---|
Min. plausibility | 0.01 |
Model | Template_relevance |
Template Set | Pistachio/Bkms_metabolic/Pistachio_ringbreaker/Reaxys/Reaxys_biocatalysis |
Top-N result to add to graph | 6 |
Feasible Synthetic Routes
Q1: How does dihydrolipoic acid (DHLA) exert its antioxidant effects?
A1: DHLA, the reduced form of α-lipoic acid, acts as a potent antioxidant through several mechanisms. It directly scavenges free radicals like hydroxyl radicals [] and reduces the phenoxyl radical of etoposide []. DHLA also participates in a redox cycle with ascorbate, regenerating it from its oxidized form, dehydroascorbate [, , ]. This recycling of ascorbate contributes to maintaining a robust antioxidant network in cells [].
Q2: Does DHLA have any pro-oxidant activities?
A2: While primarily known for its antioxidant properties, DHLA can exhibit pro-oxidant behavior under specific conditions. For example, it can stimulate superoxide anion production in rat liver mitochondria []. Additionally, the oxidation of DHLA can generate a disulfide radical anion, which reacts with oxygen to form superoxide, a reactive oxygen species with pro-oxidant potential [].
Q3: How does DHLA interact with glutathione in the cellular antioxidant system?
A3: DHLA can indirectly support the regeneration of vitamin E by reducing oxidized glutathione (GSSG) to its reduced form, glutathione (GSH) []. This interplay between DHLA, glutathione, and vitamin E highlights the interconnected nature of the cellular antioxidant network []. Additionally, DHLA can increase intracellular glutathione levels by improving cystine utilization, bypassing the need for the cystine/glutamate antiporter [].
Q4: What is the role of DHLA in the redox regulation of mitochondrial aldehyde dehydrogenase (ALDH-2)?
A4: DHLA can restore the activity of ALDH-2, a mitochondrial enzyme involved in detoxification pathways, after its inactivation by reactive oxygen species like superoxide and peroxynitrite []. This restorative effect is attributed to DHLA's ability to reduce a disulfide bond at the enzyme's active site.
Q5: What is the molecular formula and weight of DHLA?
A5: DHLA has the molecular formula C8H16O2S2 and a molecular weight of 208.33 g/mol.
Q6: What spectroscopic techniques are used to characterize DHLA?
A6: Several spectroscopic techniques are employed to characterize DHLA, including:
- NMR Spectroscopy: Used to determine the structure and study the chemical environment of DHLA and its derivatives [].
- UV-Vis Spectroscopy: DHLA absorbs UV light around 330 nm due to its strained five-membered ring. This property is useful for quantifying its concentration [].
- Electron Spin Resonance (ESR) Spectroscopy: Used to detect and characterize the radical species generated during the oxidation of DHLA [].
- X-ray Absorption Near Edge Structure (XANES) Spectroscopy: Can provide information about the binding mode of DHLA to nanoparticles [].
Q7: What alternative ligands can be used to improve the properties of DHLA-capped QDs?
A8: Compact zwitterionic ligands based on DHLA have been explored to create smaller QDs with improved colloidal stability and reduced nonspecific interactions in biological environments compared to DHLA-PEG coated QDs [].
Q8: How stable is DHLA, and what strategies can enhance its stability and bioavailability?
A9: DHLA can undergo oxidation to form α-lipoic acid. This interconversion is influenced by factors like pH and the presence of oxidizing agents. While specific formulation strategies to enhance DHLA's stability are limited in the provided literature, research suggests that administering α-lipoic acid could indirectly increase DHLA levels in vivo due to its intracellular reduction to DHLA [, ].
Q9: What is the role of DHLA in protecting against lipid peroxidation?
A10: DHLA can protect against lipid peroxidation, a damaging process affecting cell membranes, by directly scavenging peroxyl radicals and indirectly by supporting the recycling of other antioxidants like vitamin E and glutathione [, ].
Q10: How does DHLA interact with enzymes containing dithiol groups?
A11: DHLA, with its two thiol groups, can interact with and modulate the activity of enzymes containing dithiol groups. For instance, DHLA can inhibit elastase activity in cystic fibrosis sputum, potentially by disrupting disulfide bonds within the enzyme [].
Q11: What are the effects of DHLA on cellular signaling pathways?
A12: DHLA can influence cellular signaling pathways, particularly those related to oxidative stress. For example, it has been shown to suppress the expression of the proto-oncogene c-fos, which is involved in the cellular response to growth factors and oxidative stress [, ].
Q12: Can DHLA be used to repair oxidative damage to proteins?
A13: Yes, DHLA can act as a cofactor for the enzyme peptide methionine sulfoxide reductase (PMSR) [, ]. This enzyme catalyzes the reduction of methionine sulfoxide, a common oxidative modification in proteins, back to methionine, thereby repairing oxidative damage.
Q13: Are there any safety concerns regarding the use of DHLA?
A14: While generally considered safe, DHLA can exert cytotoxic effects at higher concentrations. Studies on mouse embryos showed that DHLA concentrations above 50 μM induced apoptosis and negatively impacted embryonic development []. This finding underscores the importance of carefully considering dosage and potential risks associated with DHLA exposure.
Haftungsausschluss und Informationen zu In-Vitro-Forschungsprodukten
Bitte beachten Sie, dass alle Artikel und Produktinformationen, die auf BenchChem präsentiert werden, ausschließlich zu Informationszwecken bestimmt sind. Die auf BenchChem zum Kauf angebotenen Produkte sind speziell für In-vitro-Studien konzipiert, die außerhalb lebender Organismen durchgeführt werden. In-vitro-Studien, abgeleitet von dem lateinischen Begriff "in Glas", beinhalten Experimente, die in kontrollierten Laborumgebungen unter Verwendung von Zellen oder Geweben durchgeführt werden. Es ist wichtig zu beachten, dass diese Produkte nicht als Arzneimittel oder Medikamente eingestuft sind und keine Zulassung der FDA für die Vorbeugung, Behandlung oder Heilung von medizinischen Zuständen, Beschwerden oder Krankheiten erhalten haben. Wir müssen betonen, dass jede Form der körperlichen Einführung dieser Produkte in Menschen oder Tiere gesetzlich strikt untersagt ist. Es ist unerlässlich, sich an diese Richtlinien zu halten, um die Einhaltung rechtlicher und ethischer Standards in Forschung und Experiment zu gewährleisten.