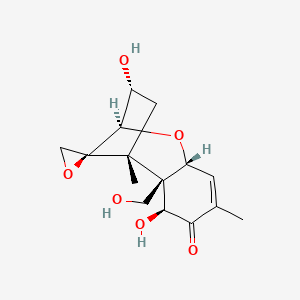
Deoxynivalenol
Übersicht
Beschreibung
Deoxynivalenol is a trichothecene mycotoxin produced by Fusarium to which wheat, barley, maize (corn) and their products are susceptible to contamination. It has a role as a mycotoxin. It is a trichothecene, a cyclic ketone, a secondary alpha-hydroxy ketone, a primary alcohol, an enone and a triol.
This compound is a natural product found in Fusarium graminearum, Fusarium culmorum, and Euglena gracilis with data available.
Wirkmechanismus
Target of Action
Deoxynivalenol (DON) primarily targets the ribosome , binding to its 60S subunit . This interaction disrupts protein synthesis, leading to a cascade of cellular effects .
Mode of Action
Upon binding to the ribosome, DON induces ribotoxic stress , disrupting macromolecule synthesis, cell signaling, differentiation, proliferation, and death . It also activates mitogen-activated protein kinases (MAPK) through a process known as the ribotoxic stress response (RSR) .
Biochemical Pathways
The biochemical pathways affected by DON are diverse. It alters neuroendocrine signaling, induces proinflammatory gene expression, disrupts the growth hormone axis, and compromises gut integrity . Additionally, DON has been reported to inhibit the TNF-α-induced NF-κB signaling pathway .
Pharmacokinetics
While specific ADME (Absorption, Distribution, Metabolism, Excretion) properties for DON in humans are not fully known, extrapolation from animal models suggests that DON has a plasma clearance of 0.24 L/(h×kg) and a volume of distribution of 1.24 L/kg . These parameters suggest that DON may have a bioavailability ranging from 50% to 90% .
Result of Action
The effects of DON are dose-dependent and can be both acute and chronic. Acute exposure can cause symptoms like abdominal pain, anorexia, diarrhea, increased salivation, vomiting, and malaise. Chronic exposure can lead to changes in dietary efficacy, weight loss, and anorexia . At the cellular level, DON can cause apoptosis, cell cycle arrest, and immunosuppression .
Action Environment
The production and action of DON are influenced by environmental factors. For instance, humidity and intensive rainfall during and after anthesis result in increased DON production . Furthermore, DON is stable at cooking temperatures and in animal feed processing, which can influence its action, efficacy, and stability .
Wissenschaftliche Forschungsanwendungen
Toxikologische Forschung
DON ist ein toxisches sekundäres Metabolit, das von Pilzen produziert wird und viele Feldfrüchte, vor allem Weizen, Mais und Gerste, kontaminiert . Es wirkt sich auf die Gesundheit von Tieren aus und verursacht bei niedrigen Dosen Darmbarrierschäden und immunstimulierende Effekte sowie Erbrechen, eine Verringerung der Futterverwertung und Immunsuppression bei hohen Dosen . Dies macht DON zu einem wichtigen Forschungsthema in der toxikologischen Forschung.
Dekontamination von Lebensmitteln und Futtermitteln
Die Kontamination von Lebensmitteln und Futtermitteln mit DON ist ein globales Problem. Es verursacht nicht nur erhebliche wirtschaftliche Verluste, sondern kann auch zu Durchfall, Erbrechen und Gastroenteritis bei Menschen und Nutztieren führen . Daher ist die Entwicklung effizienter Verfahren zur Dekontamination von Futtermitteln und Lebensmitteln mit DON ein entscheidender Forschungsbereich .
Biologische Entgiftung
Biologische Entgiftungsmethoden, die auf mikrobiellen Stämmen oder Enzymen basieren, haben den Vorteil einer hohen Spezifität, Effizienz und keiner Sekundärverschmutzung . Das Verständnis der spezifischen Mechanismen, durch die DON entgiftet wird, wird eine effiziente, sichere und wirtschaftliche Möglichkeit zur Entfernung von Toxinen aus Lebensmitteln und Futtermitteln bieten .
Phylogenetische Analyse
Das Verständnis der phylogenetischen Muster von DON-Abbauern ist ein wichtiger Schritt, um Hinweise zur Optimierung des Screenings nach neuen Organismen mit diesem Potenzial zu liefern . Dieser Forschungsbereich kann einen aktuellen Überblick über die negativen Auswirkungen von DON auf die Tierproduktion liefern .
Aktivität von antioxidativen Enzymen
Um die nachteiligen Auswirkungen von DON auf das Pflanzenwachstum zu verringern, wird die Aktivität von antioxidativen Enzymen in der Regel im Cytoplasma der Wirtspflanzen erhöht . Diese Enzyme ermöglichen die Beseitigung von extrazellulären und zellulären ROS , was dies zu einer einzigartigen Anwendung von DON in der wissenschaftlichen Forschung macht.
Risikobewertung und Regulierung
Das Vorhandensein von DON in landwirtschaftlichen Nutzpflanzen, Futtermitteln und Getreideprodukten erfordert eine wissenschaftliche Grundlage für die Risikobewertung und -regulierung . Dieser Forschungsbereich zielt darauf ab, eine wissenschaftliche Grundlage für die Notwendigkeit zu liefern, die Risikobewertung und -regulierung zu überdenken .
Biochemische Analyse
Biochemical Properties
Deoxynivalenol plays a significant role in biochemical reactions. It is a type-B trichothecene that Fusarium graminearum and Fusarium culmorum typically produce . At the molecular level, this compound disrupts normal cell function by inhibiting protein synthesis via binding to the ribosome and by activating critical cellular kinases involved in signal transduction related to proliferation, differentiation, and apoptosis .
Cellular Effects
This compound has profound effects on various types of cells and cellular processes. It influences cell function by inducing ribotoxic stress, thereby disrupting macromolecule synthesis, cell signaling, differentiation, proliferation, and death . Pathophysiologic effects associated with this compound include altered neuroendocrine signaling, proinflammatory gene induction, disruption of the growth hormone axis, and altered gut integrity .
Molecular Mechanism
This compound exerts its effects at the molecular level through several mechanisms. It binds to the ribosome, induces a ribotoxic stress leading to the activation of MAP kinases, cellular cell-cycle arrest and apoptosis . This disruption of macromolecule synthesis, cell signaling, differentiation, proliferation, and death is a key aspect of this compound’s mechanism of action .
Temporal Effects in Laboratory Settings
In laboratory settings, the effects of this compound can change over time. It is stable at high temperatures and low pH, and wheat contaminated with this compound is still toxic after 4 years of storage .
Dosage Effects in Animal Models
The effects of this compound vary with different dosages in animal models. In experimental animal models, acute this compound poisoning causes emesis, whereas chronic low-dose exposure elicits anorexia, growth retardation, immunotoxicity as well as impaired reproduction and development resulting from maternal toxicity .
Metabolic Pathways
This compound is involved in several metabolic pathways. It is rapidly absorbed following ingestion and is mainly metabolized in the liver through glucuronidation and excreted in urine .
Transport and Distribution
This compound is transported and distributed within cells and tissues. It is first enriched in the plasma, liver and kidney and subsequently accumulates in the intestine .
Subcellular Localization
It is known that this compound and its biosynthetic enzymes are compartmentalized at conserved subcellular sites that position pathway enzymes to use common biochemical precursors .
Eigenschaften
IUPAC Name |
(1R,2R,3S,7R,9R,10R,12S)-3,10-dihydroxy-2-(hydroxymethyl)-1,5-dimethylspiro[8-oxatricyclo[7.2.1.02,7]dodec-5-ene-12,2'-oxirane]-4-one | |
---|---|---|
Source | PubChem | |
URL | https://pubchem.ncbi.nlm.nih.gov | |
Description | Data deposited in or computed by PubChem | |
InChI |
InChI=1S/C15H20O6/c1-7-3-9-14(5-16,11(19)10(7)18)13(2)4-8(17)12(21-9)15(13)6-20-15/h3,8-9,11-12,16-17,19H,4-6H2,1-2H3/t8-,9-,11-,12-,13-,14-,15+/m1/s1 | |
Source | PubChem | |
URL | https://pubchem.ncbi.nlm.nih.gov | |
Description | Data deposited in or computed by PubChem | |
InChI Key |
LINOMUASTDIRTM-QGRHZQQGSA-N | |
Source | PubChem | |
URL | https://pubchem.ncbi.nlm.nih.gov | |
Description | Data deposited in or computed by PubChem | |
Canonical SMILES |
CC1=CC2C(C(C1=O)O)(C3(CC(C(C34CO4)O2)O)C)CO | |
Source | PubChem | |
URL | https://pubchem.ncbi.nlm.nih.gov | |
Description | Data deposited in or computed by PubChem | |
Isomeric SMILES |
CC1=C[C@@H]2[C@]([C@@H](C1=O)O)([C@]3(C[C@H]([C@H]([C@@]34CO4)O2)O)C)CO | |
Source | PubChem | |
URL | https://pubchem.ncbi.nlm.nih.gov | |
Description | Data deposited in or computed by PubChem | |
Molecular Formula |
C15H20O6 | |
Source | PubChem | |
URL | https://pubchem.ncbi.nlm.nih.gov | |
Description | Data deposited in or computed by PubChem | |
DSSTOX Substance ID |
DTXSID3020382 | |
Record name | Vomitoxin | |
Source | EPA DSSTox | |
URL | https://comptox.epa.gov/dashboard/DTXSID3020382 | |
Description | DSSTox provides a high quality public chemistry resource for supporting improved predictive toxicology. | |
Molecular Weight |
296.31 g/mol | |
Source | PubChem | |
URL | https://pubchem.ncbi.nlm.nih.gov | |
Description | Data deposited in or computed by PubChem | |
Physical Description |
Solid; [Merck Index] | |
Record name | Deoxynivalenol | |
Source | Haz-Map, Information on Hazardous Chemicals and Occupational Diseases | |
URL | https://haz-map.com/Agents/4683 | |
Description | Haz-Map® is an occupational health database designed for health and safety professionals and for consumers seeking information about the adverse effects of workplace exposures to chemical and biological agents. | |
Explanation | Copyright (c) 2022 Haz-Map(R). All rights reserved. Unless otherwise indicated, all materials from Haz-Map are copyrighted by Haz-Map(R). No part of these materials, either text or image may be used for any purpose other than for personal use. Therefore, reproduction, modification, storage in a retrieval system or retransmission, in any form or by any means, electronic, mechanical or otherwise, for reasons other than personal use, is strictly prohibited without prior written permission. | |
Solubility |
Soluble to 30 nM in ethanol, Soluble in polar organic solvents (e.g., aqueous methanol, ethanol, chloroform, acetonitrile, and ethyl acetate) and water[NTP; Chemical Information Review Document for Deoxynivalenol | |
Record name | DEOXYNIVALENOL | |
Source | Hazardous Substances Data Bank (HSDB) | |
URL | https://pubchem.ncbi.nlm.nih.gov/source/hsdb/7245 | |
Description | The Hazardous Substances Data Bank (HSDB) is a toxicology database that focuses on the toxicology of potentially hazardous chemicals. It provides information on human exposure, industrial hygiene, emergency handling procedures, environmental fate, regulatory requirements, nanomaterials, and related areas. The information in HSDB has been assessed by a Scientific Review Panel. | |
Mechanism of Action |
The objective of this work was to investigate whether proteomic analysis of thymoma cells treated with the trichothecene deoxynivalenol (DON) as compared to non-treated (control) cells would reveal differential protein expression, and thus would contribute to a better understanding of the mechanisms of its toxicity. For that purpose the mouse thymoma cell line EL4 was exposed to 0.5 uM DON for 6 hr. A total of 30 proteins were affected after exposure of EL4 cells to DON. Most of these proteins were up-regulated and included key metabolic enzymes (e.g., fatty acid synthase, aldose reductase, carbamoyl phosphate synthetase, glucose-6-phosphate isomerase), chaperones (e.g., HSP9AB1 and HSP70), enzymes implicated in protein folding (PDI and ERO1-l alpha), and proteins involved in protein degradation (ubiquitin-conjugating enzyme (E1) and proteasome subunit alpha type-1). In addition, an IgE-binding protein with a molecular weight of 60 kDa and My-binding protein 1a (MYBBP1A), a transcription factor, were found to be up-regulated by DON. The observed up-regulation of MYBBP1A, a known repressor of a number of transcription factors such as PGC-1 alpha, C-myb, and p65 of the NF-kappaB family, suggests that this protein might play a role in the mechanism of DON toxicity., Deoxynivalenol (DON), one of the most abundant trichothecenes found on cereals, has been implicated in mycotoxicoses in both humans and farm animals. Low-dose toxicity is characterized by reduced weight gain, diminished nutritional efficiency, and immunologic effects. The levels and patterns of human food commodity contamination justify that DON consumption constitutes a public health issue. DON stability during processing and cooking explains its large presence in human food. /The authors/ characterized here DON intoxication by showing that the toxin concomitantly affects feeding behavior, body temperature, and locomotor activity after both per os and central administration. Using c-Fos expression mapping, /the authors/ identified the neuronal structures activated in response to DON and observed that the pattern of neuronal populations activated by the toxin resembled those induced by inflammatory signals. By real-time PCR, /the authors/ report the ...evidences for a DON-induced central inflammation, attested by the strong upregulation of interleukin-1beta, interleukin-6, tumor necrosis factor-alpha, cyclooxygenase-2, and microsomal prostaglandin synthase-1 (mPGES-1) messenger RNA. However, silencing prostaglandins E2 signaling pathways using mPGES-1 knockout mice, which are resistant to cytokine-induced sickness behavior, did not modify the responses to the toxin. These results reveal that, despite strong similarities, behavioral changes observed after DON intoxication differ from classical sickness behavior evoked by inflammatory cytokines., Trichothecenes are toxic for actively dividing cells, such as the intestinal crypt epithelium and the hematopoietic cells. The cytotoxicity has been associated with either impairment of protein synthesis by the binding of the compounds to the ribosomes of eukaryotic cells, or the dysfunction of cellular membranes. Inhibition of protein synthesis has been associated with the induction of labile and regulatory proteins, such as IL-2 in immunocytes. Transport of small molecules is impaired in cell membranes by extremely low concentrations of trichothecenes. /Trichothecenes/, Most trichothecenes inhibit protein synthesis, their potency depending on structural substituents and requiring an unsaturated bond at the C9-C10 position and integrity of the 12,13-epoxy ring. Trichothecenes bind to the 60S subunit of eukaryotic ribosomes and interfere with the activity of peptidyltransferase. Deoxynivalenol, which lacks a substituent at C-4, inhibits chain elongation. Inhibition of protein synthesis is considered to be the primary toxic effect of trichothecenes, including deoxynivalenol. The ID50 for inhibition of protein synthesis in rabbit reticulocytes was 2 ug/mL... . In vitro, deoxynivalenol is about 100 times less toxic than T-2 toxin, which has been more widely studied for its macromolecular effects. Owing to differences in lipophilicity and other possible effects, the toxicity of deoxynivalenol in vivo is greater than would be expected from its effects on protein synthesis in vitro., For more Mechanism of Action (Complete) data for DEOXYNIVALENOL (10 total), please visit the HSDB record page. | |
Record name | DEOXYNIVALENOL | |
Source | Hazardous Substances Data Bank (HSDB) | |
URL | https://pubchem.ncbi.nlm.nih.gov/source/hsdb/7245 | |
Description | The Hazardous Substances Data Bank (HSDB) is a toxicology database that focuses on the toxicology of potentially hazardous chemicals. It provides information on human exposure, industrial hygiene, emergency handling procedures, environmental fate, regulatory requirements, nanomaterials, and related areas. The information in HSDB has been assessed by a Scientific Review Panel. | |
Color/Form |
Fine needles from ethyl acetate + petroleum ether, Crystals from methanol (aqueous) | |
CAS No. |
51481-10-8 | |
Record name | Deoxynivalenol | |
Source | CAS Common Chemistry | |
URL | https://commonchemistry.cas.org/detail?cas_rn=51481-10-8 | |
Description | CAS Common Chemistry is an open community resource for accessing chemical information. Nearly 500,000 chemical substances from CAS REGISTRY cover areas of community interest, including common and frequently regulated chemicals, and those relevant to high school and undergraduate chemistry classes. This chemical information, curated by our expert scientists, is provided in alignment with our mission as a division of the American Chemical Society. | |
Explanation | The data from CAS Common Chemistry is provided under a CC-BY-NC 4.0 license, unless otherwise stated. | |
Record name | Deoxynivalenol | |
Source | ChemIDplus | |
URL | https://pubchem.ncbi.nlm.nih.gov/substance/?source=chemidplus&sourceid=0051481108 | |
Description | ChemIDplus is a free, web search system that provides access to the structure and nomenclature authority files used for the identification of chemical substances cited in National Library of Medicine (NLM) databases, including the TOXNET system. | |
Record name | Vomitoxin | |
Source | EPA DSSTox | |
URL | https://comptox.epa.gov/dashboard/DTXSID3020382 | |
Description | DSSTox provides a high quality public chemistry resource for supporting improved predictive toxicology. | |
Record name | DEOXYNIVALENOL | |
Source | FDA Global Substance Registration System (GSRS) | |
URL | https://gsrs.ncats.nih.gov/ginas/app/beta/substances/JT37HYP23V | |
Description | The FDA Global Substance Registration System (GSRS) enables the efficient and accurate exchange of information on what substances are in regulated products. Instead of relying on names, which vary across regulatory domains, countries, and regions, the GSRS knowledge base makes it possible for substances to be defined by standardized, scientific descriptions. | |
Explanation | Unless otherwise noted, the contents of the FDA website (www.fda.gov), both text and graphics, are not copyrighted. They are in the public domain and may be republished, reprinted and otherwise used freely by anyone without the need to obtain permission from FDA. Credit to the U.S. Food and Drug Administration as the source is appreciated but not required. | |
Record name | DEOXYNIVALENOL | |
Source | Hazardous Substances Data Bank (HSDB) | |
URL | https://pubchem.ncbi.nlm.nih.gov/source/hsdb/7245 | |
Description | The Hazardous Substances Data Bank (HSDB) is a toxicology database that focuses on the toxicology of potentially hazardous chemicals. It provides information on human exposure, industrial hygiene, emergency handling procedures, environmental fate, regulatory requirements, nanomaterials, and related areas. The information in HSDB has been assessed by a Scientific Review Panel. | |
Melting Point |
151-153 °C | |
Record name | DEOXYNIVALENOL | |
Source | Hazardous Substances Data Bank (HSDB) | |
URL | https://pubchem.ncbi.nlm.nih.gov/source/hsdb/7245 | |
Description | The Hazardous Substances Data Bank (HSDB) is a toxicology database that focuses on the toxicology of potentially hazardous chemicals. It provides information on human exposure, industrial hygiene, emergency handling procedures, environmental fate, regulatory requirements, nanomaterials, and related areas. The information in HSDB has been assessed by a Scientific Review Panel. | |
Retrosynthesis Analysis
AI-Powered Synthesis Planning: Our tool employs the Template_relevance Pistachio, Template_relevance Bkms_metabolic, Template_relevance Pistachio_ringbreaker, Template_relevance Reaxys, Template_relevance Reaxys_biocatalysis model, leveraging a vast database of chemical reactions to predict feasible synthetic routes.
One-Step Synthesis Focus: Specifically designed for one-step synthesis, it provides concise and direct routes for your target compounds, streamlining the synthesis process.
Accurate Predictions: Utilizing the extensive PISTACHIO, BKMS_METABOLIC, PISTACHIO_RINGBREAKER, REAXYS, REAXYS_BIOCATALYSIS database, our tool offers high-accuracy predictions, reflecting the latest in chemical research and data.
Strategy Settings
Precursor scoring | Relevance Heuristic |
---|---|
Min. plausibility | 0.01 |
Model | Template_relevance |
Template Set | Pistachio/Bkms_metabolic/Pistachio_ringbreaker/Reaxys/Reaxys_biocatalysis |
Top-N result to add to graph | 6 |
Feasible Synthetic Routes
Q1: What is the primary target of deoxynivalenol within cells?
A1: this compound primarily targets the ribosome, specifically the A site, inhibiting protein synthesis [].
Q2: How does this compound binding to the ribosome lead to toxicity?
A2: Binding of this compound to the ribosome triggers a ribotoxic stress response, activating mitogen-activated protein kinases (MAPKs) []. This activation leads to a cascade of downstream effects, including the upregulation of pro-inflammatory cytokines and the disruption of cellular processes [].
Q3: Does this compound affect cell viability?
A3: Yes, this compound exhibits cytotoxic effects and can induce apoptosis in various cell types, including osteoblasts []. This effect is dose-dependent, with higher concentrations causing more significant damage [].
Q4: What is the molecular formula and weight of this compound?
A4: The molecular formula of this compound is C15H20O6, and its molecular weight is 296.32 g/mol.
Q5: Which spectroscopic techniques are used to characterize this compound and its derivatives?
A5: Nuclear Magnetic Resonance (NMR) spectroscopy, particularly 1H and 13C NMR, is crucial for structural elucidation of this compound and its derivatives, including novel modified forms like 3-lactyl-deoxynivalenol and 3-propionyl-deoxynivalenol []. Mass spectrometry (MS) is commonly used for identification and quantification, often coupled with separation techniques like liquid chromatography (LC-MS/MS) [, , , , , , ].
Q6: Is this compound stable under food processing conditions?
A6: this compound exhibits high structural stability, making its removal from contaminated food and feed challenging []. Extrusion processing, a common food manufacturing technique, only results in a 22-37% reduction in this compound levels [].
Q7: Does milling help in reducing this compound contamination in barley?
A7: Milling can significantly reduce this compound levels in barley grain. Milling hulled barley at a 67% rate led to a 90.9% reduction in this compound contamination [].
Q8: How does this compound behave during the brewing process?
A8: this compound persists throughout the brewing process, with 35-52% remaining in the final beer product after filtration [].
Q9: Are there any known catalytic applications of this compound?
A9: The research papers provided do not discuss any catalytic properties or applications of this compound. This compound is primarily studied for its toxic effects rather than any potential catalytic uses.
Q10: Have computational methods been used to study this compound interactions?
A10: Yes, in silico analysis has been employed to investigate the binding of this compound and its derivatives to the ribosome []. These studies show that modifications to the this compound structure can significantly impact its binding affinity and, consequently, its toxicity [].
Q11: How do structural modifications of this compound affect its activity?
A11: Modifications to the this compound structure, such as de-epoxidation, epimerization, or glycosylation, significantly impact its toxicity []. For instance, deepoxy-deoxynivalenol and 3-epi-deoxynivalenol show reduced binding to the ribosome compared to this compound, leading to decreased toxicity []. Glycosylation of this compound into this compound-3-s-D-glucoside (D3G) hinders its binding to the ribosome, effectively reducing its toxicity [].
Q12: Are there strategies to improve the stability or bioavailability of this compound for research purposes?
A12: The provided research papers primarily focus on the toxicological aspects of this compound and its occurrence in food and feed. There is no mention of strategies to enhance its stability or bioavailability for research purposes.
Q13: What are the regulatory limits for this compound in food and feed?
A13: Regulatory limits for this compound vary globally. The European Union has established maximum levels for this compound in various food and feed products []. For example, the maximum level for this compound in unprocessed durum wheat intended for human consumption is 1250 μg/kg [].
Q14: What are the major metabolic pathways of this compound?
A14: this compound undergoes extensive metabolism, primarily in the liver. Major metabolic pathways include glucuronidation, forming this compound-glucuronide, and oxidation, resulting in deepoxy-deoxynivalenol []. These metabolites are generally less toxic than the parent compound and are primarily excreted in urine and feces [].
Q15: What is the role of glucuronidation in this compound metabolism?
A15: Glucuronidation is a major detoxification pathway for this compound. Horses fed a diet contaminated with this compound showed a significant portion of the ingested toxin present in its glucuronide-conjugated form in their plasma []. This suggests that glucuronidation plays a crucial role in reducing the bioavailability and toxicity of this compound.
Q16: Can active carbon prevent the absorption of this compound?
A16: Yes, studies have shown that active carbon, when co-administered with this compound, can completely prevent its absorption in pigs []. This finding highlights the potential of active carbon as a mycotoxin binder to mitigate this compound exposure.
Q17: What in vitro models are used to study this compound toxicity?
A17: The human intestinal Caco-2 cell line is a widely used in vitro model to study this compound toxicity []. This model allows researchers to investigate the effects of this compound on intestinal cell viability, barrier function, and inflammatory responses [].
Q18: How does this compound affect intestinal gene expression?
A18: In ex vivo experiments using pig jejunal explants, this compound exposure led to the upregulation of pro-inflammatory cytokines, indicating an inflammatory response []. Additionally, transcriptomic analysis revealed that this compound alters the expression of numerous genes involved in immune response, oxidative stress, cell death, and other crucial cellular functions [].
Q19: What animal models are used to study this compound toxicity?
A19: Pigs are a relevant animal model for studying this compound toxicity due to their sensitivity to this mycotoxin [, ]. Studies in pigs have been conducted to assess the efficacy of mycotoxin binders, such as activated carbon, in preventing this compound absorption [].
Q20: How does this compound exposure affect rainbow trout?
A20: Rainbow trout exposed to this compound-contaminated feed (1964 μg/kg feed) for 23 days exhibited various physiological changes, including decreased mean corpuscular hemoglobin concentration (MCH) and reduced plasma levels of glucose, cholesterol, and ammonia []. Histopathological examination further revealed damage to the caudal kidney, highlighting the negative impacts of this compound on fish health [].
Q21: Are there known mechanisms of resistance to this compound in plants?
A21: Yes, research suggests that some plants can detoxify this compound through glycosylation, converting it into the less toxic D3G []. This mechanism involves UDP-glucosyltransferases (UGTs), which catalyze the transfer of a glucose molecule to this compound []. Studies using the model cereal Brachypodium distachyon have demonstrated that overexpression of the Bradi5g03300 UGT gene, encoding a UGT, enhances resistance to Fusarium graminearum infection and increases tolerance to this compound [].
Q22: What are the known toxic effects of this compound in animals?
A22: this compound exhibits a wide range of toxic effects in animals, including:
- Immunosuppression: Disrupts immune cell function and increases susceptibility to infections [].
- Growth retardation: Reduces feed intake and impairs nutrient absorption, leading to reduced weight gain [].
- Gastrointestinal disturbances: Causes vomiting, diarrhea, and intestinal damage [].
- Hepatotoxicity: Can damage the liver, potentially leading to liver dysfunction [].
- Reproductive toxicity: May impair reproductive function and development [].
Q23: Are there any biomarkers for this compound exposure?
A23: Urinary this compound and its metabolites, such as this compound-glucuronide, are considered reliable biomarkers of exposure []. Measuring these biomarkers in urine samples provides valuable information on the extent of this compound exposure in individuals and populations [].
Q24: What analytical techniques are used for this compound detection and quantification?
A24: Several analytical methods are employed for the detection and quantification of this compound and its derivatives, including:
- Thin-layer chromatography (TLC): A simple and cost-effective method for initial screening and qualitative analysis [].
- High-performance liquid chromatography (HPLC): Provides high sensitivity and selectivity for this compound analysis, often coupled with UV detection (HPLC-UV) or mass spectrometry (HPLC-MS/MS) [, , ].
- Ultra-high performance liquid chromatography-tandem mass spectrometry (UHPLC-MS/MS): Offers enhanced sensitivity, selectivity, and speed compared to traditional HPLC methods, enabling the simultaneous analysis of multiple mycotoxins, including this compound and its masked forms like this compound-3-glucoside (D3G) [].
Q25: What is the environmental fate of this compound?
A25: The provided research papers primarily focus on the occurrence, detection, and toxicological aspects of this compound. Information regarding its environmental fate, degradation pathways, or potential ecotoxicological effects is not discussed.
Q26: How are analytical methods for this compound validated?
A26: Validation of analytical methods for this compound typically involves evaluating parameters such as:
- Linearity: The ability of the method to produce results directly proportional to the analyte concentration over a specified range [, ].
- Accuracy: The closeness of the measured values to the true value, usually expressed as recovery [, ].
- Precision: The degree of agreement among individual test results under prescribed conditions, commonly assessed as repeatability (intra-day precision) and reproducibility (inter-day precision) [, ].
- Sensitivity: The method's ability to detect and quantify small amounts of the analyte, often expressed as the limit of detection (LOD) and limit of quantification (LOQ) [, ].
- Specificity: The ability of the method to discriminate between the analyte of interest and other components in the sample matrix [, ].
Q27: What are the quality control measures for this compound analysis?
A27: Quality control measures for this compound analysis often involve:
- Use of certified reference materials: Employing certified reference materials with known this compound concentrations allows laboratories to verify the accuracy and reliability of their analytical methods [].
- Regular method validation: Periodic validation of analytical methods ensures their continued suitability and performance for this compound analysis [, ].
- Participation in proficiency testing programs: Participation in proficiency testing schemes helps laboratories demonstrate their competence and identify areas for improvement in this compound testing [].
- Implementation of good laboratory practices (GLP): Adhering to GLP principles ensures data integrity, traceability, and reliability in this compound analysis [].
Q28: Does this compound induce an immune response?
A28: this compound is known to modulate immune responses, primarily through its effects on immune cells [, ]. It can impair the function of various immune cells, including lymphocytes and macrophages, leading to immunosuppression []. This immunosuppressive effect can increase susceptibility to infections and reduce the efficacy of vaccinations [].
Q29: Are there any alternatives or substitutes for this compound in research?
A29: The research papers provided focus on the naturally occurring mycotoxin this compound and do not discuss any alternatives or substitutes for research purposes.
Q30: What is the historical context of this compound research?
A30: this compound was first isolated in the 1970s and has been extensively studied since then due to its widespread occurrence in food and feed crops and its diverse toxicological effects [, ]. Research on this compound has significantly advanced our understanding of its mechanism of action, toxicological profile, and potential risks to human and animal health.
Haftungsausschluss und Informationen zu In-Vitro-Forschungsprodukten
Bitte beachten Sie, dass alle Artikel und Produktinformationen, die auf BenchChem präsentiert werden, ausschließlich zu Informationszwecken bestimmt sind. Die auf BenchChem zum Kauf angebotenen Produkte sind speziell für In-vitro-Studien konzipiert, die außerhalb lebender Organismen durchgeführt werden. In-vitro-Studien, abgeleitet von dem lateinischen Begriff "in Glas", beinhalten Experimente, die in kontrollierten Laborumgebungen unter Verwendung von Zellen oder Geweben durchgeführt werden. Es ist wichtig zu beachten, dass diese Produkte nicht als Arzneimittel oder Medikamente eingestuft sind und keine Zulassung der FDA für die Vorbeugung, Behandlung oder Heilung von medizinischen Zuständen, Beschwerden oder Krankheiten erhalten haben. Wir müssen betonen, dass jede Form der körperlichen Einführung dieser Produkte in Menschen oder Tiere gesetzlich strikt untersagt ist. Es ist unerlässlich, sich an diese Richtlinien zu halten, um die Einhaltung rechtlicher und ethischer Standards in Forschung und Experiment zu gewährleisten.