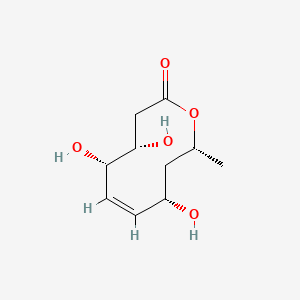
Decarestrictin D
Übersicht
Beschreibung
Decarestrictine D is a 10-membered lactone which potently inhibits cholesterol biosynthesis and shows hypolipidemic activity.
Wissenschaftliche Forschungsanwendungen
Hemmung der Cholesterinbiosynthese
Decarestrictin D wurde als Produkt von Penicillium simplicissimum (Stamm FH-A6090) entdeckt. Es wirkt als neuartiger Inhibitor der De-novo-Cholesterinbiosynthese. Studien mit Zellkulturtests an HEP-G2-Leberzellen und In-vivo-Experimenten an normolipidämischen Ratten haben seine Wirksamkeit in dieser Hinsicht gezeigt .
Antibiotische Eigenschaften
Mittelgroße Lactone, einschließlich this compound, zeigen antibiotische Wirkungen. Ihre strukturelle Flexibilität und konformationelle Vielfalt tragen zu ihrer Aktivität gegen bakterielle Krankheitserreger bei. Forscher haben ihr Potenzial als antimikrobielle Mittel untersucht .
Förderung des Pflanzenwachstums
Die Rolle von this compound in der Pflanzenbiologie ist faszinierend. Es kann das Pflanzenwachstum verbessern, möglicherweise durch Beeinflussung von Hormonsignalwegen oder Nährstoffaufnahme. Forscher untersuchen seine Auswirkungen auf den Ernteertrag und die Stresstoleranz.
Zusammenfassend lässt sich sagen, dass this compound mit seinen vielfältigen biologischen Aktivitäten eine faszinierende Verbindung für weitere Untersuchungen darstellt. Seine potenziellen Anwendungen erstrecken sich auf die Cholesterinregulation, antimikrobielle Therapie, antivirale Strategien, Krebsbehandlung, Diabetesmanagement und landwirtschaftliche Praktiken. Forscher lüften weiterhin seine Geheimnisse, und seine einzigartige Struktur inspiriert weiterhin wissenschaftliche Neugier .
Wirkmechanismus
Mode of Action
The exact mode of action of Decarestrictine D remains incompletely understood. It is known to exhibit inhibitory activity against cholesterol levels , but the specific interactions with its targets and the resulting changes are still under investigation.
Biochemical Pathways
Decarestrictine D is known to affect cholesterol biosynthesis . It is utilized as a receptor for glycosylation reactions to synthesize novel hybrid antibiotics . The specific biochemical pathways and their downstream effects are still being studied.
Pharmacokinetics
Its synthesis involves key steps such as cross-metathesis or ring-closing metathesis and yamaguchi macrolactonization . These steps could potentially impact its bioavailability.
Result of Action
The molecular and cellular effects of Decarestrictine D’s action are primarily its inhibitory activity against cholesterol levels . It is also utilized as a receptor for glycosylation reactions to synthesize novel hybrid antibiotics
Action Environment
The action of Decarestrictine D can be influenced by environmental factors. For instance, under acidic conditions during fermentation, Decarestrictine D is converted into other compounds . This suggests that the compound’s action, efficacy, and stability can be significantly affected by the environment in which it is present.
Biochemische Analyse
Biochemical Properties
Decarestrictine D plays a significant role in biochemical reactions. It exhibits inhibitory activity against cholesterol levels . It interacts with enzymes involved in cholesterol biosynthesis, leading to a decrease in cholesterol production . The nature of these interactions is inhibitory, with Decarestrictine D binding to the active sites of these enzymes and preventing them from catalyzing their respective reactions .
Cellular Effects
Decarestrictine D has profound effects on various types of cells and cellular processes. It influences cell function by inhibiting the biosynthesis of cholesterol, a critical component of cell membranes . This inhibition can impact cell signaling pathways, gene expression, and cellular metabolism, as cholesterol plays a vital role in these processes .
Molecular Mechanism
The molecular mechanism of Decarestrictine D involves its binding interactions with biomolecules, specifically enzymes involved in cholesterol biosynthesis . It acts as an enzyme inhibitor, binding to the active sites of these enzymes and preventing them from catalyzing the conversion of substrates into products . This inhibition leads to a decrease in cholesterol production, impacting various cellular functions .
Metabolic Pathways
Decarestrictine D is involved in the metabolic pathway of cholesterol biosynthesis . It interacts with enzymes in this pathway, acting as an inhibitor and reducing the production of cholesterol .
Eigenschaften
IUPAC Name |
(2R,4S,5Z,7S,8S)-4,7,8-trihydroxy-2-methyl-2,3,4,7,8,9-hexahydrooxecin-10-one | |
---|---|---|
Source | PubChem | |
URL | https://pubchem.ncbi.nlm.nih.gov | |
Description | Data deposited in or computed by PubChem | |
InChI |
InChI=1S/C10H16O5/c1-6-4-7(11)2-3-8(12)9(13)5-10(14)15-6/h2-3,6-9,11-13H,4-5H2,1H3/b3-2-/t6-,7-,8+,9+/m1/s1 | |
Source | PubChem | |
URL | https://pubchem.ncbi.nlm.nih.gov | |
Description | Data deposited in or computed by PubChem | |
InChI Key |
HWMMWMJBUOCCFZ-OJIZAPILSA-N | |
Source | PubChem | |
URL | https://pubchem.ncbi.nlm.nih.gov | |
Description | Data deposited in or computed by PubChem | |
Canonical SMILES |
CC1CC(C=CC(C(CC(=O)O1)O)O)O | |
Source | PubChem | |
URL | https://pubchem.ncbi.nlm.nih.gov | |
Description | Data deposited in or computed by PubChem | |
Isomeric SMILES |
C[C@@H]1C[C@@H](/C=C\[C@@H]([C@H](CC(=O)O1)O)O)O | |
Source | PubChem | |
URL | https://pubchem.ncbi.nlm.nih.gov | |
Description | Data deposited in or computed by PubChem | |
Molecular Formula |
C10H16O5 | |
Source | PubChem | |
URL | https://pubchem.ncbi.nlm.nih.gov | |
Description | Data deposited in or computed by PubChem | |
DSSTOX Substance ID |
DTXSID501017473 | |
Record name | Decarestrictine D | |
Source | EPA DSSTox | |
URL | https://comptox.epa.gov/dashboard/DTXSID501017473 | |
Description | DSSTox provides a high quality public chemistry resource for supporting improved predictive toxicology. | |
Molecular Weight |
216.23 g/mol | |
Source | PubChem | |
URL | https://pubchem.ncbi.nlm.nih.gov | |
Description | Data deposited in or computed by PubChem | |
CAS No. |
127393-89-9 | |
Record name | Decarestrictine D | |
Source | ChemIDplus | |
URL | https://pubchem.ncbi.nlm.nih.gov/substance/?source=chemidplus&sourceid=0127393899 | |
Description | ChemIDplus is a free, web search system that provides access to the structure and nomenclature authority files used for the identification of chemical substances cited in National Library of Medicine (NLM) databases, including the TOXNET system. | |
Record name | Decarestrictine D | |
Source | EPA DSSTox | |
URL | https://comptox.epa.gov/dashboard/DTXSID501017473 | |
Description | DSSTox provides a high quality public chemistry resource for supporting improved predictive toxicology. | |
Retrosynthesis Analysis
AI-Powered Synthesis Planning: Our tool employs the Template_relevance Pistachio, Template_relevance Bkms_metabolic, Template_relevance Pistachio_ringbreaker, Template_relevance Reaxys, Template_relevance Reaxys_biocatalysis model, leveraging a vast database of chemical reactions to predict feasible synthetic routes.
One-Step Synthesis Focus: Specifically designed for one-step synthesis, it provides concise and direct routes for your target compounds, streamlining the synthesis process.
Accurate Predictions: Utilizing the extensive PISTACHIO, BKMS_METABOLIC, PISTACHIO_RINGBREAKER, REAXYS, REAXYS_BIOCATALYSIS database, our tool offers high-accuracy predictions, reflecting the latest in chemical research and data.
Strategy Settings
Precursor scoring | Relevance Heuristic |
---|---|
Min. plausibility | 0.01 |
Model | Template_relevance |
Template Set | Pistachio/Bkms_metabolic/Pistachio_ringbreaker/Reaxys/Reaxys_biocatalysis |
Top-N result to add to graph | 6 |
Feasible Synthetic Routes
Haftungsausschluss und Informationen zu In-Vitro-Forschungsprodukten
Bitte beachten Sie, dass alle Artikel und Produktinformationen, die auf BenchChem präsentiert werden, ausschließlich zu Informationszwecken bestimmt sind. Die auf BenchChem zum Kauf angebotenen Produkte sind speziell für In-vitro-Studien konzipiert, die außerhalb lebender Organismen durchgeführt werden. In-vitro-Studien, abgeleitet von dem lateinischen Begriff "in Glas", beinhalten Experimente, die in kontrollierten Laborumgebungen unter Verwendung von Zellen oder Geweben durchgeführt werden. Es ist wichtig zu beachten, dass diese Produkte nicht als Arzneimittel oder Medikamente eingestuft sind und keine Zulassung der FDA für die Vorbeugung, Behandlung oder Heilung von medizinischen Zuständen, Beschwerden oder Krankheiten erhalten haben. Wir müssen betonen, dass jede Form der körperlichen Einführung dieser Produkte in Menschen oder Tiere gesetzlich strikt untersagt ist. Es ist unerlässlich, sich an diese Richtlinien zu halten, um die Einhaltung rechtlicher und ethischer Standards in Forschung und Experiment zu gewährleisten.