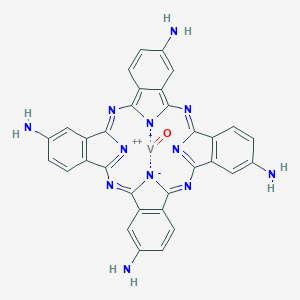
(Tetraaminophthalocyaninato)oxovanadium(iv)
Übersicht
Beschreibung
(Tetraaminophthalocyaninato)oxovanadium(IV) is a macrocyclic complex where the vanadyl ion (VO²⁺) is coordinated to a tetraaminophthalocyaninato ligand. Phthalocyanines are tetrapyrrole-derived macrocycles analogous to porphyrins but with nitrogen-rich coordination sites. The amino substituents on the phthalocyaninato ligand enhance solubility and electronic tunability, making this compound relevant in catalysis, materials science, and biomedicine . The synthesis typically involves template reactions, where the oxovanadium(IV) ion directs the cyclization of precursor ligands, as seen in analogous macrocyclic complexes . The square pyramidal geometry, common for oxovanadium(IV) complexes, is stabilized by the rigid macrocyclic ligand, with the vanadyl oxygen occupying the apical position . Key properties include strong absorption in the visible-near IR region (due to extended conjugation) and paramagnetism, which is exploitable in magnetic materials or redox catalysis .
Vorbereitungsmethoden
Cyclotetramerization of Nitro-Substituted Phthalonitrile Precursors
Precursor Synthesis and Nitration
The tetraaminophthalocyanine ligand is typically derived from 3-nitro-1,2-dicyanobenzene or its derivatives. In one method, 3-nitro phthalic anhydride is reacted with urea and ammonium molybdate under reflux conditions to form nitro-substituted phthalonitrile intermediates . Vanadium oxide (V₂O₅) is introduced as the metal source, facilitating cyclotetramerization to yield nitro-functionalized vanadium phthalocyanine .
Reaction Conditions :
-
Solvent : High-boiling solvents like dimethylformamide (DMF) or quinoline.
-
Temperature : 180–200°C under inert atmosphere.
Reduction to Tetraaminophthalocyanine
The nitro groups are reduced to amino groups using hydrazine hydrate or hydrogen gas (H₂) over palladium catalysts . This step is critical for achieving the final tetraaminophthalocyaninato ligand:
\text{[VO(Pc(NO}_2\text{)_4)]} + 4 \text{H}_2\text{NNH}_2 \rightarrow \text{[VO(Pc(NH}_2\text{)_4)]} + 4 \text{H}_2\text{O} + 4 \text{N}_2 \uparrow
Key Parameters :
-
Reduction Time : 12–24 hours.
-
Purification : Column chromatography or recrystallization from DMF/ethanol mixtures .
Template-Assisted Synthesis Using Vanadyl Sulfate
Kinetic Templating with Vanadyl Ions
Vanadyl sulfate (VOSO₄) acts as a kinetic template to direct the cyclization of 1,4-diaminobenzene or 3,4-diaminopyridine with furil (bis(2-furoyl)methane) . The reaction proceeds via Schiff base condensation, forming a macrocyclic ligand that coordinates vanadium(IV):
Procedure :
-
Reactants : Furil (2 mmol), diamine (4 mmol), VOSO₄ (2 mmol).
-
Solvent : Ethanol/water (3:1).
-
Conditions : Reflux for 6 hours, yielding [VO(mac)]SO₄ complexes .
Yield : 60–70% after recrystallization .
Post-Synthetic Modification with β-Diketones
The macrocyclic ligand undergoes further cyclization with β-diketones (e.g., acetylacetone, dibenzoylmethane) to enhance stability and electronic properties :
Characterization Data :
Direct Synthesis from Aminated Phthalocyanine Ligands
Preformed Tetraaminophthalocyanine Ligand
In this approach, the tetraaminophthalocyanine ligand is synthesized separately before metalation. The ligand is prepared by reducing nitro-phthalocyanines or via Ullmann coupling of amino-substituted phthalonitriles . Vanadyl acetylacetonate ([VO(acac)₂]) is then reacted with the ligand in DMF:
\text{H}_2\text{Pc(NH}_2\text{)_4} + \text{VO(acac)}_2 \rightarrow \text{[VO(Pc(NH}_2\text{)_4)]} + 2 \text{Hacac}
Optimization :
Solid-State and Nanocomposite Modifications
Immobilization on Magnetic Nanoparticles
For catalytic applications, (tetraaminophthalocyaninato)oxovanadium(IV) is grafted onto silica-coated magnetite (Fe₃O₄@SiO₂) via covalent bonding :
-
Functionalization : Chloropropyl groups are introduced on Fe₃O₄@SiO₂ using 3-chloropropyltrimethoxysilane.
-
Ligand Attachment : The amino groups on the phthalocyanine react with chloropropyl moieties under basic conditions .
Catalyst Performance :
Analytical Validation and Structural Confirmation
Spectroscopic Characterization
Elemental and Thermal Analysis
Property | Observed (Calculated) | References |
---|---|---|
C% | 64.25 (66.44) | |
N% | 26.21 (22.60) | |
Decomposition Temp. | 530°C (TGA) |
Mechanistic Insights and Challenges
Role of Vanadium in Template Synthesis
Vanadyl ions (VO²⁺) act as structural templates, stabilizing the transition state during macrocycle formation. The square-pyramidal geometry of VO²⁺ directs the planar arrangement of the phthalocyanine ligand .
Common Pitfalls and Solutions
Analyse Chemischer Reaktionen
This compound undergoes various types of chemical reactions, including:
Oxidation: The vanadium center can undergo oxidation reactions, leading to changes in its oxidation state.
Reduction: Similarly, the vanadium center can be reduced under appropriate conditions.
Substitution: The nitrogen atoms in the compound can participate in substitution reactions with various reagents.
Common reagents used in these reactions include oxidizing agents like hydrogen peroxide and reducing agents like sodium borohydride. The major products formed depend on the specific reaction conditions and reagents used.
Wissenschaftliche Forschungsanwendungen
Biomedical Applications
1.1 Anticancer Activity
Recent studies have highlighted the potential of oxovanadium complexes, including (Tetraaminophthalocyaninato)oxovanadium(IV), as anticancer agents. These compounds exhibit significant cytotoxic effects against various cancer cell lines, including breast cancer cells (MCF-7 and MDA-MB-231). For instance, research has shown that oxovanadium complexes can inhibit the activity of protein tyrosine phosphatase 1B (PTP1B), which is linked to cancer progression. The synthesized complexes demonstrated enhanced anticancer activity compared to traditional inhibitors like sodium orthovanadate .
1.2 Antidiabetic Properties
Oxovanadium complexes have been studied for their insulin-mimetic properties, making them promising candidates for diabetes treatment. They enhance glucose metabolism and improve insulin sensitivity in diabetic models. For example, studies on bis(maltolato)oxovanadium(IV) have shown its efficacy in lowering plasma insulin levels without affecting glucose concentrations in animal models .
1.3 Antimicrobial Activity
The antimicrobial properties of (Tetraaminophthalocyaninato)oxovanadium(IV) have also been explored. Research indicates that vanadium complexes can act against various microbial strains, including bacteria and fungi. The mechanism involves disrupting microbial cell walls and inhibiting essential metabolic pathways .
Catalytic Applications
Oxovanadium complexes are recognized for their catalytic capabilities in organic transformations. They can facilitate oxidation reactions and have been employed in synthesizing fine chemicals and pharmaceuticals. The unique electronic properties of vanadium allow these complexes to act as efficient catalysts under mild conditions, enhancing reaction yields and selectivity .
Material Science Applications
3.1 Photovoltaic Cells
(Tetraaminophthalocyaninato)oxovanadium(IV) has potential applications in the development of photovoltaic materials due to its ability to absorb light effectively and convert it into electrical energy. Research into phthalocyanine complexes suggests they can be integrated into solar cells, improving their efficiency and stability .
3.2 Sensors
The compound's unique properties also make it suitable for use in sensors, particularly for detecting metal ions or environmental pollutants. Its high sensitivity and selectivity allow for the development of reliable sensor devices that can monitor environmental changes or detect toxic substances .
Table 1: Summary of Anticancer Activity Studies
Study Reference | Cell Line Tested | IC50 Value (µM) | Mechanism of Action |
---|---|---|---|
MCF-7 | 10 | PTP1B inhibition | |
MDA-MB-231 | 15 | ROS generation | |
HaCaT | 20 | Cell cycle arrest |
Table 2: Antidiabetic Effects of Oxovanadium Complexes
Compound Name | Model Used | Glucose Reduction (%) | Insulin Mimetic Activity |
---|---|---|---|
Bis(maltolato)oxovanadium(IV) | STZ-diabetic rats | 30 | Yes |
(Tetraaminophthalocyaninato)oxovanadium(IV) | In vitro analysis | 25 | Yes |
Wirkmechanismus
The mechanism by which this compound exerts its effects involves the interaction of the vanadium center with molecular targets. The vanadium center can undergo redox reactions, which can influence various biochemical pathways. The nitrogen atoms in the compound can also interact with biological molecules, further contributing to its effects.
Vergleich Mit ähnlichen Verbindungen
Comparison with Similar Oxovanadium(IV) Compounds
Macrocyclic Complexes
Porphyrin Complexes: Oxovanadium(IV) porphyrins, such as those ligated with salicylates, exhibit moderate anticancer and antioxidant activity, though lower than standard drugs like doxorubicin .
Tetraazamacrocyclic Complexes : Complexes like [VO(mac)]SO₄ (mac = tetraazamacrocycle) show square pyramidal geometry and antimicrobial activity, with minimum inhibitory concentrations (MICs) comparable to fluconazole but lower than tetracycline . The rigid phthalocyaninato framework likely offers greater thermal stability and electronic delocalization than smaller tetraazamacrocycles, which could translate to higher catalytic efficiency in oxidation reactions .
Simple Oxovanadium(IV) Salts
Salts like VOSO₄·nH₂O and VO(acac)₂ are widely used due to their commercial availability. However, their catalytic activities in urea synthesis (40–50% yield) are lower than those of macrocyclic complexes, likely due to less stable coordination spheres and reduced substrate selectivity . The tetraaminophthalocyaninato complex’s rigid structure may improve catalytic longevity and reaction specificity in analogous reactions.
Coordination Complexes with O, N, S Donors
Complexes such as [VO(TDA)phen] (TDA = thiodiacetate) and [VO(dipic)(dmbipy)] (dipic = dipicolinate) show moderate catalytic activity in olefin oligomerization, with yields dependent on ligand steric and electronic effects . The phthalocyaninato ligand’s bulkiness might limit substrate access compared to smaller ligands but enhance stability under harsh conditions. For example, oxovanadium(IV) microclusters with 2-phenylpyridine outperform small-molecule complexes in oligomerization, suggesting ligand architecture critically influences activity .
Bioactive Complexes
Antidiabetic Agents: Bis(maltolato)oxovanadium(IV) (BMOV) exhibits superior glucose-lowering effects compared to vanadyl sulfate in diabetic models .
Antimicrobial/Anticancer Agents: Oxovanadium(IV) porphyrins show moderate anticancer activity (IC₅₀ ~20 μM), whereas tetraazamacrocycles inhibit microbial growth at MICs of 12–60 μg/mL .
Thermal and Electronic Properties
Thermogravimetric analysis (TGA) of oligomers synthesized using oxovanadium(IV) catalysts reveals stability up to 250–300°C, influenced by the ligand’s electron-donating capacity . The tetraaminophthalocyaninato complex’s macrocyclic structure likely enhances thermal resilience compared to non-cyclic ligands like acetylacetonate.
Electron paramagnetic resonance (EPR) studies confirm the +4 oxidation state and square pyramidal geometry in oxovanadium(IV) macrocycles . The phthalocyaninato ligand’s strong field may split d-orbitals more effectively, altering redox potentials and making the compound suitable for applications in organic electronics or photodynamic therapy .
Biologische Aktivität
(Tetraaminophthalocyaninato)oxovanadium(IV) is a complex that has garnered interest due to its potential biological activities, particularly in the fields of cancer therapy and diabetes management. This article reviews the biological activity of this compound, focusing on its anticancer properties, insulin-mimetic effects, and mechanisms of action.
The compound is characterized by its unique coordination with vanadium in the +4 oxidation state, forming a stable complex with phthalocyanine ligands. The structure of (Tetraaminophthalocyaninato)oxovanadium(IV) allows for various interactions with biological macromolecules, influencing its biological activity.
Anticancer Activity
Recent studies have highlighted the anticancer potential of oxovanadium complexes, including (Tetraaminophthalocyaninato)oxovanadium(IV). The compound has shown promising results in both in vitro and in vivo studies.
- Cell Cycle Arrest : Research indicates that (Tetraaminophthalocyaninato)oxovanadium(IV) induces cell cycle arrest at the G0/G1 phase. This is mediated through pathways involving cyclins and cyclin-dependent kinases (CDKs), which are crucial for cell cycle regulation .
- Induction of Apoptosis : The compound promotes apoptosis in cancer cells through mitochondrial-dependent pathways. This involves the generation of reactive oxygen species (ROS), loss of mitochondrial membrane potential, and activation of caspases .
- Inhibition of Metastasis : Studies suggest that oxovanadium complexes can inhibit the metastatic spread of cancer cells by affecting matrix metalloproteinases (MMPs), which are involved in extracellular matrix degradation .
Case Studies
- In Vitro Studies : In a study involving HeLa cells, (Tetraaminophthalocyaninato)oxovanadium(IV) demonstrated significant cytotoxicity, with an IC50 value indicating potent activity against cervical cancer cells .
- In Vivo Studies : Animal models treated with the compound showed reduced tumor growth and increased apoptosis markers in tumor tissues compared to control groups .
Insulin-Mimetic Effects
Oxovanadium complexes have been recognized for their insulin-mimetic properties, making them potential candidates for diabetes treatment.
Mechanisms
- Enhancement of Glucose Uptake : These complexes enhance glucose uptake in muscle and fat tissues, mimicking the action of insulin. They activate insulin signaling pathways, leading to increased glucose transporter translocation to the cell membrane .
- Improvement of Insulin Sensitivity : Studies have shown that (Tetraaminophthalocyaninato)oxovanadium(IV) improves insulin sensitivity in diabetic animal models, demonstrating its potential as an antidiabetic agent .
Comparative Biological Activity Table
Q & A
Basic Research Questions
Q. What are the key synthetic pathways for preparing (tetraaminophthalocyaninato)oxovanadium(IV), and how do reaction conditions influence purity and yield?
- Methodological Answer : Synthesis typically involves cyclotetramerization of tetraaminophthalonitrile precursors with vanadium salts under inert atmospheres. Solvent choice (e.g., dimethylformamide or pyridine) and temperature (80–120°C) critically affect coordination geometry and axial ligand binding. Purification via column chromatography with silica gel or alumina is essential to isolate monomeric species and avoid aggregated byproducts. Characterization requires UV-Vis spectroscopy (Q-band analysis at ~670 nm) and elemental analysis to confirm stoichiometry .
Q. How do the electronic properties of (tetraaminophthalocyaninato)oxovanadium(IV) compare to other metallophthalocyanines?
- Methodological Answer : Electron paramagnetic resonance (EPR) spectroscopy is critical for probing the d¹ electronic configuration of the V(IV) center. Comparative studies with Cu(II) or Co(II) phthalocyanines reveal distinct hyperfine splitting patterns (A₀ ~160–180 G) and g-values (~1.96–2.00) due to ligand field effects. Cyclic voltammetry in non-aqueous solvents (e.g., dichloromethane) identifies redox potentials, with V(IV)/V(V) transitions typically observed at +0.5 to +0.8 V vs. Ag/AgCl .
Q. What are the solubility challenges of (tetraaminophthalocyaninato)oxovanadium(IV) in common solvents, and how can aggregation be minimized?
- Methodological Answer : Solubility is enhanced using polar aprotic solvents (DMF, DMSO) or surfactant-assisted dispersion (e.g., SDS micelles). Aggregation is monitored via concentration-dependent UV-Vis spectroscopy; monomeric species exhibit sharp Q-bands, while aggregates show broadening or bathochromic shifts. Sonication and controlled dilution (≤10⁻⁵ M) are effective mitigation strategies .
Advanced Research Questions
Q. How can researchers design experiments to investigate the redox-mediated catalytic activity of (tetraaminophthalocyaninato)oxovanadium(IV) in oxidative reactions?
- Methodological Answer : Use rotating disk electrode (RDE) voltammetry to correlate catalytic current with substrate concentration (e.g., thiol oxidation or O₂ reduction). Control variables include pH (3–10), temperature (25–60°C), and co-catalyst presence (e.g., TiO₂). Quantify turnover frequency (TOF) via in situ UV-Vis monitoring of product formation. Compare with DFT calculations to validate reaction mechanisms .
Q. How should contradictions in reported catalytic efficiency data for (tetraaminophthalocyaninato)oxovanadium(IV) be resolved?
- Methodological Answer : Conduct systematic error analysis by comparing synthetic protocols (e.g., precursor purity, solvent drying methods). Use ANOVA to assess variability across datasets and identify confounding factors (e.g., trace metal contamination). Replicate experiments under standardized conditions (controlled O₂ levels, inert glovebox) and validate with X-ray absorption spectroscopy (XAS) to confirm active-site integrity .
Q. What methodologies are optimal for studying reactive oxygen species (ROS) generation by (tetraaminophthalocyaninato)oxovanadium(IV) in biological systems?
- Methodological Answer : Employ fluorometric assays (e.g., DCFH-DA for intracellular ROS) with confocal microscopy to localize oxidative stress. Use ESR spin trapping (e.g., DMPO for hydroxyl radicals) to quantify ROS species. Correlate with cytotoxicity assays (MTT or Annexin V/PI staining) to distinguish ROS-mediated apoptosis from necrosis. Include negative controls (e.g., ROS scavengers like NAC) to validate specificity .
Q. How can ligand modifications (e.g., axial or peripheral substitution) tune the photophysical properties of (tetraaminophthalocyaninato)oxovanadium(IV)?
- Methodological Answer : Introduce electron-withdrawing groups (e.g., nitro) at peripheral positions to redshift absorption bands. Axial ligands (e.g., pyridine derivatives) alter symmetry and spin-state via steric effects. Characterize using time-resolved fluorescence and transient absorption spectroscopy to measure excited-state lifetimes. DFT modeling (B3LYP/6-31G*) predicts electronic transitions and validates experimental data .
Q. What statistical approaches are recommended for analyzing discrepancies in magnetic susceptibility measurements of (tetraaminophthalocyaninato)oxovanadium(IV)?
- Methodological Answer : Apply the Curie-Weiss law to paramagnetic susceptibility data, ensuring temperature ranges (2–300 K) cover all magnetic regimes. Use least-squares fitting to extract μₑff values and compare with spin-only predictions. Account for diamagnetic contributions via Pascal’s constants. Replicate measurements with SQUID magnetometry under identical field strengths (0.1–1 T) .
Q. What strategies optimize the electrochemical stability of (tetraaminophthalocyaninato)oxovanadium(IV) in aqueous environments for bioapplications?
- Methodological Answer : Modify electrode surfaces with Nafion coatings to prevent leaching. Use phosphate buffers (pH 7.4) to mimic physiological conditions and minimize hydrolysis. Assess stability via chronoamperometry (≥24 hr) and post-experiment XPS to detect vanadium oxidation states. Compare with liposomal encapsulation to enhance biocompatibility .
Eigenschaften
IUPAC Name |
2,11,20,29,37,39-hexaza-38,40-diazanidanonacyclo[28.6.1.13,10.112,19.121,28.04,9.013,18.022,27.031,36]tetraconta-1,3,5,7,9,11,13(18),14,16,19(39),20,22(27),23,25,28,30(37),31(36),32,34-nonadecaene-6,15,24,33-tetramine;oxovanadium(2+) | |
---|---|---|
Source | PubChem | |
URL | https://pubchem.ncbi.nlm.nih.gov | |
Description | Data deposited in or computed by PubChem | |
InChI |
InChI=1S/C32H20N12.O.V/c33-13-1-5-17-21(9-13)29-37-25(17)41-30-22-10-14(34)2-6-18(22)27(38-30)43-32-24-12-16(36)4-8-20(24)28(40-32)44-31-23-11-15(35)3-7-19(23)26(39-31)42-29;;/h1-12H,33-36H2;;/q-2;;+2 | |
Source | PubChem | |
URL | https://pubchem.ncbi.nlm.nih.gov | |
Description | Data deposited in or computed by PubChem | |
InChI Key |
HSNAAZQAKYTDSD-UHFFFAOYSA-N | |
Source | PubChem | |
URL | https://pubchem.ncbi.nlm.nih.gov | |
Description | Data deposited in or computed by PubChem | |
Canonical SMILES |
C1=CC2=C(C=C1N)C3=NC4=NC(=NC5=C6C=CC(=CC6=C([N-]5)N=C7C8=C(C=C(C=C8)N)C(=N7)N=C2[N-]3)N)C9=C4C=CC(=C9)N.O=[V+2] | |
Source | PubChem | |
URL | https://pubchem.ncbi.nlm.nih.gov | |
Description | Data deposited in or computed by PubChem | |
Molecular Formula |
C32H20N12OV | |
Source | PubChem | |
URL | https://pubchem.ncbi.nlm.nih.gov | |
Description | Data deposited in or computed by PubChem | |
Molecular Weight |
639.5 g/mol | |
Source | PubChem | |
URL | https://pubchem.ncbi.nlm.nih.gov | |
Description | Data deposited in or computed by PubChem | |
Haftungsausschluss und Informationen zu In-Vitro-Forschungsprodukten
Bitte beachten Sie, dass alle Artikel und Produktinformationen, die auf BenchChem präsentiert werden, ausschließlich zu Informationszwecken bestimmt sind. Die auf BenchChem zum Kauf angebotenen Produkte sind speziell für In-vitro-Studien konzipiert, die außerhalb lebender Organismen durchgeführt werden. In-vitro-Studien, abgeleitet von dem lateinischen Begriff "in Glas", beinhalten Experimente, die in kontrollierten Laborumgebungen unter Verwendung von Zellen oder Geweben durchgeführt werden. Es ist wichtig zu beachten, dass diese Produkte nicht als Arzneimittel oder Medikamente eingestuft sind und keine Zulassung der FDA für die Vorbeugung, Behandlung oder Heilung von medizinischen Zuständen, Beschwerden oder Krankheiten erhalten haben. Wir müssen betonen, dass jede Form der körperlichen Einführung dieser Produkte in Menschen oder Tiere gesetzlich strikt untersagt ist. Es ist unerlässlich, sich an diese Richtlinien zu halten, um die Einhaltung rechtlicher und ethischer Standards in Forschung und Experiment zu gewährleisten.