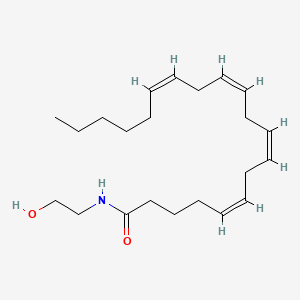
Anandamide
Übersicht
Beschreibung
Anandamide, also known as N-arachidonoylethanolamine, is a fatty acid neurotransmitter derived from arachidonic acid. It is part of the endocannabinoid system and was first discovered in 1992 by Raphael Mechoulam and his team. The name “this compound” is derived from the Sanskrit word “ananda,” meaning “bliss” or “joy,” reflecting its role in promoting feelings of happiness and well-being . This compound interacts with cannabinoid receptors in the brain, similar to the psychoactive compound tetrahydrocannabinol found in cannabis .
Vorbereitungsmethoden
Synthetische Wege und Reaktionsbedingungen: Anandamid wird durch die enzymatische Umwandlung von N-Arachidonoylphosphatidylethanolamin synthetisiert. Dieser Prozess beinhaltet die Wirkung von Phospholipase D, die Anandamid von seinem Vorläufer freigibt . Die Synthese kann in vitro mit isolierten Enzymen oder in vivo in biologischen Systemen durchgeführt werden.
Industrielle Produktionsmethoden: Die industrielle Produktion von Anandamid beinhaltet typischerweise die Extraktion und Reinigung aus biologischen Geweben wie Tiergehirnen, wo es natürlich vorkommt. Fortschrittliche Techniken wie die Hochleistungsflüssigchromatographie werden verwendet, um Anandamid aus komplexen biologischen Gemischen zu isolieren und zu reinigen .
Analyse Chemischer Reaktionen
Table 1: Biosynthetic Pathways of Anandamide
-
Key Insight : The PLC/phosphatase pathway is critical in inflammatory contexts, while NAPE-PLD dominates under physiological conditions .
Metabolic Degradation
This compound is rapidly hydrolyzed by FAAH into arachidonic acid and ethanolamine. Alternative oxidative pathways also exist:
Table 2: Metabolic Pathways of this compound
-
Pharmacological Note : Paracetamol (acetaminophen) is metabolized by FAAH into AM404, a compound that inhibits this compound reuptake and activates TRPV1 receptors, enhancing its analgesic effects .
Transport Mechanisms
This compound’s membrane transport involves lipid-binding partners and heat shock proteins:
Key Findings:
-
Cholesterol interaction : this compound preferentially binds cholesterol via hydrogen bonding, forming a complex that facilitates its movement to the CB1 receptor .
-
Heat shock proteins (Hsp70s) : Mediate intracellular transport of this compound, ensuring its delivery to metabolic enzymes like FAAH .
-
Ceramide binding : Competes with cholesterol for this compound binding, potentially modulating endocannabinoid signaling in lipid-rich membranes .
Table 3: Compounds Affecting this compound Levels
Research Implications
-
Neuropsychiatric Disorders : Reduced NAPE-PLD expression and elevated FAAH activity correlate with autism spectrum disorder (ASD) phenotypes in rodent models .
-
Liver Regeneration : this compound synthesized via FAAH-mediated conjugation promotes hepatocyte proliferation via CB1/CB2 receptors, highlighting a non-canonical biosynthetic route .
-
Tool Development : High-throughput screening identified NAPE-PLD inhibitors (e.g., LEI-401) to study this compound’s role in fear memory erasure .
Wissenschaftliche Forschungsanwendungen
Immunological Applications
Anandamide has been shown to modulate immune responses through its action on cannabinoid receptors, particularly CB1 and CB2 receptors.
Key Findings:
- This compound influences the differentiation and function of monocyte-derived Langerhans cells, promoting a Th1 phenotype while reducing pro-inflammatory cytokine production such as interleukin-6 and tumor necrosis factor-alpha at specific concentrations .
- The anti-inflammatory effects of this compound are significant in conditions like psoriasis and atopic dermatitis, suggesting its potential as a therapeutic agent in managing inflammatory skin diseases .
Table 1: Effects of this compound on Immune Cells
Concentration (nM) | Cytokines Affected | Effect |
---|---|---|
3 - 30 | IL-6, IL-8 | Decreased production |
300 - 3000 | TNFα, IFNγ, IL-4 | Inhibition of production |
Neuroscience Applications
This compound's role in sleep regulation has been highlighted in studies demonstrating its soporific effects mediated through adenosine pathways.
Study Overview:
- In a controlled study with rats, systemic administration of this compound resulted in increased levels of adenosine in the basal forebrain, correlating with enhanced slow-wave sleep . The effects were blocked by the CB1 receptor antagonist SR141716A, indicating that the sleep-inducing properties are primarily mediated through the CB1 receptor.
Table 2: Impact of this compound on Sleep
Measurement | Result |
---|---|
Adenosine Levels | Increased post-anandamide injection |
Slow-Wave Sleep Duration | Significant increase observed |
Psychiatric Applications
Research indicates that this compound may alleviate symptoms associated with psychiatric disorders.
Case Study Insights:
- A study suggests that cannabidiol enhances this compound signaling, which may help alleviate psychotic symptoms in schizophrenia patients. This interaction underscores the potential for developing treatments focusing on endocannabinoid modulation for mental health disorders .
Table 3: this compound and Psychiatric Disorders
Disorder | Mechanism | Outcome |
---|---|---|
Schizophrenia | Enhanced signaling via cannabidiol | Reduction in psychotic symptoms |
Cardiovascular Health
The relationship between this compound and cardiovascular health is gaining attention due to its potential therapeutic effects.
Research Findings:
- This compound interacts with the cardiovascular system by influencing nitric oxide signaling and renal physiology. This interaction is crucial for understanding how this compound could be utilized in developing new therapies for cardiovascular diseases .
Table 4: this compound's Effects on Cardiovascular Health
Parameter | Effect |
---|---|
Nitric Oxide Levels | Modulation observed |
Renal Function | Potential therapeutic implications |
Wirkmechanismus
Anandamide exerts its effects by binding to cannabinoid receptors, primarily CB1 and CB2, located in the central nervous system and peripheral tissues, respectively . Upon binding, this compound activates G-protein signaling pathways, leading to various biological responses, including modulation of neurotransmitter release, pain perception, and immune function . This compound also interacts with the vanilloid receptor TRPV1, contributing to its role in pain modulation .
Vergleich Mit ähnlichen Verbindungen
2-Arachidonoylglycerol (2-AG): Another endocannabinoid that interacts with cannabinoid receptors but differs in its chemical structure and synthesis pathways.
Tetrahydrocannabinol (THC): A plant-derived cannabinoid found in cannabis that shares similar receptor interactions with anandamide but has a different origin and structure.
Uniqueness of this compound: this compound is unique due to its endogenous origin and its role as a naturally occurring neurotransmitter in the human body. Unlike tetrahydrocannabinol, which is exogenous, this compound is produced within the body and plays a crucial role in maintaining homeostasis through the endocannabinoid system .
Biologische Aktivität
Anandamide (AEA), an endocannabinoid, plays a crucial role in various physiological processes through its interaction with cannabinoid receptors, primarily CB1 and CB2. This article delves into the biological activity of this compound, highlighting its mechanisms, effects on behavior, and implications for health.
This compound is synthesized from N-arachidonoyl phosphatidylethanolamine (NAPE) through the action of enzymes such as N-acylphosphatidylethanolamine-specific phospholipase D (NAPE-PLD) and phospholipases A2 and C . Once synthesized, this compound binds to cannabinoid receptors, influencing various signaling pathways.
Table 1: this compound Receptor Interactions
Receptor Type | Location | Primary Effects |
---|---|---|
CB1 | Central Nervous System | Modulates neurotransmitter release, pain perception, mood regulation |
CB2 | Peripheral tissues | Involved in immune response modulation |
2. Behavioral Effects
Research indicates that this compound has significant behavioral effects, particularly in animal models. For instance, studies involving FAAH knockout mice (which lack the enzyme responsible for this compound degradation) show that these mice exhibit enhanced sensitivity to this compound, leading to pronounced analgesic and sedative effects .
Case Study: FAAH Knockout Mice
- Objective : To assess the behavioral responses to this compound.
- Findings :
- This compound administration resulted in:
- 84% reduction in spontaneous activity
- Significant analgesia (89% maximum effect)
- Induction of cataleptic behavior (88% of test group)
- Hypothermia (7.9°C decrease in rectal temperature)
- This compound administration resulted in:
3. Cognitive and Neurochemical Effects
This compound also influences cognitive functions and neurochemical pathways. A study demonstrated that low doses of this compound improved food intake and cognitive function in mice subjected to dietary restrictions. It was observed that this compound administration increased norepinephrine and dopamine levels while decreasing serotonin concentrations .
Table 2: Neurotransmitter Changes Post-Anandamide Administration
Neurotransmitter | Change Observed | Statistical Significance |
---|---|---|
Norepinephrine | Increased | P < 0.01 |
Dopamine | Increased | P < 0.05 |
Serotonin | Decreased | P < 0.001 |
4. Sleep Induction and Adenosine Levels
This compound has been shown to enhance sleep by increasing extracellular adenosine levels. In a microdialysis study with rats, this compound administration significantly elevated adenosine levels and promoted slow-wave sleep .
5. Clinical Implications
The modulation of this compound levels has therapeutic potential, particularly in psychiatric disorders. A recent clinical trial indicated that cannabidiol (CBD) treatment could influence plasma this compound levels in individuals with cannabis use disorder, suggesting a possible avenue for managing anxiety and depression related to cannabis withdrawal .
6. Summary of Research Findings
This compound's biological activity encompasses a range of effects on behavior, cognition, and physiological processes through its interaction with cannabinoid receptors. Its rapid catabolism by FAAH limits its duration of action but also highlights the potential for pharmacological interventions targeting the endocannabinoid system.
Table 3: Summary of this compound's Biological Activities
Activity | Description |
---|---|
Pain Relief | Analgesic effects via CB1 receptor activation |
Mood Regulation | Modulation of neurotransmitter release |
Cognitive Enhancement | Improvement in learning and memory functions |
Sleep Induction | Increases in adenosine levels promoting sleep |
Q & A
Basic Research Questions
Q. What are the primary enzymatic pathways for anandamide synthesis and degradation in neural tissues?
this compound is synthesized via two main pathways:
- NAPE-PLD pathway : N-acylphosphatidylethanolamine phospholipase D (NAPE-PLD) cleaves N-arachidonoyl phosphatidylethanolamine (NAPE) to produce this compound . This pathway is predominant in brain regions like the hippocampus and thalamus .
- PLC/phosphatase pathway : Phospholipase C (PLC) generates phosphothis compound from NAPE, which is dephosphorylated by phosphatases (e.g., PTPN22) to yield this compound. This pathway is critical in macrophages under inflammatory conditions .
Degradation occurs via fatty acid amide hydrolase (FAAH) , which hydrolyzes this compound into arachidonic acid and ethanolamine. FAAH inhibitors (e.g., URB597) are used experimentally to prolong this compound signaling .
Methodological Note: Subcellular fractionation of brain tissue and radiolabeled substrate assays (e.g., [¹⁴C]ethanolamine) are key for studying synthesis kinetics .
Q. How are in vivo behavioral effects of this compound assessed experimentally?
Behavioral studies often use:
- Rodent models : Electrically evoked twitch responses (e.g., mouse vas deferens assay) to test cannabinoid receptor agonism .
- Attention-task paradigms : Dose-response analyses with this compound, controlling for time-dependent effects (data collected within 10 minutes post-administration) .
- Statistical frameworks : Mixed-effects models (e.g., SAS Proc Mixed) for repeated measures, with post-hoc Tukey tests to adjust for multiple comparisons .
Key Consideration: Short half-life of this compound necessitates precise timing for data collection to avoid confounding degradation effects .
Q. What techniques validate this compound’s interaction with cannabinoid receptors?
- Radioligand binding assays : Competitive inhibition studies using synaptosomal membranes and radiolabeled probes (e.g., [³H]CP55,940) confirm receptor affinity .
- Functional assays : Adenylate cyclase inhibition or calcium channel modulation in transfected cells (e.g., CHO cells expressing CB1/CB2) .
- Electrophysiology : Hippocampal slice recordings demonstrate this compound’s inhibition of long-term potentiation (LTP), linking it to memory modulation .
Advanced Research Questions
Q. How can contradictory findings on this compound transport mechanisms be resolved?
Two hypotheses exist:
- Carrier-mediated transport : Supported by inhibition studies with AM404, which blocks high-affinity uptake in neurons and astrocytes .
- Simple diffusion : Evidence from initial uptake rates (<1 minute) in neuroblastoma cells shows no saturation, suggesting diffusion-driven transport .
Methodological Insight: Discrepancies arise from experimental timelines. Prolonged incubations (>5 minutes) reflect FAAH-mediated degradation saturation, not transport . Use kinetic assays with short time frames and FAAH-knockout models to isolate transport mechanisms.
Q. What role does this compound play in embryo implantation and fertility?
- Blastocyst implantation : this compound levels peak during ovulation and correlate with estradiol, modulating uterine receptivity. Excess this compound (e.g., from THC) disrupts implantation .
- Clinical relevance : Hair this compound concentrations are proposed as biomarkers for infertility, though predictive validity remains unestablished .
Experimental Models: In vitro embryo co-culture systems and LC-MS quantification of uterine fluid this compound are used to study thresholds for successful implantation .
Q. How does this compound interact with non-cannabinoid receptor systems (e.g., TRPV1 or NO pathways)?
- TRPV1 activation : this compound binds vanilloid receptors, contributing to nociception. This is studied using TRPV1 antagonists (e.g., capsazepine) in thermal hyperalgesia models .
- NO-mediated vasodilation : In renal vasculature, this compound induces CB1-dependent nitric oxide release, measured via L-NAME-sensitive arteriole dilation in perfused kidney assays .
Advanced Technique: Simultaneous measurement of NO (e.g., Griess assay) and this compound (LC-MS/MS) in endothelial cell cultures .
Q. What epigenetic mechanisms regulate this compound signaling?
Emerging research highlights:
- DNA methylation : FAAH promoter methylation inversely correlates with this compound degradation rates in stress models.
- Histone modification : HDAC inhibitors upregulate CB1 expression in neuronal cultures, enhancing this compound sensitivity .
Future Directions: Single-cell RNA-seq and ChIP-seq in FAAH-knockout models to map chromatin accessibility changes .
Q. Future Research Directions
Eigenschaften
IUPAC Name |
(5Z,8Z,11Z,14Z)-N-(2-hydroxyethyl)icosa-5,8,11,14-tetraenamide | |
---|---|---|
Source | PubChem | |
URL | https://pubchem.ncbi.nlm.nih.gov | |
Description | Data deposited in or computed by PubChem | |
InChI |
InChI=1S/C22H37NO2/c1-2-3-4-5-6-7-8-9-10-11-12-13-14-15-16-17-18-19-22(25)23-20-21-24/h6-7,9-10,12-13,15-16,24H,2-5,8,11,14,17-21H2,1H3,(H,23,25)/b7-6-,10-9-,13-12-,16-15- | |
Source | PubChem | |
URL | https://pubchem.ncbi.nlm.nih.gov | |
Description | Data deposited in or computed by PubChem | |
InChI Key |
LGEQQWMQCRIYKG-DOFZRALJSA-N | |
Source | PubChem | |
URL | https://pubchem.ncbi.nlm.nih.gov | |
Description | Data deposited in or computed by PubChem | |
Canonical SMILES |
CCCCCC=CCC=CCC=CCC=CCCCC(=O)NCCO | |
Source | PubChem | |
URL | https://pubchem.ncbi.nlm.nih.gov | |
Description | Data deposited in or computed by PubChem | |
Isomeric SMILES |
CCCCC/C=C\C/C=C\C/C=C\C/C=C\CCCC(=O)NCCO | |
Source | PubChem | |
URL | https://pubchem.ncbi.nlm.nih.gov | |
Description | Data deposited in or computed by PubChem | |
Molecular Formula |
C22H37NO2 | |
Source | PubChem | |
URL | https://pubchem.ncbi.nlm.nih.gov | |
Description | Data deposited in or computed by PubChem | |
DSSTOX Substance ID |
DTXSID301017453 | |
Record name | Anandamide | |
Source | EPA DSSTox | |
URL | https://comptox.epa.gov/dashboard/DTXSID301017453 | |
Description | DSSTox provides a high quality public chemistry resource for supporting improved predictive toxicology. | |
Molecular Weight |
347.5 g/mol | |
Source | PubChem | |
URL | https://pubchem.ncbi.nlm.nih.gov | |
Description | Data deposited in or computed by PubChem | |
Physical Description |
Solid | |
Record name | Anandamide | |
Source | Human Metabolome Database (HMDB) | |
URL | http://www.hmdb.ca/metabolites/HMDB0004080 | |
Description | The Human Metabolome Database (HMDB) is a freely available electronic database containing detailed information about small molecule metabolites found in the human body. | |
Explanation | HMDB is offered to the public as a freely available resource. Use and re-distribution of the data, in whole or in part, for commercial purposes requires explicit permission of the authors and explicit acknowledgment of the source material (HMDB) and the original publication (see the HMDB citing page). We ask that users who download significant portions of the database cite the HMDB paper in any resulting publications. | |
CAS No. |
94421-68-8 | |
Record name | Anandamide | |
Source | CAS Common Chemistry | |
URL | https://commonchemistry.cas.org/detail?cas_rn=94421-68-8 | |
Description | CAS Common Chemistry is an open community resource for accessing chemical information. Nearly 500,000 chemical substances from CAS REGISTRY cover areas of community interest, including common and frequently regulated chemicals, and those relevant to high school and undergraduate chemistry classes. This chemical information, curated by our expert scientists, is provided in alignment with our mission as a division of the American Chemical Society. | |
Explanation | The data from CAS Common Chemistry is provided under a CC-BY-NC 4.0 license, unless otherwise stated. | |
Record name | Anandamide | |
Source | ChemIDplus | |
URL | https://pubchem.ncbi.nlm.nih.gov/substance/?source=chemidplus&sourceid=0094421688 | |
Description | ChemIDplus is a free, web search system that provides access to the structure and nomenclature authority files used for the identification of chemical substances cited in National Library of Medicine (NLM) databases, including the TOXNET system. | |
Record name | Anandamide | |
Source | EPA DSSTox | |
URL | https://comptox.epa.gov/dashboard/DTXSID301017453 | |
Description | DSSTox provides a high quality public chemistry resource for supporting improved predictive toxicology. | |
Record name | ANANDAMIDE | |
Source | FDA Global Substance Registration System (GSRS) | |
URL | https://gsrs.ncats.nih.gov/ginas/app/beta/substances/UR5G69TJKH | |
Description | The FDA Global Substance Registration System (GSRS) enables the efficient and accurate exchange of information on what substances are in regulated products. Instead of relying on names, which vary across regulatory domains, countries, and regions, the GSRS knowledge base makes it possible for substances to be defined by standardized, scientific descriptions. | |
Explanation | Unless otherwise noted, the contents of the FDA website (www.fda.gov), both text and graphics, are not copyrighted. They are in the public domain and may be republished, reprinted and otherwise used freely by anyone without the need to obtain permission from FDA. Credit to the U.S. Food and Drug Administration as the source is appreciated but not required. | |
Record name | Anandamide | |
Source | Human Metabolome Database (HMDB) | |
URL | http://www.hmdb.ca/metabolites/HMDB0004080 | |
Description | The Human Metabolome Database (HMDB) is a freely available electronic database containing detailed information about small molecule metabolites found in the human body. | |
Explanation | HMDB is offered to the public as a freely available resource. Use and re-distribution of the data, in whole or in part, for commercial purposes requires explicit permission of the authors and explicit acknowledgment of the source material (HMDB) and the original publication (see the HMDB citing page). We ask that users who download significant portions of the database cite the HMDB paper in any resulting publications. | |
Synthesis routes and methods
Procedure details
Retrosynthesis Analysis
AI-Powered Synthesis Planning: Our tool employs the Template_relevance Pistachio, Template_relevance Bkms_metabolic, Template_relevance Pistachio_ringbreaker, Template_relevance Reaxys, Template_relevance Reaxys_biocatalysis model, leveraging a vast database of chemical reactions to predict feasible synthetic routes.
One-Step Synthesis Focus: Specifically designed for one-step synthesis, it provides concise and direct routes for your target compounds, streamlining the synthesis process.
Accurate Predictions: Utilizing the extensive PISTACHIO, BKMS_METABOLIC, PISTACHIO_RINGBREAKER, REAXYS, REAXYS_BIOCATALYSIS database, our tool offers high-accuracy predictions, reflecting the latest in chemical research and data.
Strategy Settings
Precursor scoring | Relevance Heuristic |
---|---|
Min. plausibility | 0.01 |
Model | Template_relevance |
Template Set | Pistachio/Bkms_metabolic/Pistachio_ringbreaker/Reaxys/Reaxys_biocatalysis |
Top-N result to add to graph | 6 |
Feasible Synthetic Routes
Haftungsausschluss und Informationen zu In-Vitro-Forschungsprodukten
Bitte beachten Sie, dass alle Artikel und Produktinformationen, die auf BenchChem präsentiert werden, ausschließlich zu Informationszwecken bestimmt sind. Die auf BenchChem zum Kauf angebotenen Produkte sind speziell für In-vitro-Studien konzipiert, die außerhalb lebender Organismen durchgeführt werden. In-vitro-Studien, abgeleitet von dem lateinischen Begriff "in Glas", beinhalten Experimente, die in kontrollierten Laborumgebungen unter Verwendung von Zellen oder Geweben durchgeführt werden. Es ist wichtig zu beachten, dass diese Produkte nicht als Arzneimittel oder Medikamente eingestuft sind und keine Zulassung der FDA für die Vorbeugung, Behandlung oder Heilung von medizinischen Zuständen, Beschwerden oder Krankheiten erhalten haben. Wir müssen betonen, dass jede Form der körperlichen Einführung dieser Produkte in Menschen oder Tiere gesetzlich strikt untersagt ist. Es ist unerlässlich, sich an diese Richtlinien zu halten, um die Einhaltung rechtlicher und ethischer Standards in Forschung und Experiment zu gewährleisten.