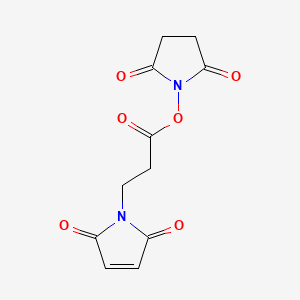
N-Succinimidyl 3-maleimidopropionate
Übersicht
Beschreibung
N-Succinimidyl 3-maleimidopropionate (also known as SMP or BMPS) is a compound with the molecular formula C11H10N2O6 . It is used as an aliphatic heterobifunctional crosslinker reagent, which means it can be used to connect two different molecules together .
Synthesis Analysis
The synthesis of N-Succinimidyl 3-maleimidopropionate has been reported in the literature. For example, a carbon-14 labeled version of the molecule was prepared for disposition studies of PEGylated biologics .Molecular Structure Analysis
The molecular structure of N-Succinimidyl 3-maleimidopropionate includes a maleimide group and an NHS ester . The InChI representation of the molecule isInChI=1S/C11H10N2O6/c14-7-1-2-8(15)12(7)6-5-11(18)19-13-9(16)3-4-10(13)17/h1-2H,3-6H2
. Chemical Reactions Analysis
The NHS ester in N-Succinimidyl 3-maleimidopropionate can be used to label the primary amines (-NH2) of proteins, amine-modified oligonucleotides, and other amine-containing molecules . The maleimide group will react with a thiol group to form a covalent bond, enabling the connection of a biomolecule with a thiol .Physical And Chemical Properties Analysis
N-Succinimidyl 3-maleimidopropionate has a molecular weight of 266.21 g/mol . It is a solid at 20 degrees Celsius and should be stored at a temperature between 0-10°C . It is sensitive to moisture and heat .Wissenschaftliche Forschungsanwendungen
Radioiodination of Antibodies
BMPS has been utilized in the radioiodination of antibodies, which is a critical process in radioimmunodetection and radioimmunotherapy . The compound facilitates the labeling of monoclonal antibodies with radioactive iodine, enhancing the detection and treatment of various diseases, including cancer.
Protein Thiolation
The reagent is used in protein thiolation, a technique that introduces sulfhydryl groups into proteins . This modification is essential for studying protein structure and function, as well as for developing therapeutic proteins.
Preparation of Hapten-Protein Conjugates
In immunology research, BMPS is employed to conjugate haptens to carrier proteins . This process is fundamental for the production of specific antibodies against small molecules, which are not immunogenic on their own.
Fluorescence Immunoassays
BMPS is instrumental in attaching fluorogenic substrates to peptidic antigens . This application is vital for fluorescence immunoassays, which are used to detect the presence of specific antigens or antibodies in a sample.
Molecular Imaging
The compound has been used in molecular imaging, particularly in immuno-spin-trapping techniques combined with molecular magnetic resonance imaging (mMRI) . This innovative approach allows for the in vivo detection of free radicals within tissues, offering insights into various pathological processes.
Cross-Linking Reagent for Bioconjugation
As a heterobifunctional cross-linker, BMPS is used to cross-link molecules like proteins and peptides to other entities, such as drugs or imaging agents . This application is crucial in the development of targeted therapies and diagnostic tools.
Safety And Hazards
Zukünftige Richtungen
N-Succinimidyl 3-maleimidopropionate has been used in the synthesis of PEGylated biologics , and as a cross-linker in the preparation of protein clusters for use as artificial O2 carriers . These applications suggest potential future directions for the use of this compound in biomedical research and therapeutics.
Relevant Papers The synthesis of a carbon-14 labeled version of N-Succinimidyl 3-maleimidopropionate for disposition studies of PEGylated biologics has been reported . Additionally, the compound has been used in the preparation of protein clusters for use as artificial O2 carriers .
Eigenschaften
IUPAC Name |
(2,5-dioxopyrrolidin-1-yl) 3-(2,5-dioxopyrrol-1-yl)propanoate | |
---|---|---|
Source | PubChem | |
URL | https://pubchem.ncbi.nlm.nih.gov | |
Description | Data deposited in or computed by PubChem | |
InChI |
InChI=1S/C11H10N2O6/c14-7-1-2-8(15)12(7)6-5-11(18)19-13-9(16)3-4-10(13)17/h1-2H,3-6H2 | |
Source | PubChem | |
URL | https://pubchem.ncbi.nlm.nih.gov | |
Description | Data deposited in or computed by PubChem | |
InChI Key |
JKHVDAUOODACDU-UHFFFAOYSA-N | |
Source | PubChem | |
URL | https://pubchem.ncbi.nlm.nih.gov | |
Description | Data deposited in or computed by PubChem | |
Canonical SMILES |
C1CC(=O)N(C1=O)OC(=O)CCN2C(=O)C=CC2=O | |
Source | PubChem | |
URL | https://pubchem.ncbi.nlm.nih.gov | |
Description | Data deposited in or computed by PubChem | |
Molecular Formula |
C11H10N2O6 | |
Source | PubChem | |
URL | https://pubchem.ncbi.nlm.nih.gov | |
Description | Data deposited in or computed by PubChem | |
DSSTOX Substance ID |
DTXSID30404976 | |
Record name | 1-{3-[(2,5-Dioxopyrrolidin-1-yl)oxy]-3-oxopropyl}-1H-pyrrole-2,5-dione | |
Source | EPA DSSTox | |
URL | https://comptox.epa.gov/dashboard/DTXSID30404976 | |
Description | DSSTox provides a high quality public chemistry resource for supporting improved predictive toxicology. | |
Molecular Weight |
266.21 g/mol | |
Source | PubChem | |
URL | https://pubchem.ncbi.nlm.nih.gov | |
Description | Data deposited in or computed by PubChem | |
Product Name |
N-Succinimidyl 3-maleimidopropionate | |
CAS RN |
55750-62-4 | |
Record name | Succinimidyl 3-maleimidopropionate | |
Source | CAS Common Chemistry | |
URL | https://commonchemistry.cas.org/detail?cas_rn=55750-62-4 | |
Description | CAS Common Chemistry is an open community resource for accessing chemical information. Nearly 500,000 chemical substances from CAS REGISTRY cover areas of community interest, including common and frequently regulated chemicals, and those relevant to high school and undergraduate chemistry classes. This chemical information, curated by our expert scientists, is provided in alignment with our mission as a division of the American Chemical Society. | |
Explanation | The data from CAS Common Chemistry is provided under a CC-BY-NC 4.0 license, unless otherwise stated. | |
Record name | 1-{3-[(2,5-Dioxopyrrolidin-1-yl)oxy]-3-oxopropyl}-1H-pyrrole-2,5-dione | |
Source | EPA DSSTox | |
URL | https://comptox.epa.gov/dashboard/DTXSID30404976 | |
Description | DSSTox provides a high quality public chemistry resource for supporting improved predictive toxicology. | |
Record name | 2,5-dioxopyrrolidin-1-yl 3-(2,5-dioxo-2,5-dihydro-1H-pyrrol-1-yl)propanoate | |
Source | European Chemicals Agency (ECHA) | |
URL | https://echa.europa.eu/information-on-chemicals | |
Description | The European Chemicals Agency (ECHA) is an agency of the European Union which is the driving force among regulatory authorities in implementing the EU's groundbreaking chemicals legislation for the benefit of human health and the environment as well as for innovation and competitiveness. | |
Explanation | Use of the information, documents and data from the ECHA website is subject to the terms and conditions of this Legal Notice, and subject to other binding limitations provided for under applicable law, the information, documents and data made available on the ECHA website may be reproduced, distributed and/or used, totally or in part, for non-commercial purposes provided that ECHA is acknowledged as the source: "Source: European Chemicals Agency, http://echa.europa.eu/". Such acknowledgement must be included in each copy of the material. ECHA permits and encourages organisations and individuals to create links to the ECHA website under the following cumulative conditions: Links can only be made to webpages that provide a link to the Legal Notice page. | |
Synthesis routes and methods I
Procedure details
Synthesis routes and methods II
Procedure details
Retrosynthesis Analysis
AI-Powered Synthesis Planning: Our tool employs the Template_relevance Pistachio, Template_relevance Bkms_metabolic, Template_relevance Pistachio_ringbreaker, Template_relevance Reaxys, Template_relevance Reaxys_biocatalysis model, leveraging a vast database of chemical reactions to predict feasible synthetic routes.
One-Step Synthesis Focus: Specifically designed for one-step synthesis, it provides concise and direct routes for your target compounds, streamlining the synthesis process.
Accurate Predictions: Utilizing the extensive PISTACHIO, BKMS_METABOLIC, PISTACHIO_RINGBREAKER, REAXYS, REAXYS_BIOCATALYSIS database, our tool offers high-accuracy predictions, reflecting the latest in chemical research and data.
Strategy Settings
Precursor scoring | Relevance Heuristic |
---|---|
Min. plausibility | 0.01 |
Model | Template_relevance |
Template Set | Pistachio/Bkms_metabolic/Pistachio_ringbreaker/Reaxys/Reaxys_biocatalysis |
Top-N result to add to graph | 6 |
Feasible Synthetic Routes
Q & A
Q1: How do Bone Morphogenetic Proteins (BMPs) exert their effects on cells?
A1: BMPs bind to specific receptors on the cell surface, known as BMP type I and type II receptors. [] This binding triggers a signaling cascade that involves the phosphorylation of downstream molecules called SMADs (specifically, SMAD1/5/8). [, ] These phosphorylated SMADs then translocate to the nucleus where they regulate the expression of target genes involved in various cellular processes, including cell differentiation, proliferation, and apoptosis. [, ]
Q2: Can you give an example of how BMP signaling is regulated in a specific tissue?
A2: In bone marrow, BMP-2 and BMP-4 play a crucial role in maintaining bone homeostasis by regulating the differentiation of both osteoblasts (bone-forming cells) and osteoclasts (bone-resorbing cells). [] The protein Noggin acts as an antagonist by binding to BMPs and preventing their interaction with receptors, thus modulating BMP signaling and subsequent bone remodeling. []
Q3: What are the implications of BMP heterodimer formation?
A3: BMPs can form homodimers (e.g., BMP-2/BMP-2) or heterodimers (e.g., BMP-2/BMP-7). Notably, heterodimers often exhibit significantly higher signaling activity compared to their homodimeric counterparts. [] For instance, BMP-2/7 heterodimers demonstrate a 20-fold higher specific activity in inducing alkaline phosphatase, a marker of osteoblast differentiation, compared to BMP homodimers. [] This enhanced activity is observed both in vitro and in vivo, highlighting the biological significance of heterodimer formation. []
Q4: Are there links between BMPs and specific disease states?
A4: Yes, dysregulation of BMP signaling has been implicated in various diseases. In ankylosing spondylitis, an inflammatory disease affecting the spine, serum levels of certain BMPs (BMP-2, BMP-4, BMP-6) are elevated and correlate with disease activity and radiological indices. [] Conversely, Dickkopf homologue-1 (Dkk-1), a BMP antagonist, is found at lower levels in these patients. [] These findings suggest that BMPs and their antagonists could serve as potential biomarkers or therapeutic targets for ankylosing spondylitis.
Q5: How do statin drugs, typically used for cholesterol management, interact with the BMP pathway in colorectal cancer?
A5: Studies show that statins can induce the expression of BMP-2 in certain colorectal cancer cell lines, leading to apoptosis (programmed cell death). [] This effect is dependent on the presence of SMAD4, a key component of the BMP signaling pathway. [] Interestingly, statin treatment may have adverse effects in colorectal cancers lacking SMAD4, highlighting the importance of understanding the molecular context for effective therapeutic targeting. []
Q6: How do BMPs contribute to embryonic development?
A6: BMPs play critical roles in dorsal-ventral patterning during embryonic development. [] In the developing chicken forebrain, ectopic expression of BMP-4 or BMP-5 disrupts normal patterning, leading to malformations such as holoprosencephaly (a single cerebral hemisphere) and cyclopia (a single midline eye). [] These findings underscore the importance of tightly regulated BMP signaling during critical developmental stages.
Haftungsausschluss und Informationen zu In-Vitro-Forschungsprodukten
Bitte beachten Sie, dass alle Artikel und Produktinformationen, die auf BenchChem präsentiert werden, ausschließlich zu Informationszwecken bestimmt sind. Die auf BenchChem zum Kauf angebotenen Produkte sind speziell für In-vitro-Studien konzipiert, die außerhalb lebender Organismen durchgeführt werden. In-vitro-Studien, abgeleitet von dem lateinischen Begriff "in Glas", beinhalten Experimente, die in kontrollierten Laborumgebungen unter Verwendung von Zellen oder Geweben durchgeführt werden. Es ist wichtig zu beachten, dass diese Produkte nicht als Arzneimittel oder Medikamente eingestuft sind und keine Zulassung der FDA für die Vorbeugung, Behandlung oder Heilung von medizinischen Zuständen, Beschwerden oder Krankheiten erhalten haben. Wir müssen betonen, dass jede Form der körperlichen Einführung dieser Produkte in Menschen oder Tiere gesetzlich strikt untersagt ist. Es ist unerlässlich, sich an diese Richtlinien zu halten, um die Einhaltung rechtlicher und ethischer Standards in Forschung und Experiment zu gewährleisten.