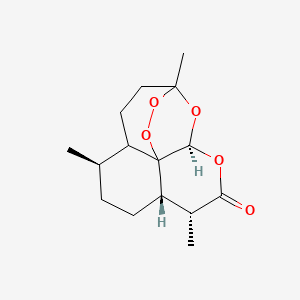
Artemisinin
Übersicht
Beschreibung
Es wurde 1972 von Tu Youyou entdeckt, die 2015 für diese Entdeckung den Nobelpreis für Physiologie oder Medizin erhielt . Artemisinin und seine Derivate sind bekannt für ihre potente antimalarielle Wirkung, insbesondere gegen Plasmodium falciparum, den Parasiten, der für die schwerste Form der Malaria verantwortlich ist .
Wissenschaftliche Forschungsanwendungen
Artemisinin and its derivatives have a wide range of scientific research applications:
Wirkmechanismus
Target of Action
Artemisinin, a sesquiterpene lactone isolated from the plant Artemisia annua, is primarily used in the treatment of malaria due to Plasmodium falciparum . The primary targets of this compound are the erythrocytic stages of P. falciparum . It has been suggested that this compound compounds may exert their functions via mechanisms like regulating key factors such as apoptosis-related BAX, FASL and caspase-3, multi-drug resistance genes, cytokines such as CD4+ and CD8+, inflammation-related NF-κB and COX2, telomerase, oxidative stress molecules, and so on . Two proteins related to heme catabolic pathway—heme detoxification protein (HDP) and histidine-rich protein II (HRP II)—have been posited as targets of this compound action .
Mode of Action
This compound and its derivatives are all sesquiterpene lactones containing an unusual peroxide bridge . This endoperoxide 1,2,4-trioxane ring is responsible for their antimalarial properties . The endoperoxide bond could be activated by reduced heme or ferrous iron , leading to the production of cytotoxic carbon-centered radicals, which are highly potent alkylating agents . These radicals may target essential parasite macromolecules, causing the parasite’s death .
Biochemical Pathways
The building blocks of this compound, isopentyl pyrophosphate (IPP) and dimethylallyl pyrophosphate (DMAPP), are synthesized from the mevalonate (MVA) and 2-C-methyl-D-erythritol-4-phosphate (MEP) pathways . Two genes of the this compound biosynthetic pathway have now been identified .
Pharmacokinetics
This compound and its derivatives are characterized by a short half-life . They are converted primarily, but to different extents, to the bioactive metabolite dihydrothis compound . The rate of conversion is lowest for artelinic acid and highest for the water-soluble artesunate . Oral bioavailability in animals ranges, approximately, between 19 and 35% . A first-pass effect is highly probable for all compounds when administered orally .
Result of Action
This compound and its derivatives have shown broad-spectrum antitumor activities in vitro and in vivo . The endoperoxide moiety of this compound reacts with the iron in cancer cells to produce reactive oxygen species (ROS) including superoxide and hydroxyl radicals which elicit cellular destruction . This compound was proved to reduce glomerular permeability and improve proteinuria in LN mice by inhibiting vascular endothelial growth factor (VEGF) .
Action Environment
Partial resistance to this compound (ART-R) is becoming a major threat to the efficacy of this compound-based combination therapies (ACTs) and intravenous artesunate . This situation has changed, with the emergence of ART-R in multiple countries in eastern Africa . The emergence of ART-R in Africa is an urgent concern, and it is essential that we increase efforts to characterize its spread and mitigate its impact .
Biochemische Analyse
Biochemical Properties
Artemisinin plays a crucial role in biochemical reactions, primarily through its interaction with heme and iron. The endoperoxide bridge in this compound is activated by reduced heme or ferrous iron, leading to the production of cytotoxic carbon-centered radicals . These radicals are highly potent alkylating agents that target essential macromolecules within parasites, leading to their death . This compound interacts with several enzymes and proteins, including apoptosis-related proteins such as BAX, FASL, and caspase-3, as well as multi-drug resistance genes and cytokines like CD4+ and CD8+ . Additionally, this compound affects inflammation-related molecules such as NF-κB and COX2, telomerase, and oxidative stress molecules .
Cellular Effects
This compound exerts significant effects on various types of cells and cellular processes. It has been shown to regulate the expression of proinflammatory and anti-inflammatory cytokines, influencing the activation and frequency of T helper and B cells, macrophages, dendritic cells, neutrophils, mast cells, and myeloid-derived suppressor cells . This compound also affects cell signaling pathways, gene expression, and cellular metabolism. For instance, it can induce apoptosis, cause cell cycle arrest, and disrupt cancer invasion and metastasis . Furthermore, this compound influences the tumor microenvironment and mediates tumor-related signaling pathways .
Molecular Mechanism
The molecular mechanism of this compound involves the activation of its endoperoxide bridge by heme or ferrous iron, resulting in the generation of free radicals . These radicals damage susceptible proteins and macromolecules within parasites, leading to their death . This compound also regulates key factors such as apoptosis-related proteins (BAX, FASL, caspase-3), multi-drug resistance genes, cytokines (CD4+, CD8+), inflammation-related molecules (NF-κB, COX2), telomerase, and oxidative stress molecules . Additionally, this compound can inhibit or activate enzymes, alter gene expression, and disrupt cellular signaling pathways .
Temporal Effects in Laboratory Settings
In laboratory settings, the effects of this compound can change over time. This compound and its derivatives have demonstrated stability and efficacy in various in vitro and in vivo studies . The long-term effects on cellular function and potential degradation of the compound are still being investigated. Studies have shown that this compound can maintain its antimalarial activity over extended periods, but its stability and degradation in different environments need further exploration .
Dosage Effects in Animal Models
The effects of this compound vary with different dosages in animal models. At therapeutic doses, this compound has shown efficacy in treating malaria and other diseases without significant adverse effects . At high doses, this compound can induce toxicity and adverse effects, including fetal death and malformations in rodents during early embryogenesis . The therapeutic window and threshold effects of this compound need to be carefully considered in clinical applications .
Metabolic Pathways
This compound is involved in several metabolic pathways, including the mevalonate pathway and the methylerythritol phosphate pathway . These pathways produce isopentenyl diphosphate and dimethylallyl diphosphate, which are converted into farnesyl pyrophosphate by farnesyl pyrophosphate synthase . Farnesyl pyrophosphate is a key intermediate in the biosynthesis of this compound . This compound also interacts with various enzymes and cofactors, affecting metabolic flux and metabolite levels .
Transport and Distribution
This compound is transported and distributed within cells and tissues through various mechanisms. It interacts with transporters and binding proteins that facilitate its localization and accumulation . This compound and its derivatives exhibit variations in distribution based on species and developmental stages . The compound’s transport and distribution are influenced by factors such as leaf age, morphology, and trichome distribution .
Subcellular Localization
This compound’s subcellular localization plays a crucial role in its activity and function. Studies have shown that this compound accumulates in the endoplasmic reticulum and vesicles involved in intracellular trafficking . It also localizes near the mitochondria upon treatment with dihydrothis compound . The subcellular localization of this compound is influenced by targeting signals and post-translational modifications that direct it to specific compartments or organelles .
Vorbereitungsmethoden
Synthetische Wege und Reaktionsbedingungen
Artemisinin kann durch verschiedene Verfahren synthetisiert werden, darunter die Extraktion aus Artemisia annua und die Verwendung gentechnisch veränderter Hefe. Der Extraktionsprozess beinhaltet die Isolierung der Verbindung aus den getrockneten Blättern oder Blütenständen der Pflanze . Der synthetische Weg unter Verwendung gentechnisch veränderter Hefe beinhaltet die Biosynthese von Artemisininsäure, die dann chemisch zu this compound umgewandelt wird .
Industrielle Produktionsverfahren
Die industrielle Produktion von this compound umfasst typischerweise den Anbau von Artemisia annua, gefolgt von Extraktions- und Reinigungsprozessen. Die Extraktion erfolgt häufig unter Verwendung von Lösungsmitteln wie Hexan oder Petrolether, und die Reinigung wird durch Umkristallisation erreicht . Fortschritte in der Biotechnologie haben auch die Produktion von this compound durch mikrobielle Fermentation ermöglicht, was einen nachhaltigeren und skalierbareren Ansatz bietet .
Chemische Reaktionsanalyse
Arten von Reaktionen
This compound durchläuft verschiedene chemische Reaktionen, darunter Oxidation, Reduktion und Substitution. Die bemerkenswerteste Reaktion ist die Spaltung seiner Endoperoxidbrücke, die für seine antimalarielle Aktivität entscheidend ist .
Häufige Reagenzien und Bedingungen
Oxidation: this compound kann mit Reagenzien wie Wasserstoffperoxid oder m-Chlorperbenzoesäure oxidiert werden.
Reduktion: Die Reduktion von this compound kann mit Natriumborhydrid oder Lithiumaluminiumhydrid erreicht werden.
Substitution: Substitutionsreaktionen beinhalten oft Nukleophile wie Amine oder Thiole.
Hauptprodukte
Die Hauptprodukte, die aus diesen Reaktionen entstehen, sind Dihydrothis compound, Artemether und Artesunat. Diese Derivate behalten die antimalariellen Eigenschaften von this compound bei und werden in Kombinationstherapien eingesetzt .
Wissenschaftliche Forschungsanwendungen
This compound und seine Derivate haben eine breite Palette von wissenschaftlichen Forschungsanwendungen:
Medizin: This compound wird hauptsächlich zur Behandlung von Malaria eingesetzt, wird aber auch auf sein Potenzial zur Behandlung anderer parasitärer Infektionen, Krebs und Autoimmunerkrankungen untersucht
Wirkmechanismus
Der Wirkmechanismus von this compound beinhaltet die Spaltung seiner Endoperoxidbrücke durch Eisenionen in den Zellen des Parasiten. Diese Reaktion erzeugt reaktive Sauerstoffspezies, die wichtige Proteine und Membranen schädigen und zum Tod des Parasiten führen . This compound zielt auf das Schizont-Stadium des Lebenszyklus des Parasiten ab, das für seine schnellen antimalariellen Wirkungen entscheidend ist .
Analyse Chemischer Reaktionen
Types of Reactions
Artemisinin undergoes various chemical reactions, including oxidation, reduction, and substitution. The most notable reaction is the cleavage of its endoperoxide bridge, which is crucial for its antimalarial activity .
Common Reagents and Conditions
Oxidation: this compound can be oxidized using reagents such as hydrogen peroxide or m-chloroperbenzoic acid.
Reduction: Reduction of this compound can be achieved using sodium borohydride or lithium aluminum hydride.
Substitution: Substitution reactions often involve nucleophiles such as amines or thiols.
Major Products
The major products formed from these reactions include dihydrothis compound, artemether, and artesunate. These derivatives retain the antimalarial properties of this compound and are used in combination therapies .
Vergleich Mit ähnlichen Verbindungen
Ähnliche Verbindungen
- Dihydroartemisinin
- Artemether
- Artesunat
- Arteether
Einzigartigkeit
This compound ist aufgrund seiner Endoperoxidbrücke einzigartig, die für seine antimalarielle Aktivität unerlässlich ist. Dieses strukturelle Merkmal ist in anderen Naturstoffen nicht üblich, wodurch this compound und seine Derivate besonders wirksam gegen Malaria sind . Darüber hinaus hat this compound ein breiteres Wirkungsspektrum und eine schnellere Parasitenclearance im Vergleich zu anderen antimalariellen Medikamenten .
Eigenschaften
CAS-Nummer |
63968-64-9 |
---|---|
Molekularformel |
C15H22O5 |
Molekulargewicht |
282.33 g/mol |
IUPAC-Name |
(1R,13R)-1,5,9-trimethyl-11,14,15,16-tetraoxatetracyclo[10.3.1.04,13.08,13]hexadecan-10-one |
InChI |
InChI=1S/C15H22O5/c1-8-4-5-11-9(2)12(16)17-13-15(11)10(8)6-7-14(3,18-13)19-20-15/h8-11,13H,4-7H2,1-3H3/t8?,9?,10?,11?,13?,14-,15-/m1/s1 |
InChI-Schlüssel |
BLUAFEHZUWYNDE-WJRFXBFBSA-N |
SMILES |
CC1CCC2C(C(=O)OC3C24C1CCC(O3)(OO4)C)C |
Isomerische SMILES |
CC1CCC2C(C(=O)OC3[C@@]24C1CC[C@](O3)(OO4)C)C |
Kanonische SMILES |
CC1CCC2C(C(=O)OC3C24C1CCC(O3)(OO4)C)C |
Aussehen |
Solid powder |
Key on ui other cas no. |
63968-64-9 |
Piktogramme |
Flammable; Environmental Hazard |
Reinheit |
>98% (or refer to the Certificate of Analysis) |
Haltbarkeit |
>2 years if stored properly |
Löslichkeit |
Soluble in DMSO, not in water |
Lagerung |
Dry, dark and at 0 - 4 C for short term (days to weeks) or -20 C for long term (months to years). |
Synonyme |
arteannuin artemisinin artemisinine qinghaosu quing hau sau quinghaosu |
Herkunft des Produkts |
United States |
Retrosynthesis Analysis
AI-Powered Synthesis Planning: Our tool employs the Template_relevance Pistachio, Template_relevance Bkms_metabolic, Template_relevance Pistachio_ringbreaker, Template_relevance Reaxys, Template_relevance Reaxys_biocatalysis model, leveraging a vast database of chemical reactions to predict feasible synthetic routes.
One-Step Synthesis Focus: Specifically designed for one-step synthesis, it provides concise and direct routes for your target compounds, streamlining the synthesis process.
Accurate Predictions: Utilizing the extensive PISTACHIO, BKMS_METABOLIC, PISTACHIO_RINGBREAKER, REAXYS, REAXYS_BIOCATALYSIS database, our tool offers high-accuracy predictions, reflecting the latest in chemical research and data.
Strategy Settings
Precursor scoring | Relevance Heuristic |
---|---|
Min. plausibility | 0.01 |
Model | Template_relevance |
Template Set | Pistachio/Bkms_metabolic/Pistachio_ringbreaker/Reaxys/Reaxys_biocatalysis |
Top-N result to add to graph | 6 |
Feasible Synthetic Routes
Haftungsausschluss und Informationen zu In-Vitro-Forschungsprodukten
Bitte beachten Sie, dass alle Artikel und Produktinformationen, die auf BenchChem präsentiert werden, ausschließlich zu Informationszwecken bestimmt sind. Die auf BenchChem zum Kauf angebotenen Produkte sind speziell für In-vitro-Studien konzipiert, die außerhalb lebender Organismen durchgeführt werden. In-vitro-Studien, abgeleitet von dem lateinischen Begriff "in Glas", beinhalten Experimente, die in kontrollierten Laborumgebungen unter Verwendung von Zellen oder Geweben durchgeführt werden. Es ist wichtig zu beachten, dass diese Produkte nicht als Arzneimittel oder Medikamente eingestuft sind und keine Zulassung der FDA für die Vorbeugung, Behandlung oder Heilung von medizinischen Zuständen, Beschwerden oder Krankheiten erhalten haben. Wir müssen betonen, dass jede Form der körperlichen Einführung dieser Produkte in Menschen oder Tiere gesetzlich strikt untersagt ist. Es ist unerlässlich, sich an diese Richtlinien zu halten, um die Einhaltung rechtlicher und ethischer Standards in Forschung und Experiment zu gewährleisten.