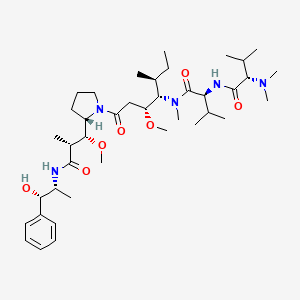
Auristatin E
Übersicht
Beschreibung
Auristatin E is a synthetic derivative of dolastatin 10, a peptide originally isolated from the marine mollusk Dolabella auricularia. It is a potent antimitotic agent that inhibits cell division by blocking the polymerization of tubulin, a protein essential for cell division . Due to its high cytotoxicity, this compound is primarily used in the form of antibody-drug conjugates for targeted cancer therapy .
Vorbereitungsmethoden
Synthetic Routes and Reaction Conditions: Auristatin E is synthesized through a series of peptide coupling reactions. The synthesis involves the coupling of various amino acid derivatives under controlled conditions to form the peptide backbone. The key steps include:
- Protection and deprotection of functional groups to ensure selective reactions.
- Coupling reactions using reagents such as dicyclohexylcarbodiimide and N-hydroxysuccinimide.
- Purification of the final product using techniques like high-performance liquid chromatography to achieve high purity .
Industrial Production Methods: Industrial production of this compound involves optimizing the synthetic route to increase yield and purity. This includes:
- Scaling up the reaction conditions while maintaining the integrity of the product.
- Implementing robust purification processes to remove impurities and degradation products.
- Ensuring the stability of the compound during storage and transportation .
Analyse Chemischer Reaktionen
Types of Reactions: Auristatin E undergoes various chemical reactions, including:
Oxidation: this compound can be oxidized under specific conditions to form oxidized derivatives.
Reduction: Reduction reactions can modify the functional groups on this compound, altering its activity.
Substitution: Substitution reactions can introduce different functional groups, potentially enhancing its properties.
Common Reagents and Conditions:
Oxidation: Reagents like hydrogen peroxide or potassium permanganate under controlled conditions.
Reduction: Reagents such as sodium borohydride or lithium aluminum hydride.
Substitution: Various nucleophiles or electrophiles depending on the desired modification.
Major Products Formed:
- Oxidized this compound derivatives.
- Reduced this compound with modified functional groups.
- Substituted this compound with enhanced or altered properties .
Wissenschaftliche Forschungsanwendungen
Antibody-Drug Conjugates (ADCs)
MMAE is a key component in several FDA-approved ADCs, including Adcetris® (brentuximab vedotin) and Polivy® (polatuzumab vedotin). These ADCs utilize monoclonal antibodies to target specific antigens on cancer cells, ensuring that MMAE is delivered directly to the tumor site. This targeted approach enhances therapeutic efficacy while minimizing damage to healthy tissues .
Table 1: FDA-Approved ADCs Containing MMAE
ADC Name | Target Antigen | Indication | Approval Year |
---|---|---|---|
Adcetris® | CD30 | Hodgkin lymphoma | 2011 |
Polivy® | CD79b | Diffuse large B-cell lymphoma | 2019 |
Clinical Applications and Trials
Recent studies have demonstrated the effectiveness of MMAE in various cancer types through ADC formulations:
- Hodgkin Lymphoma : The combination of MMAE with the anti-CD30 antibody has shown significant antitumor activity in clinical settings, leading to improved patient outcomes .
- Non-Hodgkin Lymphoma : ADCs utilizing MMAE have been evaluated in multiple clinical trials, showing promising results in terms of response rates and overall survival .
Case Study: cAC10-vcMMAE
The chimeric monoclonal antibody cAC10 conjugated with MMAE has been studied extensively. In preclinical models, it demonstrated strong antitumor activity against CD30+ cell lines and induced apoptosis through G2/M-phase growth arrest . This case exemplifies the potential of MMAE in enhancing the efficacy of targeted therapies.
Innovative Delivery Systems
Recent advancements have focused on improving the delivery mechanisms of MMAE:
- Light-Activated Prodrug Nanoparticles : Research has explored nanoparticles that release MMAE upon light activation, providing a novel approach for treating pancreatic cancer with reduced systemic toxicity. This method combines photodynamic therapy with chemotherapy to enhance tumor targeting .
- Integrin-Targeted Peptide-Drug Conjugates : Studies have developed peptide-drug conjugates that selectively target integrin αvβ6, a receptor implicated in tumor progression. These conjugates showed high stability and cytotoxicity against integrin-positive tumors, demonstrating the versatility of MMAE in various therapeutic contexts .
Ongoing Research and Future Directions
The landscape of MMAE applications continues to evolve with ongoing research into new ADC formulations and combination therapies. Current investigations are focusing on:
- Enhancing the specificity and efficacy of MMAE through novel linkers and conjugation strategies.
- Exploring combination therapies that integrate MMAE with immunotherapies to improve patient outcomes.
- Evaluating the pharmacokinetics and biodistribution of MMAE across different species to better translate preclinical findings to clinical settings .
Wirkmechanismus
Auristatin E exerts its effects by inhibiting the polymerization of tubulin, a protein essential for the formation of microtubules during cell division. This inhibition leads to cell cycle arrest and apoptosis (programmed cell death) in rapidly dividing cells, such as cancer cells . The compound is often linked to monoclonal antibodies that specifically target cancer cells, ensuring selective delivery and minimizing toxicity to healthy cells .
Vergleich Mit ähnlichen Verbindungen
Monomethyl Auristatin E: A derivative of this compound with a single methyl group, used in antibody-drug conjugates for targeted cancer therapy.
Monomethyl Auristatin F: Another derivative with a different functional group, offering distinct pharmacokinetic properties and reduced bystander effects.
Uniqueness of this compound: this compound is unique due to its high potency and ability to be modified for various applications. Its derivatives, such as monomethyl this compound and monomethyl auristatin F, provide additional options for targeted cancer therapy, each with specific advantages and limitations .
Biologische Aktivität
Overview
Auristatin E, particularly in its monomethyl form (MMAE), is a potent antimitotic agent derived from the natural product Dolastatin 10. It exhibits significant cytotoxic properties by inhibiting tubulin polymerization, thereby blocking cell division. This compound is primarily utilized in antibody-drug conjugates (ADCs) for targeted cancer therapy, enhancing the therapeutic index while minimizing systemic toxicity.
- Chemical Name : (S)-N-((3R,4S,5S)-1-((S)-2-((1R,2R)-3-(((1S,2R)-1-hydroxy-1-phenylpropan-2-yl)amino)-1-methoxy-2-methyl-3-oxopropyl)pyrrolidin-1-yl)-3-methoxy-5-methyl-1-oxoheptan-4-yl)-N,3-dimethyl-2-((S)-3-methyl-2-(methylamino)butanamido)butanamide
- Molecular Weight : 717.98 g/mol
- Formula : C39H67N5O7
- CAS Number : 474645-27-7
- Solubility : Soluble in DMSO up to 20 mM
This compound functions by disrupting microtubule dynamics:
- Inhibition of Tubulin Polymerization : MMAE binds to tubulin, preventing its polymerization into microtubules, which are essential for mitosis.
- Targeted Delivery via ADCs : MMAE is conjugated to monoclonal antibodies that target specific antigens on cancer cells. Once the ADC binds to the target cell and is internalized, MMAE is released intracellularly, exerting its cytotoxic effects.
Clinical Applications
MMAE has been incorporated into several ADCs, demonstrating efficacy in various cancers. Notable ADCs include:
- Brentuximab Vedotin : Targets CD30-positive malignancies.
- DLYE5953A : An anti-LY6E antibody linked to MMAE showed promising results in a Phase I study with partial responses observed in metastatic solid tumors .
Efficacy and Safety Profiles
- Phase I Study of DLYE5953A :
- Combination with Radiotherapy :
Comparative Efficacy
ADC Name | Target | Response Rate | Notable Adverse Effects |
---|---|---|---|
Brentuximab Vedotin | CD30 | ~75% | Peripheral neuropathy |
DLYE5953A | LY6E | 12% | Neutropenia |
BT5528 | EphA2 | TBD | TBD |
BT8009 | Nectin-4 | TBD | TBD |
Eigenschaften
IUPAC Name |
(2S)-2-[[(2S)-2-(dimethylamino)-3-methylbutanoyl]amino]-N-[(3R,4S,5S)-1-[(2S)-2-[(1R,2R)-3-[[(1S,2R)-1-hydroxy-1-phenylpropan-2-yl]amino]-1-methoxy-2-methyl-3-oxopropyl]pyrrolidin-1-yl]-3-methoxy-5-methyl-1-oxoheptan-4-yl]-N,3-dimethylbutanamide | |
---|---|---|
Source | PubChem | |
URL | https://pubchem.ncbi.nlm.nih.gov | |
Description | Data deposited in or computed by PubChem | |
InChI |
InChI=1S/C40H69N5O7/c1-14-26(6)35(44(11)40(50)33(24(2)3)42-39(49)34(25(4)5)43(9)10)31(51-12)23-32(46)45-22-18-21-30(45)37(52-13)27(7)38(48)41-28(8)36(47)29-19-16-15-17-20-29/h15-17,19-20,24-28,30-31,33-37,47H,14,18,21-23H2,1-13H3,(H,41,48)(H,42,49)/t26-,27+,28+,30-,31+,33-,34-,35-,36+,37+/m0/s1 | |
Source | PubChem | |
URL | https://pubchem.ncbi.nlm.nih.gov | |
Description | Data deposited in or computed by PubChem | |
InChI Key |
WOWDZACBATWTAU-FEFUEGSOSA-N | |
Source | PubChem | |
URL | https://pubchem.ncbi.nlm.nih.gov | |
Description | Data deposited in or computed by PubChem | |
Canonical SMILES |
CCC(C)C(C(CC(=O)N1CCCC1C(C(C)C(=O)NC(C)C(C2=CC=CC=C2)O)OC)OC)N(C)C(=O)C(C(C)C)NC(=O)C(C(C)C)N(C)C | |
Source | PubChem | |
URL | https://pubchem.ncbi.nlm.nih.gov | |
Description | Data deposited in or computed by PubChem | |
Isomeric SMILES |
CC[C@H](C)[C@@H]([C@@H](CC(=O)N1CCC[C@H]1[C@@H]([C@@H](C)C(=O)N[C@H](C)[C@H](C2=CC=CC=C2)O)OC)OC)N(C)C(=O)[C@H](C(C)C)NC(=O)[C@H](C(C)C)N(C)C | |
Source | PubChem | |
URL | https://pubchem.ncbi.nlm.nih.gov | |
Description | Data deposited in or computed by PubChem | |
Molecular Formula |
C40H69N5O7 | |
Source | PubChem | |
URL | https://pubchem.ncbi.nlm.nih.gov | |
Description | Data deposited in or computed by PubChem | |
Molecular Weight |
732.0 g/mol | |
Source | PubChem | |
URL | https://pubchem.ncbi.nlm.nih.gov | |
Description | Data deposited in or computed by PubChem | |
CAS No. |
160800-57-7 | |
Record name | L-Valinamide, N,N-dimethyl-L-valyl-N-[(1S,2R)-4-[(2S)-2-[(1R,2R)-3-[[(1R,2S)-2-hydro xy-1-methyl- | |
Source | European Chemicals Agency (ECHA) | |
URL | https://echa.europa.eu/information-on-chemicals | |
Description | The European Chemicals Agency (ECHA) is an agency of the European Union which is the driving force among regulatory authorities in implementing the EU's groundbreaking chemicals legislation for the benefit of human health and the environment as well as for innovation and competitiveness. | |
Explanation | Use of the information, documents and data from the ECHA website is subject to the terms and conditions of this Legal Notice, and subject to other binding limitations provided for under applicable law, the information, documents and data made available on the ECHA website may be reproduced, distributed and/or used, totally or in part, for non-commercial purposes provided that ECHA is acknowledged as the source: "Source: European Chemicals Agency, http://echa.europa.eu/". Such acknowledgement must be included in each copy of the material. ECHA permits and encourages organisations and individuals to create links to the ECHA website under the following cumulative conditions: Links can only be made to webpages that provide a link to the Legal Notice page. | |
Retrosynthesis Analysis
AI-Powered Synthesis Planning: Our tool employs the Template_relevance Pistachio, Template_relevance Bkms_metabolic, Template_relevance Pistachio_ringbreaker, Template_relevance Reaxys, Template_relevance Reaxys_biocatalysis model, leveraging a vast database of chemical reactions to predict feasible synthetic routes.
One-Step Synthesis Focus: Specifically designed for one-step synthesis, it provides concise and direct routes for your target compounds, streamlining the synthesis process.
Accurate Predictions: Utilizing the extensive PISTACHIO, BKMS_METABOLIC, PISTACHIO_RINGBREAKER, REAXYS, REAXYS_BIOCATALYSIS database, our tool offers high-accuracy predictions, reflecting the latest in chemical research and data.
Strategy Settings
Precursor scoring | Relevance Heuristic |
---|---|
Min. plausibility | 0.01 |
Model | Template_relevance |
Template Set | Pistachio/Bkms_metabolic/Pistachio_ringbreaker/Reaxys/Reaxys_biocatalysis |
Top-N result to add to graph | 6 |
Feasible Synthetic Routes
Haftungsausschluss und Informationen zu In-Vitro-Forschungsprodukten
Bitte beachten Sie, dass alle Artikel und Produktinformationen, die auf BenchChem präsentiert werden, ausschließlich zu Informationszwecken bestimmt sind. Die auf BenchChem zum Kauf angebotenen Produkte sind speziell für In-vitro-Studien konzipiert, die außerhalb lebender Organismen durchgeführt werden. In-vitro-Studien, abgeleitet von dem lateinischen Begriff "in Glas", beinhalten Experimente, die in kontrollierten Laborumgebungen unter Verwendung von Zellen oder Geweben durchgeführt werden. Es ist wichtig zu beachten, dass diese Produkte nicht als Arzneimittel oder Medikamente eingestuft sind und keine Zulassung der FDA für die Vorbeugung, Behandlung oder Heilung von medizinischen Zuständen, Beschwerden oder Krankheiten erhalten haben. Wir müssen betonen, dass jede Form der körperlichen Einführung dieser Produkte in Menschen oder Tiere gesetzlich strikt untersagt ist. Es ist unerlässlich, sich an diese Richtlinien zu halten, um die Einhaltung rechtlicher und ethischer Standards in Forschung und Experiment zu gewährleisten.