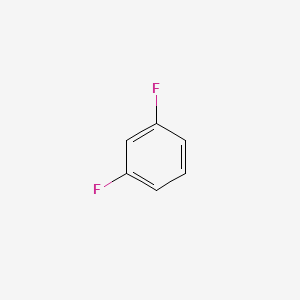
1,3-Difluorobenzene
Übersicht
Beschreibung
1,3-Difluorobenzene, also known as m-difluorobenzene, is a clear colorless to yellowish liquid . It has the molecular formula C6H4F2 and a molecular weight of 114.0928 . It is stable under normal temperatures and pressures .
Synthesis Analysis
1,3-Difluorobenzene can be synthesized from m-phenylenediamine through a process called double diazotization . This process is exothermic and the diazonium intermediates are extremely unstable, which may lead to undesired coupling products and decomposition .
Molecular Structure Analysis
The molecular structure of 1,3-Difluorobenzene consists of a benzene ring with two fluorine atoms attached at the 1 and 3 positions . The IUPAC Standard InChIKey for 1,3-Difluorobenzene is UEMGWPRHOOEKTA-UHFFFAOYSA-N .
Physical And Chemical Properties Analysis
1,3-Difluorobenzene is a liquid at room temperature with a boiling point of 83 °C and a melting point of -59 °C . It has a density of 1.163 g/mL at 25 °C . It is insoluble in water .
Wissenschaftliche Forschungsanwendungen
Pharmaceutical Synthesis
1,3-Difluorobenzene is utilized as an intermediate in the synthesis of various fluorinated medicines. Notable examples include antifungal agents like Fluconazole and other pharmaceuticals such as Diflunisal and Enoxacin .
Pesticide Production
This compound plays a role in the creation of pesticides, including Flucycloxuron and Diflubenzuro, serving as a crucial intermediate in their chemical synthesis .
Liquid Crystal Materials
The synthesis of liquid crystal materials, which are essential components in displays and other electronic devices, often involves 1,3-Difluorobenzene as a key ingredient .
Electrochemical Analysis
1,3-Difluorobenzene is used as a solvent in the electrochemical analysis of transition metal complexes due to its chemical inertness and high dielectric constant .
Calorimetric Studies
Low-temperature calorimetric studies utilize 1,3-Difluorobenzene to understand its chemical thermodynamic properties better .
Stabilizing Electrode Interfaces
In battery technology, specifically within lithium-ion batteries, 1,3-Difluorobenzene is added as a diluent to stabilize the electrode interface, which can improve the battery’s performance and longevity .
Wirkmechanismus
. The primary targets of 1,3-Difluorobenzene are not well-defined due to the lack of specific studies on this compound. .
Mode of Action
The specific interactions of 1,3-Difluorobenzene with its targets would depend on the specific context of its use, particularly in the synthesis of other compounds .
Biochemical Pathways
The downstream effects of these reactions would depend on the specific context of the compound’s use .
Pharmacokinetics
It is known that the compound is a liquid at room temperature , which could influence its absorption and distribution. The metabolism and excretion of the compound would likely depend on the specific biological system in which it is used.
Result of Action
As a fluorinated compound, it could potentially influence a variety of molecular and cellular processes due to the unique properties of fluorine .
Action Environment
The action, efficacy, and stability of 1,3-Difluorobenzene can be influenced by various environmental factors. For instance, it should be handled in a well-ventilated area and kept away from heat, sparks, open flames, and hot surfaces . The specific effects of these environmental factors would depend on the context of the compound’s use.
Safety and Hazards
1,3-Difluorobenzene is highly flammable and poses a severe fire hazard when exposed to heat, flame, and/or oxidizers . It is toxic and may emit toxic fumes of carbon monoxide (CO) when combusted . It is advised to avoid breathing its dust, fume, gas, mist, vapors, or spray, and to use personal protective equipment when handling it .
Zukünftige Richtungen
Eigenschaften
IUPAC Name |
1,3-difluorobenzene | |
---|---|---|
Source | PubChem | |
URL | https://pubchem.ncbi.nlm.nih.gov | |
Description | Data deposited in or computed by PubChem | |
InChI |
InChI=1S/C6H4F2/c7-5-2-1-3-6(8)4-5/h1-4H | |
Source | PubChem | |
URL | https://pubchem.ncbi.nlm.nih.gov | |
Description | Data deposited in or computed by PubChem | |
InChI Key |
UEMGWPRHOOEKTA-UHFFFAOYSA-N | |
Source | PubChem | |
URL | https://pubchem.ncbi.nlm.nih.gov | |
Description | Data deposited in or computed by PubChem | |
Canonical SMILES |
C1=CC(=CC(=C1)F)F | |
Source | PubChem | |
URL | https://pubchem.ncbi.nlm.nih.gov | |
Description | Data deposited in or computed by PubChem | |
Molecular Formula |
C6H4F2 | |
Source | PubChem | |
URL | https://pubchem.ncbi.nlm.nih.gov | |
Description | Data deposited in or computed by PubChem | |
DSSTOX Substance ID |
DTXSID50190682 | |
Record name | 1,3-Difluorobenzene | |
Source | EPA DSSTox | |
URL | https://comptox.epa.gov/dashboard/DTXSID50190682 | |
Description | DSSTox provides a high quality public chemistry resource for supporting improved predictive toxicology. | |
Molecular Weight |
114.09 g/mol | |
Source | PubChem | |
URL | https://pubchem.ncbi.nlm.nih.gov | |
Description | Data deposited in or computed by PubChem | |
CAS RN |
372-18-9 | |
Record name | 1,3-Difluorobenzene | |
Source | CAS Common Chemistry | |
URL | https://commonchemistry.cas.org/detail?cas_rn=372-18-9 | |
Description | CAS Common Chemistry is an open community resource for accessing chemical information. Nearly 500,000 chemical substances from CAS REGISTRY cover areas of community interest, including common and frequently regulated chemicals, and those relevant to high school and undergraduate chemistry classes. This chemical information, curated by our expert scientists, is provided in alignment with our mission as a division of the American Chemical Society. | |
Explanation | The data from CAS Common Chemistry is provided under a CC-BY-NC 4.0 license, unless otherwise stated. | |
Record name | 1,3-Difluorobenzene | |
Source | ChemIDplus | |
URL | https://pubchem.ncbi.nlm.nih.gov/substance/?source=chemidplus&sourceid=0000372189 | |
Description | ChemIDplus is a free, web search system that provides access to the structure and nomenclature authority files used for the identification of chemical substances cited in National Library of Medicine (NLM) databases, including the TOXNET system. | |
Record name | 1,3-DIFLUOROBENZENE | |
Source | DTP/NCI | |
URL | https://dtp.cancer.gov/dtpstandard/servlet/dwindex?searchtype=NSC&outputformat=html&searchlist=10285 | |
Description | The NCI Development Therapeutics Program (DTP) provides services and resources to the academic and private-sector research communities worldwide to facilitate the discovery and development of new cancer therapeutic agents. | |
Explanation | Unless otherwise indicated, all text within NCI products is free of copyright and may be reused without our permission. Credit the National Cancer Institute as the source. | |
Record name | 1,3-Difluorobenzene | |
Source | EPA DSSTox | |
URL | https://comptox.epa.gov/dashboard/DTXSID50190682 | |
Description | DSSTox provides a high quality public chemistry resource for supporting improved predictive toxicology. | |
Record name | 1,3-difluorobenzene | |
Source | European Chemicals Agency (ECHA) | |
URL | https://echa.europa.eu/substance-information/-/substanceinfo/100.006.133 | |
Description | The European Chemicals Agency (ECHA) is an agency of the European Union which is the driving force among regulatory authorities in implementing the EU's groundbreaking chemicals legislation for the benefit of human health and the environment as well as for innovation and competitiveness. | |
Explanation | Use of the information, documents and data from the ECHA website is subject to the terms and conditions of this Legal Notice, and subject to other binding limitations provided for under applicable law, the information, documents and data made available on the ECHA website may be reproduced, distributed and/or used, totally or in part, for non-commercial purposes provided that ECHA is acknowledged as the source: "Source: European Chemicals Agency, http://echa.europa.eu/". Such acknowledgement must be included in each copy of the material. ECHA permits and encourages organisations and individuals to create links to the ECHA website under the following cumulative conditions: Links can only be made to webpages that provide a link to the Legal Notice page. | |
Record name | M-DIFLUOROBENZENE | |
Source | FDA Global Substance Registration System (GSRS) | |
URL | https://gsrs.ncats.nih.gov/ginas/app/beta/substances/VO3C26WOC2 | |
Description | The FDA Global Substance Registration System (GSRS) enables the efficient and accurate exchange of information on what substances are in regulated products. Instead of relying on names, which vary across regulatory domains, countries, and regions, the GSRS knowledge base makes it possible for substances to be defined by standardized, scientific descriptions. | |
Explanation | Unless otherwise noted, the contents of the FDA website (www.fda.gov), both text and graphics, are not copyrighted. They are in the public domain and may be republished, reprinted and otherwise used freely by anyone without the need to obtain permission from FDA. Credit to the U.S. Food and Drug Administration as the source is appreciated but not required. | |
Synthesis routes and methods I
Procedure details
Synthesis routes and methods II
Procedure details
Synthesis routes and methods III
Procedure details
Retrosynthesis Analysis
AI-Powered Synthesis Planning: Our tool employs the Template_relevance Pistachio, Template_relevance Bkms_metabolic, Template_relevance Pistachio_ringbreaker, Template_relevance Reaxys, Template_relevance Reaxys_biocatalysis model, leveraging a vast database of chemical reactions to predict feasible synthetic routes.
One-Step Synthesis Focus: Specifically designed for one-step synthesis, it provides concise and direct routes for your target compounds, streamlining the synthesis process.
Accurate Predictions: Utilizing the extensive PISTACHIO, BKMS_METABOLIC, PISTACHIO_RINGBREAKER, REAXYS, REAXYS_BIOCATALYSIS database, our tool offers high-accuracy predictions, reflecting the latest in chemical research and data.
Strategy Settings
Precursor scoring | Relevance Heuristic |
---|---|
Min. plausibility | 0.01 |
Model | Template_relevance |
Template Set | Pistachio/Bkms_metabolic/Pistachio_ringbreaker/Reaxys/Reaxys_biocatalysis |
Top-N result to add to graph | 6 |
Feasible Synthetic Routes
Q & A
Q1: What is the molecular formula and weight of 1,3-difluorobenzene?
A1: The molecular formula of 1,3-difluorobenzene is C6H4F2. Its molecular weight is 114.09 g/mol. []
Q2: What spectroscopic data is available for characterizing 1,3-difluorobenzene?
A2: Several spectroscopic techniques are helpful in characterizing 1,3-difluorobenzene. These include:
- Nuclear Magnetic Resonance (NMR) Spectroscopy: Both 1H and 19F NMR provide valuable information about the structure and environment of the hydrogen and fluorine atoms within the molecule. []
- Microwave Spectroscopy: This technique has been used to determine the bond lengths and angles within the molecule, offering insights into the structural distortion of the benzene ring upon fluorination. [, ]
- Infrared (IR) and Raman Spectroscopy: These techniques provide information about the vibrational modes of the molecule, further aiding in structural characterization. []
Q3: What are common synthetic approaches for 1,3-difluorobenzene?
A3: 1,3-Difluorobenzene can be synthesized through various methods:
- Schiemann Reaction: This classic approach involves the diazotization of 1,3-phenylenediamine followed by thermal decomposition of the resulting diazonium salt in the presence of fluoroboric acid. []
- Halogen Exchange: Starting from 1,3-dichlorobenzene, a nucleophilic aromatic substitution using potassium fluoride can be employed to replace the chlorine atoms with fluorine. [, ]
- Deamination of 2,4-Difluoroaniline: This method involves the conversion of 2,4-difluoroaniline to the corresponding diazonium salt, followed by its reduction to yield 1,3-difluorobenzene. []
Q4: How does the reactivity of 1,3-difluorobenzene compare to benzene?
A4: The presence of two fluorine atoms in 1,3-difluorobenzene significantly impacts its reactivity compared to benzene:
- Electrophilic Aromatic Substitution: The electron-withdrawing nature of fluorine deactivates the aromatic ring, making electrophilic substitution reactions slower and requiring harsher conditions. []
- Nucleophilic Aromatic Substitution: The fluorine atoms, due to their electronegativity, activate the ring towards nucleophilic attack, particularly at the ortho and para positions. [, ]
- Metalation: The ortho-positions to the fluorine atoms are electronically activated for deprotonation by strong bases, enabling the formation of organometallic reagents for further functionalization. [, , ]
Q5: What are the key features and applications of C–H and C–F activation reactions involving 1,3-difluorobenzene?
A5: Both C–H and C–F activation reactions involving 1,3-difluorobenzene provide valuable synthetic tools:
- C–H Borylation: Iridium-catalyzed C–H borylation of 1,3-difluorobenzene allows for the introduction of a boronate ester group, a versatile intermediate for further functionalization, with high regioselectivity. [, ]
- C–F Activation: While less common, C–F activation can be achieved under specific conditions, often involving transition metal catalysts. This offers a pathway to replace fluorine with other functional groups. [, ]
Q6: Can you explain the regioselectivity observed in reactions of 1,3-difluorobenzene?
A6: The regioselectivity in reactions of 1,3-difluorobenzene arises from a combination of electronic and steric factors:
- Electronic Effects: The fluorine atoms direct electrophilic attack towards the ortho/para positions due to resonance effects. Conversely, they activate the ortho positions for deprotonation by strong bases. [, ]
- Steric Effects: The relatively small size of fluorine minimizes steric hindrance, allowing for reactions even at the ortho positions. []
Q7: How has computational chemistry been used to study 1,3-difluorobenzene?
A7: Computational methods have been instrumental in understanding various aspects of 1,3-difluorobenzene:
- Structural Characterization: Quantum chemical calculations have been employed to predict the equilibrium structure of 1,3-difluorobenzene, providing bond lengths and angles, and insights into ring deformation caused by fluorine substitution. [, ]
- Reaction Mechanisms: Computational studies can help elucidate reaction mechanisms, such as those involved in C–H and C–F activation, by providing information about transition states and intermediates. [, , ]
- Intermolecular Interactions: Calculations have been used to investigate the weak intermolecular forces present in 1,3-difluorobenzene, such as C–H⋯F hydrogen bonding and C–H⋯π interactions, which are important for crystal packing and complex formation with other molecules. [, ]
Q8: What are some notable applications of 1,3-difluorobenzene?
A8: 1,3-Difluorobenzene serves as a versatile building block in organic synthesis and finds applications in various fields:
- Pharmaceuticals: It acts as a key intermediate in the synthesis of several pharmaceuticals, including antifungal agents and antipsychotic drugs like Iloperidone and Risperidone. [, , ]
- Polymers and Materials Science: It can be incorporated into polymers to modify their properties, such as solubility, thermal stability, and optical characteristics. []
Q9: How does the presence of fluorine atoms influence the material properties of 1,3-difluorobenzene?
A9: The fluorine atoms in 1,3-difluorobenzene significantly affect its physical and chemical properties compared to benzene:
- Boiling Point: 1,3-Difluorobenzene has a lower boiling point (82-83 °C) than benzene (80 °C) due to weaker intermolecular forces. []
- Solubility: The presence of fluorine generally reduces the solubility of 1,3-difluorobenzene in polar solvents like water, while its solubility in non-polar solvents remains relatively high. []
- Dipole Moment: 1,3-Difluorobenzene possesses a dipole moment (μ = 1.48 D) due to the asymmetric arrangement of the fluorine atoms, making it more polar than benzene. []
Q10: Is there information available on the environmental impact and degradation of 1,3-difluorobenzene?
A10: While specific data on the environmental fate and ecotoxicological effects of 1,3-difluorobenzene might be limited, studies on the biodegradation of similar fluorinated aromatic compounds can offer some insights:
Haftungsausschluss und Informationen zu In-Vitro-Forschungsprodukten
Bitte beachten Sie, dass alle Artikel und Produktinformationen, die auf BenchChem präsentiert werden, ausschließlich zu Informationszwecken bestimmt sind. Die auf BenchChem zum Kauf angebotenen Produkte sind speziell für In-vitro-Studien konzipiert, die außerhalb lebender Organismen durchgeführt werden. In-vitro-Studien, abgeleitet von dem lateinischen Begriff "in Glas", beinhalten Experimente, die in kontrollierten Laborumgebungen unter Verwendung von Zellen oder Geweben durchgeführt werden. Es ist wichtig zu beachten, dass diese Produkte nicht als Arzneimittel oder Medikamente eingestuft sind und keine Zulassung der FDA für die Vorbeugung, Behandlung oder Heilung von medizinischen Zuständen, Beschwerden oder Krankheiten erhalten haben. Wir müssen betonen, dass jede Form der körperlichen Einführung dieser Produkte in Menschen oder Tiere gesetzlich strikt untersagt ist. Es ist unerlässlich, sich an diese Richtlinien zu halten, um die Einhaltung rechtlicher und ethischer Standards in Forschung und Experiment zu gewährleisten.