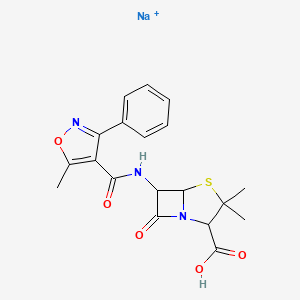
Oxacillin sodium
Übersicht
Beschreibung
Oxacillin sodium is a penicillin antibiotic used to treat a number of susceptible bacterial infections . It is a semisynthetic penicillin derived from the penicillin nucleus, 6-aminopenicillanic acid . It is resistant to inactivation by the enzyme penicillinase (beta-lactamase) and is available as the sodium salt in parenteral dosage form for intramuscular or intravenous use .
Synthesis Analysis
Oxacillin sodium is synthesized using a cell wall synthesis inhibitor, combined with Gram staining and machine vision (MV) analysis . A spectrophotometric method was developed for the determination of Oxacillin sodium based on charge transfer complexation reaction of the drug with iodine . A patent also describes the crystallization process of Oxacillin sodium .Molecular Structure Analysis
The molecular formula of Oxacillin sodium is C19H19N3O5S . The average molecular weight is 423.42 g/mol . The structure of Oxacillin sodium includes a 4-Thia-1-azabicyclo [3.2.0]heptane-2-carboxylic acid .Chemical Reactions Analysis
Oxacillin sodium is stable against hydrolysis by a variety of beta-lactamases, including penicillinases, and cephalosporinases and extended spectrum beta-lactamases .Physical And Chemical Properties Analysis
Oxacillin sodium is a fine, white, crystalline powder that is odorless or has a slight odor . It is freely soluble in water and has a pK of about 2.8 . The sodium content is 57.4 mg (2.5 mEq) per gram oxacillin .Wissenschaftliche Forschungsanwendungen
Analytical Chemistry Spectrophotometric Determination
A novel spectrophotometric method has been developed for the determination of Oxacillin sodium, which is based on charge transfer complexation reaction of the drug with iodine. This method is particularly useful in analyzing drug concentration in human urine samples .
Microbiology MRSA Identification
Oxacillin sodium salt is used in a simple and rapid method to discriminate between Methicillin-Resistant Staphylococcus aureus (MRSA) and Methicillin-Sensitive Staphylococcus aureus (MSSA). This involves treatment with Oxacillin sodium followed by Gram staining and microscopic examination .
Safety And Hazards
Oxacillin sodium may cause allergy or asthma symptoms or breathing difficulties if inhaled . It may cause an allergic skin reaction, eye irritation on direct contact, and ingestion may cause irritation and malaise . It may cause serious or fatal hypersensitivity (anaphylactic) reactions in some individuals .
Zukünftige Richtungen
Oxacillin sodium continues to be a subject of research, particularly in the context of enhancing its effectiveness against Methicillin-resistant Staphylococcus aureus (MRSA) and other resistant bacterial strains . The development of drug delivery systems that can enhance drug efficiency is one of the promising approaches being explored .
Eigenschaften
IUPAC Name |
sodium;(2S,5R,6R)-3,3-dimethyl-6-[(5-methyl-3-phenyl-1,2-oxazole-4-carbonyl)amino]-7-oxo-4-thia-1-azabicyclo[3.2.0]heptane-2-carboxylate | |
---|---|---|
Source | PubChem | |
URL | https://pubchem.ncbi.nlm.nih.gov | |
Description | Data deposited in or computed by PubChem | |
InChI |
InChI=1S/C19H19N3O5S.Na/c1-9-11(12(21-27-9)10-7-5-4-6-8-10)15(23)20-13-16(24)22-14(18(25)26)19(2,3)28-17(13)22;/h4-8,13-14,17H,1-3H3,(H,20,23)(H,25,26);/q;+1/p-1/t13-,14+,17-;/m1./s1 | |
Source | PubChem | |
URL | https://pubchem.ncbi.nlm.nih.gov | |
Description | Data deposited in or computed by PubChem | |
InChI Key |
VDUVBBMAXXHEQP-SLINCCQESA-M | |
Source | PubChem | |
URL | https://pubchem.ncbi.nlm.nih.gov | |
Description | Data deposited in or computed by PubChem | |
Canonical SMILES |
CC1=C(C(=NO1)C2=CC=CC=C2)C(=O)NC3C4N(C3=O)C(C(S4)(C)C)C(=O)[O-].[Na+] | |
Source | PubChem | |
URL | https://pubchem.ncbi.nlm.nih.gov | |
Description | Data deposited in or computed by PubChem | |
Isomeric SMILES |
CC1=C(C(=NO1)C2=CC=CC=C2)C(=O)N[C@H]3[C@@H]4N(C3=O)[C@H](C(S4)(C)C)C(=O)[O-].[Na+] | |
Source | PubChem | |
URL | https://pubchem.ncbi.nlm.nih.gov | |
Description | Data deposited in or computed by PubChem | |
Molecular Formula |
C19H18N3NaO5S | |
Source | PubChem | |
URL | https://pubchem.ncbi.nlm.nih.gov | |
Description | Data deposited in or computed by PubChem | |
Related CAS |
66-79-5 (Parent) | |
Record name | Oxacillin Sodium [USP] | |
Source | ChemIDplus | |
URL | https://pubchem.ncbi.nlm.nih.gov/substance/?source=chemidplus&sourceid=0001173882 | |
Description | ChemIDplus is a free, web search system that provides access to the structure and nomenclature authority files used for the identification of chemical substances cited in National Library of Medicine (NLM) databases, including the TOXNET system. | |
DSSTOX Substance ID |
DTXSID9048980 | |
Record name | Oxacillin sodium salt | |
Source | EPA DSSTox | |
URL | https://comptox.epa.gov/dashboard/DTXSID9048980 | |
Description | DSSTox provides a high quality public chemistry resource for supporting improved predictive toxicology. | |
Molecular Weight |
423.4 g/mol | |
Source | PubChem | |
URL | https://pubchem.ncbi.nlm.nih.gov | |
Description | Data deposited in or computed by PubChem | |
Product Name |
Oxacillin sodium | |
CAS RN |
1173-88-2, 7240-38-2 | |
Record name | Oxacillin Sodium [USP] | |
Source | ChemIDplus | |
URL | https://pubchem.ncbi.nlm.nih.gov/substance/?source=chemidplus&sourceid=0001173882 | |
Description | ChemIDplus is a free, web search system that provides access to the structure and nomenclature authority files used for the identification of chemical substances cited in National Library of Medicine (NLM) databases, including the TOXNET system. | |
Record name | Oxacillin sodium salt | |
Source | EPA DSSTox | |
URL | https://comptox.epa.gov/dashboard/DTXSID9048980 | |
Description | DSSTox provides a high quality public chemistry resource for supporting improved predictive toxicology. | |
Record name | Sodium [2S-(2α,5α,6β)]-3,3-dimethyl-6-[[(5-methyl-3-phenylisoxazol-4-yl)carbonyl]amino]-7-oxo-4-thia-1-azabicyclo[3.2.0]heptane-2-carboxylate | |
Source | European Chemicals Agency (ECHA) | |
URL | https://echa.europa.eu/substance-information/-/substanceinfo/100.013.306 | |
Description | The European Chemicals Agency (ECHA) is an agency of the European Union which is the driving force among regulatory authorities in implementing the EU's groundbreaking chemicals legislation for the benefit of human health and the environment as well as for innovation and competitiveness. | |
Explanation | Use of the information, documents and data from the ECHA website is subject to the terms and conditions of this Legal Notice, and subject to other binding limitations provided for under applicable law, the information, documents and data made available on the ECHA website may be reproduced, distributed and/or used, totally or in part, for non-commercial purposes provided that ECHA is acknowledged as the source: "Source: European Chemicals Agency, http://echa.europa.eu/". Such acknowledgement must be included in each copy of the material. ECHA permits and encourages organisations and individuals to create links to the ECHA website under the following cumulative conditions: Links can only be made to webpages that provide a link to the Legal Notice page. | |
Record name | Monosodium (2S,5R,6R)-3,3-dimethyl-6-(5-methyl-3-phenyl-4-isoxazolecarboxamido)-7-oxo-4-thia-1-azabicyclo [3.2.0] heptane-2-carboxylate monohydrate. | |
Source | European Chemicals Agency (ECHA) | |
URL | https://echa.europa.eu/information-on-chemicals | |
Description | The European Chemicals Agency (ECHA) is an agency of the European Union which is the driving force among regulatory authorities in implementing the EU's groundbreaking chemicals legislation for the benefit of human health and the environment as well as for innovation and competitiveness. | |
Explanation | Use of the information, documents and data from the ECHA website is subject to the terms and conditions of this Legal Notice, and subject to other binding limitations provided for under applicable law, the information, documents and data made available on the ECHA website may be reproduced, distributed and/or used, totally or in part, for non-commercial purposes provided that ECHA is acknowledged as the source: "Source: European Chemicals Agency, http://echa.europa.eu/". Such acknowledgement must be included in each copy of the material. ECHA permits and encourages organisations and individuals to create links to the ECHA website under the following cumulative conditions: Links can only be made to webpages that provide a link to the Legal Notice page. | |
Record name | OXACILLIN SODIUM ANHYDROUS | |
Source | FDA Global Substance Registration System (GSRS) | |
URL | https://gsrs.ncats.nih.gov/ginas/app/beta/substances/4TWD2995UP | |
Description | The FDA Global Substance Registration System (GSRS) enables the efficient and accurate exchange of information on what substances are in regulated products. Instead of relying on names, which vary across regulatory domains, countries, and regions, the GSRS knowledge base makes it possible for substances to be defined by standardized, scientific descriptions. | |
Explanation | Unless otherwise noted, the contents of the FDA website (www.fda.gov), both text and graphics, are not copyrighted. They are in the public domain and may be republished, reprinted and otherwise used freely by anyone without the need to obtain permission from FDA. Credit to the U.S. Food and Drug Administration as the source is appreciated but not required. | |
Retrosynthesis Analysis
AI-Powered Synthesis Planning: Our tool employs the Template_relevance Pistachio, Template_relevance Bkms_metabolic, Template_relevance Pistachio_ringbreaker, Template_relevance Reaxys, Template_relevance Reaxys_biocatalysis model, leveraging a vast database of chemical reactions to predict feasible synthetic routes.
One-Step Synthesis Focus: Specifically designed for one-step synthesis, it provides concise and direct routes for your target compounds, streamlining the synthesis process.
Accurate Predictions: Utilizing the extensive PISTACHIO, BKMS_METABOLIC, PISTACHIO_RINGBREAKER, REAXYS, REAXYS_BIOCATALYSIS database, our tool offers high-accuracy predictions, reflecting the latest in chemical research and data.
Strategy Settings
Precursor scoring | Relevance Heuristic |
---|---|
Min. plausibility | 0.01 |
Model | Template_relevance |
Template Set | Pistachio/Bkms_metabolic/Pistachio_ringbreaker/Reaxys/Reaxys_biocatalysis |
Top-N result to add to graph | 6 |
Feasible Synthetic Routes
Q & A
Q1: How does oxacillin sodium exert its antibacterial activity?
A: Oxacillin sodium is a β-lactam antibiotic that targets bacterial cell wall synthesis. It specifically inhibits the formation of peptidoglycans, essential components of the bacterial cell wall, by binding to penicillin-binding proteins (PBPs). This disruption of cell wall integrity leads to bacterial cell death. [, , , ]
Q2: What is the molecular formula and weight of oxacillin sodium?
A: The molecular formula of oxacillin sodium is C19H18N3NaO5S•H2O, and its molecular weight is 427.42 g/mol. [, ]
Q3: Are there any specific spectroscopic data available for oxacillin sodium?
A: Spectroscopic techniques like high-performance liquid chromatography-ultraviolet (HPLC-UV) are frequently employed to analyze oxacillin sodium. Studies have utilized a detection wavelength of 225 nm for HPLC analysis. [, ] Additionally, spectrophotometric methods based on charge-transfer reactions have been developed for oxacillin sodium determination. [, ]
Q4: How does storage temperature impact the stability of oxacillin sodium solutions?
A: Studies on intravenous oxacillin sodium solutions (5 mg/mL in 0.9% sodium chloride or 5% dextrose water) stored in Accufuser® devices reveal that storage at room temperature (25 ± 2°C) leads to significant decreases in oxacillin concentration and pH changes after two weeks. In contrast, solutions stored at controlled temperatures (4 ± 2°C) remained stable for up to six weeks. []
Q5: Can oxacillin sodium be frozen for storage?
A: Research indicates that carbenicillin disodium and oxacillin sodium, when frozen at -20°C in syringes (both glass and plastic) for three months, remain stable. This finding suggests the potential for freezing as a storage method, but further investigation is needed. []
Q6: Are there compatibility issues when administering oxacillin sodium with other drugs?
A: Yes, compatibility studies have shown that administering oxacillin sodium with certain drugs can lead to precipitation and drug degradation. For example, combining oxacillin sodium with verapamil hydrochloride results in significant loss of verapamil hydrochloride due to precipitation. [] Similarly, Y-site administration of vancomycin (10 mg/mL) and oxacillin (20 mg/mL) resulted in immediate precipitation, indicating incompatibility. [] Therefore, it is crucial to consult compatibility charts and guidelines before co-administering oxacillin sodium with other medications.
Q7: How does the structure of oxacillin sodium contribute to its penicillinase resistance?
A: Oxacillin sodium, a penicillinase-resistant penicillin, possesses a bulky side chain at position 6 of the penicillin nucleus. This steric hindrance prevents penicillinase, an enzyme produced by some bacteria to inactivate penicillins, from accessing and hydrolyzing the β-lactam ring, thus preserving its antibacterial activity. [, ]
Q8: Can the stability of oxacillin sodium in acidic environments be enhanced?
A: Research indicates that the stability of oxacillin sodium in acidic environments, like the stomach, can be improved by complexation with cyclodextrins (β-cyclodextrin and 2-HP-β-cyclodextrin). These cyclic oligosaccharides form inclusion complexes with oxacillin, shielding it from degradation and potentially enhancing its bioavailability. []
Q9: What analytical methods are commonly employed to assess the quality of oxacillin sodium?
A9: Various analytical methods are used to ensure the quality and purity of oxacillin sodium. These include:
- High-Performance Liquid Chromatography (HPLC): This technique is widely utilized to separate and quantify oxacillin sodium, as well as to identify and analyze related substances and impurities. [, , , , ]
- Spectrophotometry: This method, based on the absorption or transmission of light through a solution, is employed to determine the concentration of oxacillin sodium. [, ]
- Polarimetry: This technique measures the rotation of plane-polarized light by chiral molecules like oxacillin sodium and can be used for content determination. []
- Microbiological Assays: These assays assess the antibacterial activity of oxacillin sodium by measuring its ability to inhibit the growth of susceptible bacterial strains. []
Q10: What are the major mechanisms of resistance to oxacillin sodium in bacteria?
A: The primary mechanism of resistance to oxacillin sodium and other β-lactam antibiotics is the acquisition of the mecA gene. This gene encodes for penicillin-binding protein 2a (PBP2a), which has a low affinity for β-lactams, enabling resistant strains to continue cell wall synthesis even in the presence of these antibiotics. [, , ]
Q11: What are the potential adverse effects associated with oxacillin sodium use?
A: While generally safe, oxacillin sodium can cause adverse effects, including hypersensitivity reactions ranging from mild skin rashes to severe anaphylaxis. Other potential side effects include gastrointestinal disturbances, hematological abnormalities, and, rarely, hepatotoxicity. [, , , ]
Q12: Are there specific patient populations where oxacillin sodium use requires caution?
A: Oxacillin sodium use necessitates caution in patients with a history of penicillin allergy, as cross-reactivity can occur. Additionally, dosage adjustments may be required in patients with renal impairment to prevent drug accumulation and potential toxicity. [, , ]
Q13: Is there any information available on the environmental impact of oxacillin sodium?
A: Currently, the provided research papers do not delve into the environmental impact or degradation of oxacillin sodium. Further research is needed to assess its potential ecotoxicological effects and develop strategies for responsible disposal and mitigation of any negative environmental impacts. [, ]
Haftungsausschluss und Informationen zu In-Vitro-Forschungsprodukten
Bitte beachten Sie, dass alle Artikel und Produktinformationen, die auf BenchChem präsentiert werden, ausschließlich zu Informationszwecken bestimmt sind. Die auf BenchChem zum Kauf angebotenen Produkte sind speziell für In-vitro-Studien konzipiert, die außerhalb lebender Organismen durchgeführt werden. In-vitro-Studien, abgeleitet von dem lateinischen Begriff "in Glas", beinhalten Experimente, die in kontrollierten Laborumgebungen unter Verwendung von Zellen oder Geweben durchgeführt werden. Es ist wichtig zu beachten, dass diese Produkte nicht als Arzneimittel oder Medikamente eingestuft sind und keine Zulassung der FDA für die Vorbeugung, Behandlung oder Heilung von medizinischen Zuständen, Beschwerden oder Krankheiten erhalten haben. Wir müssen betonen, dass jede Form der körperlichen Einführung dieser Produkte in Menschen oder Tiere gesetzlich strikt untersagt ist. Es ist unerlässlich, sich an diese Richtlinien zu halten, um die Einhaltung rechtlicher und ethischer Standards in Forschung und Experiment zu gewährleisten.