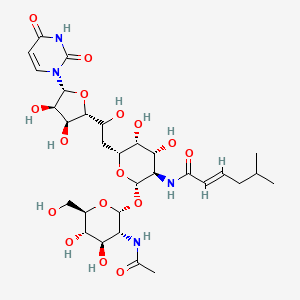
Tunicamycin
- Klicken Sie auf QUICK INQUIRY, um ein Angebot von unserem Expertenteam zu erhalten.
- Mit qualitativ hochwertigen Produkten zu einem WETTBEWERBSFÄHIGEN Preis können Sie sich mehr auf Ihre Forschung konzentrieren.
Übersicht
Beschreibung
Es ist ein Gemisch homologer Nukleosid-Antibiotika, das von verschiedenen Bakterien produziert wird, darunter Streptomyces clavuligerus und Streptomyces lysosuperficus . Tunicamycin blockiert die Bildung von N-glykosidischen Protein-Kohlenhydrat-Bindungen und ist daher ein wertvolles Werkzeug in der biologischen Forschung .
Wissenschaftliche Forschungsanwendungen
Tunicamycin hat eine breite Palette von Anwendungen in der wissenschaftlichen Forschung:
Wirkmechanismus
This compound hemmt die UDP-HexNAc: Polyprenol-P HexNAc-1-P-Familie von Enzymen, einschließlich GlcNAc-Phosphotransferase (GPT) . Dieses Enzym katalysiert den Transfer von N-Acetylglucosamin-1-Phosphat von UDP-N-Acetylglucosamin auf Dolicholphosphat, ein entscheidender Schritt in der Glykoproteinsynthese . Durch die Blockierung dieses Prozesses verhindert this compound die N-gebundene Glykosylierung, was zu einer Zellzyklusarretierung und anderen biologischen Wirkungen führt .
Wirkmechanismus
Target of Action
Tunicamycin primarily targets the UDP-HexNAc: polyprenol-P HexNAc-1-P family of enzymes . In eukaryotes, this includes the enzyme GlcNAc phosphotransferase (GPT), which catalyzes the transfer of N-acetylglucosamine-1-phosphate from UDP-N-acetylglucosamine to dolichol phosphate in the first step of glycoprotein synthesis .
Mode of Action
this compound inhibits the GPT enzyme, thereby blocking N-linked glycosylation (N-glycans) . This inhibition prevents the formation of dolichyl(pyro)-phosphate N-acetylglucosamine (dolichyl-PP-GlcNAc), a crucial intermediate in the glycosylation process .
Biochemical Pathways
By inhibiting N-linked glycosylation, this compound affects the protein glycosylation pathway . This disruption can lead to the accumulation of misfolded proteins in the endoplasmic reticulum, triggering the unfolded protein response .
Pharmacokinetics
It’s known that this compound can alter the disposition of oatp substrates, suggesting it may interact with drug transporters .
Result of Action
The inhibition of N-linked glycosylation by this compound can cause cell cycle arrest in the G1 phase in human cells . This can lead to cytotoxic effects, increasing the efficacy of certain anticancer drugs .
Action Environment
The action of this compound can be influenced by various environmental factors. For instance, the presence of other drugs or disease states can affect its efficacy and toxicity . .
Safety and Hazards
Zukünftige Richtungen
Tunicamycin is one of the potent nucleoside antibiotics and an inhibitor of aberrant glycosylation in various cancer cells, including breast cancer, gastric cancer, and pancreatic cancer, parallel with the inhibition of cancer cell growth and progression of tumors . Therefore, therapies targeting the activation of endoplasmic reticulum stress and its downstream signaling pathways are potentially helpful and novel tools to counteract and fight cancer .
Biochemische Analyse
Biochemical Properties
Tunicamycin inhibits the enzyme GlcNAc phosphotransferase (GPT), which catalyzes the transfer of N-acetylglucosamine-1-phosphate from UDP-N-acetylglucosamine to dolichol phosphate in the first step of glycoprotein synthesis . This inhibition blocks N-linked glycosylation (N-glycans), a major post-translational modification that plays a critical role in glycoprotein folding, stability, and subcellular localization .
Cellular Effects
This compound treatment causes cell cycle arrest in the G1 phase in human cells . It has been shown to significantly inhibit growth and arrest the cell cycle of breast cancer cells in a dose-dependent manner . Moreover, this compound treatment suppresses the migration and invasion of breast cancer cells . It also induces the unfolded protein response (UPR) by blocking aberrant glycosylation .
Molecular Mechanism
This compound’s mechanism of action is primarily through the inhibition of N-linked glycosylation . By blocking this process, this compound disrupts protein folding within the endoplasmic reticulum, leading to ER stress and the induction of the UPR . This can result in cell cycle arrest and apoptosis .
Temporal Effects in Laboratory Settings
This compound’s effects on cells can change over time. For example, it has been shown to cause marked morphological alterations and a significant inhibition of cell growth in transformed cells after 24 hours . Moreover, the cytotoxicity of this compound alters the morphology of their regular epithelioid shapes into long, pointed structures .
Dosage Effects in Animal Models
The effects of this compound can vary with different dosages in animal models. For instance, this compound has been shown to have potent antitumor activities in various cancers and may promote chemosensitivity . Its lack of cell-type-specific cytotoxicity impedes its anticancer efficacy .
Metabolic Pathways
This compound inhibits the first step in the lipid-linked saccharide pathway . This inhibition consequently disrupts the N-linked oligosaccharide formation of glycoproteins in the endoplasmic reticulum .
Transport and Distribution
The mechanism by which this compound enters cells has been a mystery. Recent research suggests that the human MFSD2A gene encodes a plasma membrane transporter that mediates the uptake of this compound into cells .
Subcellular Localization
This compound’s primary site of action is the endoplasmic reticulum, where it inhibits N-linked glycosylation, a critical process for protein folding that occurs within this organelle . This leads to the accumulation of misfolded proteins, causing ER stress and triggering the UPR .
Vorbereitungsmethoden
Tunicamycin wird von Bakterien wie Streptomyces chartreusis durch eine Reihe enzymatischer Reaktionen unter Beteiligung des Tun-Genclusters (TunA-N) synthetisiert . Die Biosynthese beginnt mit der Startereinheit Uridindiphosphat-N-Acetyl-Glucosamin (UDP-GlcNAc), die einer Dehydratation und anderen Modifikationen unterzogen wird, um das Endprodukt zu bilden . Industrielle Produktionsverfahren umfassen typischerweise die Kultivierung dieser Bakterien unter kontrollierten Bedingungen, um den Ertrag zu maximieren .
Analyse Chemischer Reaktionen
Tunicamycin unterliegt verschiedenen chemischen Reaktionen, darunter:
Oxidation: this compound kann unter bestimmten Bedingungen oxidiert werden, was zur Bildung verschiedener Oxidationsprodukte führt.
Reduktion: Reduktionsreaktionen können die funktionellen Gruppen in this compound modifizieren und so seine biologische Aktivität verändern.
Substitution: This compound kann Substitutionsreaktionen eingehen, bei denen bestimmte Atome oder Gruppen durch andere ersetzt werden.
Häufige Reagenzien, die in diesen Reaktionen verwendet werden, umfassen Oxidationsmittel wie Wasserstoffperoxid, Reduktionsmittel wie Natriumborhydrid und verschiedene Katalysatoren, um Substitutionsreaktionen zu erleichtern . Die wichtigsten gebildeten Produkte hängen von den spezifischen Reaktionsbedingungen und den verwendeten Reagenzien ab .
Vergleich Mit ähnlichen Verbindungen
Tunicamycin ist unter den Nukleosid-Antibiotika einzigartig aufgrund seiner spezifischen Hemmung der N-gebundenen Glykosylierung. Ähnliche Verbindungen umfassen:
Thapsigargin: Ein weiteres Nukleosid-Antibiotikum mit anderen biologischen Zielen und Wirkungen.
Rapamycin: Hemmt einen anderen Satz von Enzymen und hat unterschiedliche biologische Anwendungen.
Die Fähigkeit von this compound, die N-gebundene Glykosylierung zu blockieren, unterscheidet es von diesen Verbindungen und macht es zu einem wertvollen Werkzeug in bestimmten Forschungskontexten .
Eigenschaften
{ "Design of the Synthesis Pathway": "The synthesis pathway of Tunicamycin involves several steps that include the protection of functional groups, coupling reactions, and deprotection reactions. The overall strategy is to build up the molecule in a stepwise manner, starting from simpler building blocks and gradually introducing more complex functional groups.", "Starting Materials": [ "D-Glucosamine hydrochloride", "D-Mannose", "Acetic anhydride", "Pyridine", "Triethylamine", "Methanol", "Ethyl acetate", "Dimethylformamide", "Diisopropylcarbodiimide", "N,N-Dimethylaminopyridine", "Trifluoroacetic acid", "Toluene", "Hydrogen peroxide", "Sodium borohydride", "Sodium periodate", "Sodium chlorite", "Sodium hydroxide" ], "Reaction": [ "Protection of the hydroxyl groups of D-Mannose with acetic anhydride and pyridine to form the acetyl-protected intermediate", "Coupling of the acetyl-protected D-Mannose with D-Glucosamine hydrochloride using diisopropylcarbodiimide (DIC) and N,N-Dimethylaminopyridine (DMAP) as catalyst to form the disaccharide intermediate", "Deprotection of the acetyl groups using trifluoroacetic acid (TFA) to form the deacetylated intermediate", "Oxidation of the C4 hydroxyl group using sodium periodate to form the aldehyde intermediate", "Coupling of the aldehyde intermediate with the amino group of the deacetylated intermediate using sodium cyanoborohydride as reducing agent to form the imine intermediate", "Reduction of the imine intermediate using sodium borohydride to form the amine intermediate", "Protection of the amine group using tert-butyloxycarbonyl (Boc) to form the Boc-protected intermediate", "Oxidation of the C7 hydroxyl group using sodium chlorite to form the aldehyde intermediate", "Coupling of the aldehyde intermediate with the amino group of the Boc-protected intermediate using sodium cyanoborohydride as reducing agent to form the imine intermediate", "Reduction of the imine intermediate using sodium borohydride to form the amine intermediate", "Deprotection of the Boc group using trifluoroacetic acid (TFA) to form the final product, Tunicamycin" ] } | |
CAS-Nummer |
11089-65-9 |
Molekularformel |
C30H46N4O16 |
Molekulargewicht |
718.7 g/mol |
IUPAC-Name |
(E)-N-[(2S,3R,4R,5R,6R)-2-[(2R,3R,4R,5S,6R)-3-acetamido-4,5-dihydroxy-6-(hydroxymethyl)oxan-2-yl]oxy-6-[2-[(2R,3S,4R,5R)-5-(2,4-dioxopyrimidin-1-yl)-3,4-dihydroxyoxolan-2-yl]-2-hydroxyethyl]-4,5-dihydroxyoxan-3-yl]-5-methylhex-2-enamide |
InChI |
InChI=1S/C30H46N4O16/c1-11(2)5-4-6-16(38)32-19-23(43)20(40)14(47-29(19)50-28-18(31-12(3)36)22(42)21(41)15(10-35)48-28)9-13(37)26-24(44)25(45)27(49-26)34-8-7-17(39)33-30(34)46/h4,6-8,11,13-15,18-29,35,37,40-45H,5,9-10H2,1-3H3,(H,31,36)(H,32,38)(H,33,39,46)/b6-4+/t13?,14-,15-,18-,19-,20+,21-,22-,23-,24+,25-,26-,27-,28-,29+/m1/s1 |
InChI-Schlüssel |
ZHSGGJXRNHWHRS-VIDYELAYSA-N |
Isomerische SMILES |
CC(C)C/C=C/C(=O)N[C@@H]1[C@H]([C@H]([C@H](O[C@H]1O[C@@H]2[C@@H]([C@H]([C@@H]([C@H](O2)CO)O)O)NC(=O)C)CC([C@@H]3[C@H]([C@H]([C@@H](O3)N4C=CC(=O)NC4=O)O)O)O)O)O |
SMILES |
CC(C)CC=CC(=O)NC1C(C(C(OC1OC2C(C(C(C(O2)CO)O)O)NC(=O)C)CC(C3C(C(C(O3)N4C=CC(=O)NC4=O)O)O)O)O)O |
Kanonische SMILES |
CC(C)CC=CC(=O)NC1C(C(C(OC1OC2C(C(C(C(O2)CO)O)O)NC(=O)C)CC(C3C(C(C(O3)N4C=CC(=O)NC4=O)O)O)O)O)O |
Synonyme |
tunicamycin B2 |
Herkunft des Produkts |
United States |
Q1: What is the primary molecular target of tunicamycin?
A1: this compound inhibits N-linked glycosylation by blocking the enzyme UDP-N-acetylglucosamine: polyprenol phosphate translocase (GPT), specifically the bacterial enzyme MraY and its human homolog. [, , ] This enzyme catalyzes the first step in the biosynthesis of dolichol-linked oligosaccharides, essential for N-glycosylation. [, , ]
Q2: What are the downstream effects of this compound's inhibition of N-linked glycosylation?
A2: this compound treatment leads to various downstream effects, including:
- Endoplasmic Reticulum (ER) Stress: Accumulation of unfolded proteins in the ER, triggering the unfolded protein response (UPR). [, , , , , , , ]
- Autophagy: Activation of autophagy, a cellular degradation pathway, potentially as a response to ER stress. [, , ]
- Apoptosis: Induction of apoptosis, or programmed cell death, often as a consequence of prolonged ER stress. [, , , , , , , , ]
- Inhibition of Cell Proliferation and Growth: Disruption of cell cycle progression and inhibition of cell growth. [, , , , , ]
- Alterations in Cell Signaling: Modulation of various cell signaling pathways, including PI3K/Akt/mTOR. [, ]
- Changes in Cell Surface Properties: Altered adhesion, migration, and invasion of cells. [, , ]
Q3: How does this compound affect viral infection?
A3: this compound can inhibit viral replication by interfering with the glycosylation of viral proteins, crucial for their folding, assembly, and infectivity. [, ] For example, it prevents the formation of infectious virions and the expression of spike proteins in certain viruses. []
Q4: Does this compound affect insulin binding and proteoglycan synthesis?
A4: Research suggests that this compound can decrease insulin binding to chondrosarcoma chondrocytes, possibly due to the diminished synthesis of receptors for stimulatory hormones. [] It also suppresses proteoglycan synthesis without affecting the size or secretion of the molecule. []
Q5: How do structural modifications of this compound affect its activity?
A6: While the provided research doesn't delve deeply into specific structural modifications, it does highlight that the N-acetylglucosamine (GlcNAc) moiety is crucial for this compound's activity. Replacing it with a MurNAc amide can enhance the selectivity toward bacterial MraY over human GPT. []
Q6: Is there information on the stability of this compound under various conditions or formulation strategies to improve its properties?
A6: The provided research focuses primarily on this compound's biological activity and mechanisms. Detailed information on its stability, formulation strategies, or techniques to enhance its solubility or bioavailability is not included.
Q7: What analytical methods were used to study the effects of this compound?
A7: The research papers employed various methods, including:
- Western Blot Analysis: To assess protein expression levels, such as ER stress markers (GRP78, CHOP, PERK, eIF2α), apoptotic markers (PARP, caspase-9, caspase-3, Bax, Bcl-2), and signaling pathway proteins (PI3K/Akt/mTOR). [, , , , , , , , ]
- Reverse Transcription-Quantitative Polymerase Chain Reaction (RT-qPCR): To measure mRNA levels of specific genes, including ER stress markers and TRAIL receptors. [, , , ]
- Immunohistochemistry: To visualize the distribution and localization of proteins in tissues. [, ]
- Apoptosis Assays: To quantify apoptotic cells, such as flow cytometry using Annexin V/PI staining or analysis of sub-G1 cell cycle populations. [, , , , , , ]
- Immunofluorescence: To visualize cellular structures and protein localization. [, ]
- Cell Viability Assays: To assess cell viability and proliferation, such as MTT assays. [, , , , , ]
- Migration and Invasion Assays: To evaluate the effects on cell motility. [, , ]
- Electron Microscopy: To examine morphological changes in cells and organelles. []
Haftungsausschluss und Informationen zu In-Vitro-Forschungsprodukten
Bitte beachten Sie, dass alle Artikel und Produktinformationen, die auf BenchChem präsentiert werden, ausschließlich zu Informationszwecken bestimmt sind. Die auf BenchChem zum Kauf angebotenen Produkte sind speziell für In-vitro-Studien konzipiert, die außerhalb lebender Organismen durchgeführt werden. In-vitro-Studien, abgeleitet von dem lateinischen Begriff "in Glas", beinhalten Experimente, die in kontrollierten Laborumgebungen unter Verwendung von Zellen oder Geweben durchgeführt werden. Es ist wichtig zu beachten, dass diese Produkte nicht als Arzneimittel oder Medikamente eingestuft sind und keine Zulassung der FDA für die Vorbeugung, Behandlung oder Heilung von medizinischen Zuständen, Beschwerden oder Krankheiten erhalten haben. Wir müssen betonen, dass jede Form der körperlichen Einführung dieser Produkte in Menschen oder Tiere gesetzlich strikt untersagt ist. Es ist unerlässlich, sich an diese Richtlinien zu halten, um die Einhaltung rechtlicher und ethischer Standards in Forschung und Experiment zu gewährleisten.