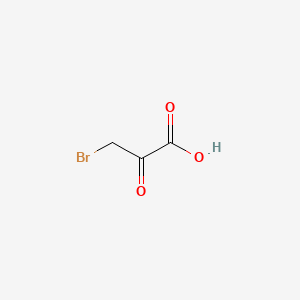
3-Bromopyruvat
- Klicken Sie auf QUICK INQUIRY, um ein Angebot von unserem Expertenteam zu erhalten.
- Mit qualitativ hochwertigen Produkten zu einem WETTBEWERBSFÄHIGEN Preis können Sie sich mehr auf Ihre Forschung konzentrieren.
Übersicht
Beschreibung
3-bromopyruvate is a 2-oxo monocarboxylic acid anion that is the conjugate base of 3-bromopyruvic acid, arising from deprotonation of the carboxy group. It is functionally related to a pyruvate. It is a conjugate base of a 3-bromopyruvic acid.
Wissenschaftliche Forschungsanwendungen
Krebshemmstoff
3-Bromopyruvat (3BP) gilt als potenter Krebshemmstoff und zeigt vielversprechende Eigenschaften als Therapeutikum gegen verschiedene Krebsarten. Es wirkt, indem es den Stoffwechsel von Krebszellen gezielt unterdrückt, der aufgrund von Genmutationen bevorzugt auf aerobe Glykolyse angewiesen ist .
Affinitätsmarkierung
Es dient als Affinitätsmarkierung für Cysteinreste in Proteinen. Diese Anwendung ist entscheidend für die Untersuchung von Proteinstrukturen und -funktionen, da sie die spezifische Markierung von Cysteinresten ermöglicht .
Synthese von heterozyklischen Verbindungen
This compound ist an der Synthese von Imidazo[1,2-a]pyridin-2-carbonsäuren und substituierten Pyranonen beteiligt, die in der medizinischen Chemie wertvoll sind, um verschiedene therapeutische Wirkstoffe zu erzeugen .
Vernetzer in der Biochemie
Es spielt eine wichtige Rolle als Vernetzer zwischen Nukleinsäuren und Proteinen, was für die Untersuchung der Interaktionen zwischen diesen Biomolekülen unerlässlich ist .
Hemmer der Glykolyse
Als Pyruvat-Mimetikum hemmt this compound (3-BP) die Glykolyse, was in Tierstudien eine Wirksamkeit bei der Verhinderung des Tumorwachstums und sogar bei der Ausrottung bestehender Tumoren gezeigt hat .
Stoffwechselforschung
Aufgrund seiner Wirkung auf die Glykolyse und den Energiestoffwechsel wird 3BP in der Stoffwechselforschung eingesetzt, um die Energiedynamik in Zellen zu verstehen .
Wirkmechanismus
Target of Action
3-Bromopyruvic acid (3-BP) is a potent anticancer compound that targets key enzymes involved in energy metabolism in cancer cells . The primary targets of 3-BP are glyceraldehyde-3-phosphate dehydrogenase (GAPDH) and hexokinase II (HK II) . These enzymes play crucial roles in glycolysis, a metabolic pathway that is often upregulated in cancer cells .
Mode of Action
3-BP acts as an alkylating agent and an antimetabolite of pyruvate . It inhibits its targets by alkylation, typically at cysteine side-chains, leading to the disruption of disulfide bridges . This inhibition disrupts the normal function of these enzymes, thereby suppressing glycolysis and mitochondrial metabolism .
Biochemical Pathways
The inhibition of GAPDH and HK II by 3-BP disrupts the glycolytic pathway, reducing the production of ATP, a key energy molecule . This disruption of energy production is particularly detrimental to cancer cells, which rely heavily on glycolysis for their energy needs . Additionally, 3-BP has been shown to harm mitochondrial NADH dehydrogenase activity .
Pharmacokinetics
It is known that 3-bp can be delivered into cells via the pyruvate transporter system . Once inside the cell, 3-BP exerts its effects on its target enzymes.
Result of Action
The action of 3-BP leads to a decrease in ATP production, causing energy depletion in cancer cells . This energy depletion can lead to cell death, thereby preventing tumor growth and even eradicating existing tumors . Furthermore, 3-BP has been shown to reduce the proliferation of certain types of cancer cells .
Action Environment
The efficacy of 3-BP can be influenced by environmental factors such as nutrient availability. For instance, starvation has been shown to sensitize certain cancer cells to 3-BP treatment . Additionally, the overexpression of certain proteins, such as proteasome activator (PA) 28, can lead to a partial resistance to the stress provoked by 3-BP .
Biochemische Analyse
Biochemical Properties
3-Bromopyruvic acid is known to interact with several enzymes, proteins, and other biomolecules. The primary intracellular target of 3-Bromopyruvic acid is glyceraldehyde-3-phosphate dehydrogenase, which is highly sensitive to inhibition by 3-Bromopyruvic acid . It also inhibits key glycolytic enzymes including hexokinase II , glyceraldehyde 3-phosphate dehydrogenase (GAPDH) , and lactate dehydrogenase (LDH) .
Cellular Effects
3-Bromopyruvic acid has significant effects on various types of cells and cellular processes. It suppresses cancer cell growth by targeting glycolytic and mitochondrial metabolism . It has been shown to decrease proliferation of hepatocellular carcinoma cells and reduce tumor growth in animal studies . It also influences cell function by disrupting glucose metabolism leading to cell death .
Molecular Mechanism
3-Bromopyruvic acid exerts its effects at the molecular level through several mechanisms. It is a strong alkylating agent and it irreversibly alkylates the glycolytic enzyme, glyceraldehyde-3-phosphate dehydrogenase (GAPDH), resulting in the disruption of glucose metabolism leading to cell death .
Dosage Effects in Animal Models
In animal models, the effects of 3-Bromopyruvic acid vary with different dosages. For instance, 3-Bromopyruvic acid treatment (50 mg/kg ip. daily, 6 days/week for three weeks) was effective in attenuating tumor growth and causing tumor necrosis in a hepatocellular carcinoma mouse xenograft model .
Metabolic Pathways
3-Bromopyruvic acid is involved in the glycolytic pathway, where it inhibits key enzymes and disrupts glucose metabolism . It also targets mitochondrial metabolism .
Transport and Distribution
3-Bromopyruvic acid is transported into cells via the pyruvate transporter system . Once intracellular, it interacts with various biomolecules to exert its effects
Subcellular Localization
Given its role as a glycolytic inhibitor and its effects on mitochondrial metabolism, it can be inferred that it likely localizes to the cytoplasm and mitochondria where these metabolic processes occur .
Eigenschaften
CAS-Nummer |
1113-59-3 |
---|---|
Molekularformel |
C3H2BrO3- |
Molekulargewicht |
165.95 g/mol |
IUPAC-Name |
3-bromo-2-oxopropanoate |
InChI |
InChI=1S/C3H3BrO3/c4-1-2(5)3(6)7/h1H2,(H,6,7)/p-1 |
InChI-Schlüssel |
PRRZDZJYSJLDBS-UHFFFAOYSA-M |
SMILES |
C(C(=O)C(=O)O)Br |
Kanonische SMILES |
C(C(=O)C(=O)[O-])Br |
Key on ui other cas no. |
1113-59-3 |
Piktogramme |
Corrosive |
Synonyme |
3-bromopyruvate 3-bromopyruvic acid bromopyruvate |
Herkunft des Produkts |
United States |
Retrosynthesis Analysis
AI-Powered Synthesis Planning: Our tool employs the Template_relevance Pistachio, Template_relevance Bkms_metabolic, Template_relevance Pistachio_ringbreaker, Template_relevance Reaxys, Template_relevance Reaxys_biocatalysis model, leveraging a vast database of chemical reactions to predict feasible synthetic routes.
One-Step Synthesis Focus: Specifically designed for one-step synthesis, it provides concise and direct routes for your target compounds, streamlining the synthesis process.
Accurate Predictions: Utilizing the extensive PISTACHIO, BKMS_METABOLIC, PISTACHIO_RINGBREAKER, REAXYS, REAXYS_BIOCATALYSIS database, our tool offers high-accuracy predictions, reflecting the latest in chemical research and data.
Strategy Settings
Precursor scoring | Relevance Heuristic |
---|---|
Min. plausibility | 0.01 |
Model | Template_relevance |
Template Set | Pistachio/Bkms_metabolic/Pistachio_ringbreaker/Reaxys/Reaxys_biocatalysis |
Top-N result to add to graph | 6 |
Feasible Synthetic Routes
Haftungsausschluss und Informationen zu In-Vitro-Forschungsprodukten
Bitte beachten Sie, dass alle Artikel und Produktinformationen, die auf BenchChem präsentiert werden, ausschließlich zu Informationszwecken bestimmt sind. Die auf BenchChem zum Kauf angebotenen Produkte sind speziell für In-vitro-Studien konzipiert, die außerhalb lebender Organismen durchgeführt werden. In-vitro-Studien, abgeleitet von dem lateinischen Begriff "in Glas", beinhalten Experimente, die in kontrollierten Laborumgebungen unter Verwendung von Zellen oder Geweben durchgeführt werden. Es ist wichtig zu beachten, dass diese Produkte nicht als Arzneimittel oder Medikamente eingestuft sind und keine Zulassung der FDA für die Vorbeugung, Behandlung oder Heilung von medizinischen Zuständen, Beschwerden oder Krankheiten erhalten haben. Wir müssen betonen, dass jede Form der körperlichen Einführung dieser Produkte in Menschen oder Tiere gesetzlich strikt untersagt ist. Es ist unerlässlich, sich an diese Richtlinien zu halten, um die Einhaltung rechtlicher und ethischer Standards in Forschung und Experiment zu gewährleisten.