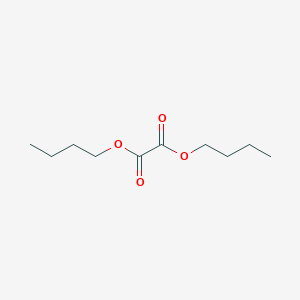
Dibutyl oxalate
Übersicht
Beschreibung
The rate of dibutyl oxalate degradation in ruminal contents from adapted and non-adapted animals was studied.
Oxalic acid dibutyl ester, also known as butyl oxalate or dibutyl ester OF oxalic acid, belongs to the class of organic compounds known as dicarboxylic acids and derivatives. These are organic compounds containing exactly two carboxylic acid groups. Oxalic acid dibutyl ester exists as a solid and is considered to be practically insoluble (in water) and relatively neutral. Within the cell, oxalic acid dibutyl ester is primarily located in the membrane (predicted from logP). Outside of the human body, oxalic acid dibutyl ester can be found in nuts and tea. This makes oxalic acid dibutyl ester a potential biomarker for the consumption of these food products.
Wissenschaftliche Forschungsanwendungen
Energy Storage Materials
Dibutyl oxalate, as part of transition metal oxalates, has been increasingly recognized for its application in energy storage materials. These materials have shown promise in various energy storage devices, including lithium-ion batteries, sodium-ion batteries, supercapacitors, and redox flow batteries. Transition metal oxalates are noted for their roles depending on the energy storage method, such as acting as a conversion-type negative electrode material in lithium-ion batteries or as a stabilizer additive in redox flow batteries. Their electrochemical performances are comparable to, or sometimes better than, their equivalent oxides and can be further improved through elemental substitution and blending with carbon-based materials. However, the details of the electrochemical reactions involved are still under investigation (Yeoh, Armer, & Lowe, 2018).
Urinary Calculi Treatment
In the realm of healthcare, especially concerning urinary calculi (kidney stones), this compound's role is indirectly noted. Calcium oxalate is the most common type of stone in urinary calculi, and while this compound itself is not directly used in treatment, the understanding of calcium oxalate's behavior and characteristics is crucial in managing and treating this condition. Tribulus terrestris, a medicinal plant, has shown efficacy in treating urinary calculi by interacting with the formation and behavior of calcium oxalate stones (Mahboubi, 2022).
Conservation of Cultural Heritage
In the field of cultural heritage conservation, the role of oxalates, including this compound, is being explored. Research into consolidants and protective treatments for porous calcareous materials such as limestone and lime plaster has covered treatments involving barium hydroxide and ammonium oxalate. These treatments aim to address issues related to application techniques, performance, compatibility, and retreatability in the conservation process (Hansen et al., 2003).
Oxalate-Degrading Bacteria in Probiotics
Oxalate-degrading bacteria, which can be found in the mammalian gut, play a significant role in degrading oxalate, a toxin detrimental to human health. The environmental factors influencing the efficacy of these probiotic bacteria in relation to oxalate metabolism have been studied. Understanding the mechanism of oxalate catabolism and its consumption by these bacteria provides insights into their potential therapeutic application in individuals with conditions like hyperoxaluria (Karamad et al., 2022).
Wirkmechanismus
Target of Action
Dibutyl oxalate is a type of organic compound that is primarily used as a solvent and in organic synthesis It is known that similar compounds, such as phthalates, can act as endocrine-disrupting chemicals, potentially affecting hormone balance and inducing neurological disorders .
Mode of Action
It is known that it can undergo monohydrolysis reactions, which involve the breaking of one ester bond in the this compound molecule . This process can lead to the formation of monoalkyl oxalates, which are important building blocks in organic synthesis .
Biochemical Pathways
DBP can be degraded by certain bacterial strains, leading to the formation of phthalic acid (PA) and other intermediates . These intermediates can then enter the tricarboxylic acid (TCA) cycle . It’s possible that this compound might have similar effects, but more research is needed to confirm this.
Pharmacokinetics
It is known that this compound can be synthesized in aqueous media, suggesting that it might be soluble in water to some extent .
Result of Action
Safety data sheets indicate that this compound can cause skin irritation, serious eye damage, and may cause respiratory irritation . These effects suggest that this compound can interact with cells and tissues, leading to these adverse reactions.
Action Environment
The action of this compound can be influenced by various environmental factors. For instance, the compound’s stability and efficacy can be affected by temperature, as its monohydrolysis reactions are carried out at around 0–5 °C . Moreover, safety data sheets recommend using this compound only outdoors or in a well-ventilated area, suggesting that air circulation can influence its action .
Safety and Hazards
Zukünftige Richtungen
Biochemische Analyse
Biochemical Properties
Dibutyl oxalate is involved in several biochemical reactions. It is used in the synthesis of monoalkyl oxalates, which are important building blocks for a variety of significant classes of compounds . The monohydrolysis of this compound is a key step in this process .
Cellular Effects
The cellular effects of this compound are not well-studied. Related compounds such as dibutyl phthalate have been shown to affect cellular processes. For example, exposure to dibutyl phthalate has been found to impair lipid metabolism and cause inflammation in cells .
Molecular Mechanism
It is known that it participates in the monohydrolysis reactions of symmetric diesters . This reaction is crucial in the synthesis of monoalkyl oxalates .
Temporal Effects in Laboratory Settings
Safety data sheets indicate that this compound poses no health hazard and would offer no hazard beyond that of ordinary combustible materials .
Dosage Effects in Animal Models
There is limited information available on the effects of this compound dosage in animal models. Studies on related compounds such as dibutyl phthalate have shown that exposure can lead to increased body weight and changes in hepatic triglyceride and total cholesterol levels .
Metabolic Pathways
Oxalate, a related compound, is known to be involved in several metabolic pathways in bacteria, including the glycolate pathway and the serine pathway .
Eigenschaften
IUPAC Name |
dibutyl oxalate | |
---|---|---|
Source | PubChem | |
URL | https://pubchem.ncbi.nlm.nih.gov | |
Description | Data deposited in or computed by PubChem | |
InChI |
InChI=1S/C10H18O4/c1-3-5-7-13-9(11)10(12)14-8-6-4-2/h3-8H2,1-2H3 | |
Source | PubChem | |
URL | https://pubchem.ncbi.nlm.nih.gov | |
Description | Data deposited in or computed by PubChem | |
InChI Key |
JKRZOJADNVOXPM-UHFFFAOYSA-N | |
Source | PubChem | |
URL | https://pubchem.ncbi.nlm.nih.gov | |
Description | Data deposited in or computed by PubChem | |
Canonical SMILES |
CCCCOC(=O)C(=O)OCCCC | |
Source | PubChem | |
URL | https://pubchem.ncbi.nlm.nih.gov | |
Description | Data deposited in or computed by PubChem | |
Molecular Formula |
C10H18O4 | |
Source | PubChem | |
URL | https://pubchem.ncbi.nlm.nih.gov | |
Description | Data deposited in or computed by PubChem | |
DSSTOX Substance ID |
DTXSID7041916 | |
Record name | Dibutyl ethanedioate | |
Source | EPA DSSTox | |
URL | https://comptox.epa.gov/dashboard/DTXSID7041916 | |
Description | DSSTox provides a high quality public chemistry resource for supporting improved predictive toxicology. | |
Molecular Weight |
202.25 g/mol | |
Source | PubChem | |
URL | https://pubchem.ncbi.nlm.nih.gov | |
Description | Data deposited in or computed by PubChem | |
Physical Description |
Clear colorless liquid with a butyl-like odor; [Acros Organics MSDS], Liquid | |
Record name | Dibutyl oxalate | |
Source | Haz-Map, Information on Hazardous Chemicals and Occupational Diseases | |
URL | https://haz-map.com/Agents/20381 | |
Description | Haz-Map® is an occupational health database designed for health and safety professionals and for consumers seeking information about the adverse effects of workplace exposures to chemical and biological agents. | |
Explanation | Copyright (c) 2022 Haz-Map(R). All rights reserved. Unless otherwise indicated, all materials from Haz-Map are copyrighted by Haz-Map(R). No part of these materials, either text or image may be used for any purpose other than for personal use. Therefore, reproduction, modification, storage in a retrieval system or retransmission, in any form or by any means, electronic, mechanical or otherwise, for reasons other than personal use, is strictly prohibited without prior written permission. | |
Record name | Oxalic acid dibutyl ester | |
Source | Human Metabolome Database (HMDB) | |
URL | http://www.hmdb.ca/metabolites/HMDB0040196 | |
Description | The Human Metabolome Database (HMDB) is a freely available electronic database containing detailed information about small molecule metabolites found in the human body. | |
Explanation | HMDB is offered to the public as a freely available resource. Use and re-distribution of the data, in whole or in part, for commercial purposes requires explicit permission of the authors and explicit acknowledgment of the source material (HMDB) and the original publication (see the HMDB citing page). We ask that users who download significant portions of the database cite the HMDB paper in any resulting publications. | |
Boiling Point |
241.00 °C. @ 760.00 mm Hg | |
Record name | Oxalic acid dibutyl ester | |
Source | Human Metabolome Database (HMDB) | |
URL | http://www.hmdb.ca/metabolites/HMDB0040196 | |
Description | The Human Metabolome Database (HMDB) is a freely available electronic database containing detailed information about small molecule metabolites found in the human body. | |
Explanation | HMDB is offered to the public as a freely available resource. Use and re-distribution of the data, in whole or in part, for commercial purposes requires explicit permission of the authors and explicit acknowledgment of the source material (HMDB) and the original publication (see the HMDB citing page). We ask that users who download significant portions of the database cite the HMDB paper in any resulting publications. | |
Vapor Pressure |
0.09 [mmHg] | |
Record name | Dibutyl oxalate | |
Source | Haz-Map, Information on Hazardous Chemicals and Occupational Diseases | |
URL | https://haz-map.com/Agents/20381 | |
Description | Haz-Map® is an occupational health database designed for health and safety professionals and for consumers seeking information about the adverse effects of workplace exposures to chemical and biological agents. | |
Explanation | Copyright (c) 2022 Haz-Map(R). All rights reserved. Unless otherwise indicated, all materials from Haz-Map are copyrighted by Haz-Map(R). No part of these materials, either text or image may be used for any purpose other than for personal use. Therefore, reproduction, modification, storage in a retrieval system or retransmission, in any form or by any means, electronic, mechanical or otherwise, for reasons other than personal use, is strictly prohibited without prior written permission. | |
CAS No. |
2050-60-4 | |
Record name | Dibutyl oxalate | |
Source | CAS Common Chemistry | |
URL | https://commonchemistry.cas.org/detail?cas_rn=2050-60-4 | |
Description | CAS Common Chemistry is an open community resource for accessing chemical information. Nearly 500,000 chemical substances from CAS REGISTRY cover areas of community interest, including common and frequently regulated chemicals, and those relevant to high school and undergraduate chemistry classes. This chemical information, curated by our expert scientists, is provided in alignment with our mission as a division of the American Chemical Society. | |
Explanation | The data from CAS Common Chemistry is provided under a CC-BY-NC 4.0 license, unless otherwise stated. | |
Record name | Dibutyl oxalate | |
Source | ChemIDplus | |
URL | https://pubchem.ncbi.nlm.nih.gov/substance/?source=chemidplus&sourceid=0002050604 | |
Description | ChemIDplus is a free, web search system that provides access to the structure and nomenclature authority files used for the identification of chemical substances cited in National Library of Medicine (NLM) databases, including the TOXNET system. | |
Record name | Dibutyl oxalate | |
Source | DTP/NCI | |
URL | https://dtp.cancer.gov/dtpstandard/servlet/dwindex?searchtype=NSC&outputformat=html&searchlist=8468 | |
Description | The NCI Development Therapeutics Program (DTP) provides services and resources to the academic and private-sector research communities worldwide to facilitate the discovery and development of new cancer therapeutic agents. | |
Explanation | Unless otherwise indicated, all text within NCI products is free of copyright and may be reused without our permission. Credit the National Cancer Institute as the source. | |
Record name | Ethanedioic acid, 1,2-dibutyl ester | |
Source | EPA Chemicals under the TSCA | |
URL | https://www.epa.gov/chemicals-under-tsca | |
Description | EPA Chemicals under the Toxic Substances Control Act (TSCA) collection contains information on chemicals and their regulations under TSCA, including non-confidential content from the TSCA Chemical Substance Inventory and Chemical Data Reporting. | |
Record name | Dibutyl ethanedioate | |
Source | EPA DSSTox | |
URL | https://comptox.epa.gov/dashboard/DTXSID7041916 | |
Description | DSSTox provides a high quality public chemistry resource for supporting improved predictive toxicology. | |
Record name | Dibutyl oxalate | |
Source | European Chemicals Agency (ECHA) | |
URL | https://echa.europa.eu/substance-information/-/substanceinfo/100.016.449 | |
Description | The European Chemicals Agency (ECHA) is an agency of the European Union which is the driving force among regulatory authorities in implementing the EU's groundbreaking chemicals legislation for the benefit of human health and the environment as well as for innovation and competitiveness. | |
Explanation | Use of the information, documents and data from the ECHA website is subject to the terms and conditions of this Legal Notice, and subject to other binding limitations provided for under applicable law, the information, documents and data made available on the ECHA website may be reproduced, distributed and/or used, totally or in part, for non-commercial purposes provided that ECHA is acknowledged as the source: "Source: European Chemicals Agency, http://echa.europa.eu/". Such acknowledgement must be included in each copy of the material. ECHA permits and encourages organisations and individuals to create links to the ECHA website under the following cumulative conditions: Links can only be made to webpages that provide a link to the Legal Notice page. | |
Record name | DIBUTYL OXALATE | |
Source | FDA Global Substance Registration System (GSRS) | |
URL | https://gsrs.ncats.nih.gov/ginas/app/beta/substances/S18C168OWC | |
Description | The FDA Global Substance Registration System (GSRS) enables the efficient and accurate exchange of information on what substances are in regulated products. Instead of relying on names, which vary across regulatory domains, countries, and regions, the GSRS knowledge base makes it possible for substances to be defined by standardized, scientific descriptions. | |
Explanation | Unless otherwise noted, the contents of the FDA website (www.fda.gov), both text and graphics, are not copyrighted. They are in the public domain and may be republished, reprinted and otherwise used freely by anyone without the need to obtain permission from FDA. Credit to the U.S. Food and Drug Administration as the source is appreciated but not required. | |
Record name | Oxalic acid dibutyl ester | |
Source | Human Metabolome Database (HMDB) | |
URL | http://www.hmdb.ca/metabolites/HMDB0040196 | |
Description | The Human Metabolome Database (HMDB) is a freely available electronic database containing detailed information about small molecule metabolites found in the human body. | |
Explanation | HMDB is offered to the public as a freely available resource. Use and re-distribution of the data, in whole or in part, for commercial purposes requires explicit permission of the authors and explicit acknowledgment of the source material (HMDB) and the original publication (see the HMDB citing page). We ask that users who download significant portions of the database cite the HMDB paper in any resulting publications. | |
Melting Point |
-29 °C | |
Record name | Oxalic acid dibutyl ester | |
Source | Human Metabolome Database (HMDB) | |
URL | http://www.hmdb.ca/metabolites/HMDB0040196 | |
Description | The Human Metabolome Database (HMDB) is a freely available electronic database containing detailed information about small molecule metabolites found in the human body. | |
Explanation | HMDB is offered to the public as a freely available resource. Use and re-distribution of the data, in whole or in part, for commercial purposes requires explicit permission of the authors and explicit acknowledgment of the source material (HMDB) and the original publication (see the HMDB citing page). We ask that users who download significant portions of the database cite the HMDB paper in any resulting publications. | |
Synthesis routes and methods I
Procedure details
Synthesis routes and methods II
Procedure details
Synthesis routes and methods III
Procedure details
Retrosynthesis Analysis
AI-Powered Synthesis Planning: Our tool employs the Template_relevance Pistachio, Template_relevance Bkms_metabolic, Template_relevance Pistachio_ringbreaker, Template_relevance Reaxys, Template_relevance Reaxys_biocatalysis model, leveraging a vast database of chemical reactions to predict feasible synthetic routes.
One-Step Synthesis Focus: Specifically designed for one-step synthesis, it provides concise and direct routes for your target compounds, streamlining the synthesis process.
Accurate Predictions: Utilizing the extensive PISTACHIO, BKMS_METABOLIC, PISTACHIO_RINGBREAKER, REAXYS, REAXYS_BIOCATALYSIS database, our tool offers high-accuracy predictions, reflecting the latest in chemical research and data.
Strategy Settings
Precursor scoring | Relevance Heuristic |
---|---|
Min. plausibility | 0.01 |
Model | Template_relevance |
Template Set | Pistachio/Bkms_metabolic/Pistachio_ringbreaker/Reaxys/Reaxys_biocatalysis |
Top-N result to add to graph | 6 |
Feasible Synthetic Routes
Q1: What is the molecular formula, weight, and structure of dibutyl oxalate?
A1: this compound has the molecular formula C10H18O4 and a molecular weight of 202.25 g/mol. Its structure consists of an oxalic acid backbone esterified with two n-butyl groups.
Q2: What spectroscopic data can be used to characterize this compound?
A2: this compound can be characterized using Fourier Transform Infrared Spectroscopy (FT-IR) and refractive index measurements. FT-IR analysis reveals characteristic peaks for dibutyl groups, confirming its identity [, ]. The refractive index aligns with literature values, further validating its purity [].
Q3: What are the common synthetic routes for this compound?
A3: this compound is primarily synthesized through the esterification of oxalic acid with n-butanol. This reaction is typically catalyzed by various agents, including:
- Strong acids: Sulfuric acid (H2SO4) [], p-toluene sulfonic acid []
- Solid superacids: SO42−/TiO2/Al2O3 []
- Metal salts: Ferric chloride (FeCl3) [], Potassium bisulfate (KHSO4) [], Zinc sulfate hydrate [], Stannic chloride (SnCl4) [, ], Nanometer Samarium Oxide (Sm2O3) []
- Supported catalysts: Activated carbon supported tungstosilicic acid [], stannic chloride pentahydrate/active carbon [], SO42−/Bentonite []
Q4: What factors influence the yield of this compound during synthesis?
A4: Several factors affect the yield of this compound synthesis, including:
- Catalyst type and concentration: Different catalysts exhibit varying activities. Optimal catalyst concentration is crucial to balance reaction rate and selectivity [, , , , , , , , , , , , ].
- Molar ratio of reactants: The ratio of n-butanol to oxalic acid significantly impacts the yield, with an excess of alcohol generally favoring esterification [, , , , , , , , , , , , ].
- Reaction temperature and time: Optimal temperature and time profiles are essential to maximize yield while minimizing side reactions [, , , , , , , , , , ].
- Water removal: Since esterification is an equilibrium reaction, removing water from the reaction mixture, often using a water-carrying agent like cyclohexane or toluene, drives the reaction towards product formation [, , , , , , , , , , , , ].
Q5: Can the catalysts used in this compound synthesis be reused?
A5: Yes, some catalysts used in this compound synthesis, such as potassium bisulfate [], strong acid ionic exchange resin [], activated carbon supported tungstosilicic acid [], and SO42−/Bentonite [], exhibit good reusability, maintaining their catalytic activity for multiple cycles.
Q6: What are the main applications of this compound?
A6: this compound has a variety of applications, including:
- Cetane number improver: this compound has been investigated as a potential cetane number improver for diesel fuel, particularly for diesel derived from direct coal liquefaction, which typically has a lower cetane number than conventional diesel [].
- Plasticizer: this compound can function as a plasticizer, improving the flexibility and workability of polymers [, ].
- Chemical intermediate: It serves as a valuable intermediate in synthesizing various organic compounds, including pharmaceuticals and polymers [, ].
Q7: How does the solubility of this compound vary?
A7: this compound exhibits varying solubility in different solvents. Studies have explored its solubility in binary solvent mixtures containing alkanes and other organic compounds like dimethyl adipate [].
Q8: Are there any studies on the environmental impact of this compound?
A8: While specific studies on the environmental impact of this compound are limited within the provided research, its use as a potential fuel additive suggests the importance of investigating its environmental fate and effects.
Q9: What are some areas for future research on this compound?
A9: Future research on this compound could focus on:
Haftungsausschluss und Informationen zu In-Vitro-Forschungsprodukten
Bitte beachten Sie, dass alle Artikel und Produktinformationen, die auf BenchChem präsentiert werden, ausschließlich zu Informationszwecken bestimmt sind. Die auf BenchChem zum Kauf angebotenen Produkte sind speziell für In-vitro-Studien konzipiert, die außerhalb lebender Organismen durchgeführt werden. In-vitro-Studien, abgeleitet von dem lateinischen Begriff "in Glas", beinhalten Experimente, die in kontrollierten Laborumgebungen unter Verwendung von Zellen oder Geweben durchgeführt werden. Es ist wichtig zu beachten, dass diese Produkte nicht als Arzneimittel oder Medikamente eingestuft sind und keine Zulassung der FDA für die Vorbeugung, Behandlung oder Heilung von medizinischen Zuständen, Beschwerden oder Krankheiten erhalten haben. Wir müssen betonen, dass jede Form der körperlichen Einführung dieser Produkte in Menschen oder Tiere gesetzlich strikt untersagt ist. Es ist unerlässlich, sich an diese Richtlinien zu halten, um die Einhaltung rechtlicher und ethischer Standards in Forschung und Experiment zu gewährleisten.