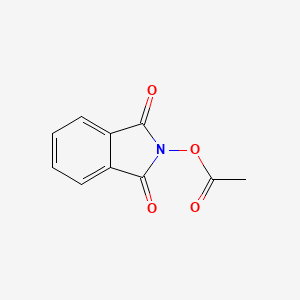
N-acetoxyphthalimide
Übersicht
Beschreibung
N-Acetoxyphthalimide (NAPI) is an acyloxyphthalimide derivative characterized by an acetoxy group (-OAc) attached to the nitrogen atom of the phthalimide ring (Fig. 1). Its molecular formula is C₁₀H₇NO₄, with a molecular weight of 205.16 g/mol . Synthesized via reaction of phthalimide with acetic acid derivatives, NAPI serves as a versatile reagent in organic synthesis, particularly in radical-mediated C–H functionalization, acetylation reactions, and polymer modification . Its mechanism often involves N–O bond cleavage under thermal or photochemical conditions, generating acetoxyl or methyl radicals for hydrogen atom transfer (HAT) or carbocation formation .
Vorbereitungsmethoden
Synthetic Routes and Reaction Conditions
N-acetoxyphthalimide can be synthesized through several methods. One common approach involves the reaction of phthalic anhydride with hydroxylamine to form N-hydroxyphthalimide, which is then acetylated using acetic anhydride to yield this compound . The reaction typically occurs under mild conditions and does not require a catalyst.
Industrial Production Methods
While specific industrial production methods for this compound are not extensively documented, the synthesis generally follows the laboratory procedures with scale-up modifications to accommodate larger quantities. The use of readily available starting materials and straightforward reaction conditions makes it feasible for industrial applications.
Analyse Chemischer Reaktionen
Photoredox Decarboxylative Coupling with Aryl Thiols
NAPI undergoes visible-light-driven decarboxylative coupling with aryl thiols without requiring an external photocatalyst. This reaction proceeds under mild conditions (room temperature, 10–20 hours) and tolerates functional groups such as halides, esters, and nitro groups.
Mechanism :
-
Visible light irradiation generates an electron-deficient intermediate via single-electron transfer (SET) from NAPI.
-
Decarboxylation releases CO, forming a carbon-centered radical.
-
Radical coupling with aryl thiols yields thioether products.
Key Data :
Substrate (Thiol) | Product Yield (%) | Conditions |
---|---|---|
4-Isopropylthiophenol | 93 | DMF, CsCO, 40 W CFL |
4-Trifluoromethylthiophenol | 85 | DMF, NaHCO, blue LED |
Methyl 4-mercaptobenzoate | 70 | DCE, KCO, 3 W LED |
Reductive N–O Bond Cleavage
Electrochemical studies reveal that NAPI undergoes reductive cleavage of the N–O bond in DMF, producing phthalimide radicals and acetate ions. This process is catalytic and self-sustaining due to electron transfer from the phthalimide radical anion back to NAPI.
Key Observations :
-
Reduction Potential : −1.2 V vs. SCE (irreversible wave).
-
Chain Propagation : Radical intermediates propagate decomposition, reducing the effective electron requirement to <1 e/molecule.
-
Byproducts : Carbonyl compounds (e.g., benzaldehyde) form via C–C bond cleavage.
Formation of N,O-Acetals via Carbonyl Insertion
NAPI reacts with aldehydes under LiBF/EtN catalysis to form N,O-acetals through insertion of the aldehyde carbonyl into the amide C–N bond. This method avoids toxic reagents like lead or mercury.
Example Reaction :
Scope :
-
Aldehydes : Isobutyraldehyde (79% yield), benzaldehyde (65%), formaldehyde (57%).
-
Limitations : Electron-deficient aldehydes (e.g., nitrobenzaldehyde) show reduced reactivity.
Radical-Mediated C–H Functionalization
NAPI serves as a nitrogen radical precursor in C–H amination reactions. Under visible light irradiation with Ir(ppy), it generates phthalimidyl radicals (PhthN- ), which add to arenes or heteroarenes.
Mechanistic Steps :
-
Photoexcitation : Ir(ppy) absorbs light, forming an excited state.
-
SET to NAPI : Generates PhthN- and regenerates Ir(ppy).
-
Radical Addition : PhthN- adds to aromatic substrates.
-
Deprotonation : Trifluoroacetate deprotonates the Wheland intermediate.
Applications :
-
Substrates : Toluene, xylene, pyridine derivatives.
-
Yields : 60–85% for heterocyclic amines.
Wissenschaftliche Forschungsanwendungen
Polymer Functionalization
1.1 Grafting onto Polyolefins
NAPI has been effectively utilized as a hydrogen-abstracting agent for the grafting of maleic anhydride (MA) onto polyethylene. The mechanism involves the thermal decomposition of NAPI at elevated temperatures (180°C to 250°C), which generates radicals that facilitate the grafting process. Studies have shown that using NAPI can achieve high grafting degrees, improving the material properties of polyethylene without significant crosslinking, which is often a drawback in similar processes using peroxides .
Table 1: Grafting Efficiency of NAPI on Polyethylene
Temperature (°C) | MA Grafting Degree (wt%) | Remarks |
---|---|---|
190 | 2.98 | Optimal conditions observed |
230 | Variable | Dependent on radical stability |
1.2 Modification of Poly(lactic acid) (PLA)
In another study, NAPI was used for the chemical modification of PLA through reactive extrusion. The thermal degradation of NAPI produced aminyl and nitroxide radicals, which were utilized to create macroradicals in PLA. This modification resulted in changes to the optical properties of PLA, with grafting rates reaching up to 0.24 mol% depending on the processing conditions .
Table 2: Grafting Rates in PLA Modification
NAPI Concentration (%) | Grafting Rate (mol%) | Temperature (°C) |
---|---|---|
0.5 | 0.12 | 180 |
1.0 | 0.24 | 200 |
Radical Initiation in Photoredox Reactions
NAPI has been identified as a pro-photosensitizer in visible-light photoredox reactions, specifically in decarboxylative arylthiation processes. The compound facilitates the generation of radicals under light irradiation, enabling efficient coupling reactions that are valuable in organic synthesis . This application showcases NAPI's potential beyond traditional thermal processes.
Thermal Decomposition Studies
Research has highlighted the decomposition behavior of NAPI under various conditions, revealing its utility as a radical source for polymer modification. The studies indicate complete decomposition within one hour at elevated temperatures, primarily through N-O bond dissociation mechanisms . This rapid decomposition is advantageous for industrial applications where time efficiency is crucial.
Case Study 1: Grafting Maleic Anhydride onto Polyethylene
A study conducted by Bélékian et al. demonstrated the use of NAPI for grafting MA onto low-density polyethylene (LDPE). The experiments were carried out using a mini-extruder at varying temperatures and initial MA concentrations, leading to optimized conditions that minimized unwanted crosslinking while maximizing grafting efficiency .
Case Study 2: Functionalization of Poly(lactic acid)
Rolere et al. investigated the modification of PLA using NAPI during melt processing. The study revealed that different processing parameters significantly affected the degree of functionalization and the resulting material properties, emphasizing the importance of controlling reaction conditions for desired outcomes .
Wirkmechanismus
The mechanism by which N-acetoxyphthalimide exerts its effects involves the formation of reactive intermediates. Upon activation, the compound can generate radicals or other reactive species that facilitate various chemical transformations. The molecular targets and pathways involved depend on the specific reaction context, but generally, the acetoxy group plays a crucial role in initiating these processes .
Vergleich Mit ähnlichen Verbindungen
N-Hydroxyphthalimide (NHPI) and Derivatives
NAPI is structurally related to NHPI (N-hydroxypthalimide) but differs in the substituent on the nitrogen (acetoxy vs. hydroxyl). This difference critically impacts their catalytic properties:
- Mechanistic Insight : Lower bond dissociation energy (BDE) of the N–O bond correlates with higher catalytic activity in oxidation reactions. In cumene oxidation, 4-tert-butyl-NHPI outperformed NAPI due to its lower BDE (32 vs. 50 kcal/mol), enabling faster hydroperoxide formation .
- Selectivity : In ethylbenzene aerobic oxidation, NAPI exhibited lower conversion efficiency compared to NHPI derivatives like NDHPI (N,N-dihydroxypyromellitimide) under identical conditions, likely due to steric and electronic effects of the acetoxy group .
Other N-Acyloxyphthalimide Derivatives
NAPI belongs to a broader class of N-acyloxyimides, including:
- N-Acetoxynaphthalimide (NANP) : Larger aromatic core enhances radical stability.
- N-Acetoxysuccinimide (NAS) : Smaller ring size increases reactivity but reduces thermal stability.
Property | NAPI | NANP | NAS |
---|---|---|---|
Decomposition Temp | 180°C | 200°C | 160°C |
Radical Stability | Moderate | High | Low |
Application | Polyethylene grafting | High-temperature polymer modifications | Low-temperature reactions |
- Thermal Stability : NAPI decomposes fully at 180°C via N–O cleavage, making it suitable for polymer functionalization in extruders (190–250°C). NANP’s higher stability allows prolonged radical generation in harsh conditions .
This compound vs. Alternative Acetylating Agents
In azide reduction/acetylation reactions, NAPI was compared with traditional reagents:
Reagent | Yield of 8a–c | Purity | Conditions |
---|---|---|---|
NAPI | Low (<50%) | Poor | H₂/Pd, DTT/DIPA |
Ac₂O (Acetic Anhydride) | High (>80%) | High | Standard acetylation |
- Limitation : NAPI’s poor performance in these reactions highlights its sensitivity to reaction conditions (e.g., moisture) and competing side reactions like Michael additions .
Photoredox Catalysis: Comparison with Peroxide-Based Systems
In photoredox C–H fluorination, NAPI’s role diverges from peroxide oxidants like TBPB (tert-butyl peroxybenzoate*):
- Advantage : NAPI enables broader nucleophile trapping (e.g., azides, thiols) via carbocation intermediates, whereas TBPB systems are less versatile .
Biologische Aktivität
N-acetoxyphthalimide (NAPI) is a compound that has garnered attention for its biological activity, particularly in the context of radical chemistry and potential applications in polymer functionalization. This article explores the biological properties, mechanisms of action, and applications of NAPI, supported by experimental data and case studies.
This compound is characterized by its ability to generate radicals upon thermal decomposition. At elevated temperatures, specifically around 180°C, NAPI decomposes primarily through N-O bond dissociation to produce acyloxyl and aminyl radicals. This radical generation is crucial for its application as an H-abstracting agent in various chemical reactions .
Decomposition Kinetics
The decomposition kinetics of NAPI have been studied extensively. For instance, complete decomposition occurs within one hour at 180°C, as confirmed by nuclear magnetic resonance (NMR) spectroscopy. The formation of acetic acid as a byproduct was also noted during this process . The radical species generated from NAPI are instrumental in facilitating reactions such as grafting maleic anhydride onto polyethylene .
Cytotoxicity and Mutagenicity
Research indicates that this compound exhibits significant cytotoxic and mutagenic properties. In genetic studies involving Drosophila, it was found that NAPI demonstrated higher mutagenicity compared to its parent amine compound. The mutagenicity was assessed through various genetic functions, revealing dose-dependent effects that were consistent across different concentrations .
Table 1: Summary of Biological Activity Studies on NAPI
Applications in Polymer Chemistry
This compound's radical-generating ability has led to its application in polymer chemistry, particularly in the functionalization of polyolefins and polylactides. Studies have shown that using NAPI in reactive extrusion processes enhances the degree of grafting with maleic anhydride significantly .
Case Study: Grafting Maleic Anhydride
In a practical application, the use of NAPI as a radical initiator resulted in a grafting degree of up to 2.98 wt% when applied to polyethylene. This demonstrates not only the efficiency of NAPI as a radical source but also its potential utility in modifying polymer properties for various industrial applications .
Q & A
Basic Research Questions
Q. What are the primary synthetic routes for preparing N-acetoxyphthalimide, and how do reaction conditions influence yield and purity?
this compound is commonly synthesized via nucleophilic substitution or cross-dehydrogenative coupling (CDC) reactions. For example, phthalimide derivatives react with acetylating agents under acidic or basic conditions. Optimization requires controlling stoichiometry, temperature (e.g., 25–80°C), and solvent polarity. Impurities such as unreacted phthalimide or over-acetylated byproducts can be minimized using column chromatography or recrystallization .
Q. How does this compound function as a catalyst in oxidation reactions, and what experimental parameters enhance its activity?
this compound acts as a redox-active catalyst in aerobic oxidations (e.g., cumene to hydroperoxide). Its activity correlates inversely with the bond dissociation energy (BDE) of the N–O bond; lower BDE values favor radical initiation. Key parameters include substrate-to-catalyst ratios (typically 1–5 mol%), solvent choice (polar aprotic solvents like acetonitrile), and oxygen pressure (1–5 atm). Yields up to 50% are achievable under moderate conditions .
Q. What safety and handling protocols are critical when working with isotopically labeled this compound (e.g., [¹⁴C] or [³H] variants)?
Radiolabeled this compound (e.g., [acetoxy-¹⁴C], 1.85–2.22 GBq/mmol) requires shielded storage in ethyl acetate at –20°C. Handling mandates glove boxes, dose-rate monitors, and waste neutralization protocols. Purification via HPLC with radiometric detection ensures isotopic integrity. Specific activity must be verified before use to avoid experimental artifacts .
Advanced Research Questions
Q. How can computational methods predict the catalytic efficiency of this compound derivatives in novel reaction systems?
Density functional theory (DFT) calculations can model N–O bond cleavage energetics and radical stabilization. Parameters like reduction potential (Ered ≈ –1.1 V) and frontier molecular orbitals (HOMO/LUMO gaps) predict reactivity in decarboxylation or C–H activation pathways. Comparative studies with TEMPO or NHPI analogs reveal substituent effects on catalytic cycles .
Q. What analytical techniques resolve contradictions in reported catalytic performance across different studies?
Discrepancies in turnover numbers (TON) or selectivity may arise from trace metal impurities or solvent effects. Use inductively coupled plasma mass spectrometry (ICP-MS) to detect metals, and electron paramagnetic resonance (EPR) to confirm radical intermediates. Kinetic studies (e.g., Arrhenius plots) under standardized conditions (pH, temperature) isolate variables .
Q. How do kinetic isotope effects (KIE) elucidate the mechanism of this compound-mediated oxidations?
KIE experiments using deuterated substrates (e.g., cumene-d12) compare rate constants (kH/kD) to distinguish hydrogen abstraction steps. A KIE > 2 indicates rate-limiting H-atom transfer, while KIE ≈ 1 suggests electron transfer dominates. Isotopic labeling of the catalyst’s acetoxy group ([³H]) tracks its participation in radical chains .
Q. What strategies improve the stability of this compound in long-term catalytic applications?
Encapsulation in mesoporous silica or covalent grafting onto polymeric supports reduces decomposition. Stabilizing additives (e.g., hydroquinone) suppress premature radical termination. Real-time monitoring via in situ IR or UV-vis spectroscopy identifies degradation pathways (e.g., hydrolysis or photolysis) .
Q. Methodological Considerations
Table 1: Key Physicochemical Properties of this compound
Property | Value/Method | Reference |
---|---|---|
Reduction Potential (Ered) | –1.1 V vs. SCE (measured by cyclic voltammetry) | |
BDE (N–O) | ~45 kcal/mol (calculated via DFT) | |
Solubility | >50 mg/mL in ethyl acetate, DMSO | |
Radiolabeled Specific Activity | 1.85–2.22 GBq/mmol (¹⁴C), 30–60 Ci/mmol (³H) |
Table 2: Comparative Catalytic Performance in Cumene Oxidation
Catalyst | Yield (%) | Conditions | Reference |
---|---|---|---|
This compound | 50 | 25°C, O2 balloon | |
4-tert-Butyl-NHPI | 65 | 25°C, O2 balloon | |
TEMPO | 75 | 60°C, 5 atm O2 |
Q. Critical Analysis and Future Directions
- Gaps in Literature: Limited data on enantioselective applications or scalability in flow reactors.
- Recommendations: Explore co-catalytic systems with transition metals (e.g., Fe, Cu) to enhance TON. Integrate operando spectroscopy for mechanistic clarity.
Eigenschaften
IUPAC Name |
(1,3-dioxoisoindol-2-yl) acetate | |
---|---|---|
Source | PubChem | |
URL | https://pubchem.ncbi.nlm.nih.gov | |
Description | Data deposited in or computed by PubChem | |
InChI |
InChI=1S/C10H7NO4/c1-6(12)15-11-9(13)7-4-2-3-5-8(7)10(11)14/h2-5H,1H3 | |
Source | PubChem | |
URL | https://pubchem.ncbi.nlm.nih.gov | |
Description | Data deposited in or computed by PubChem | |
InChI Key |
IJRNYIWWGDQEIY-UHFFFAOYSA-N | |
Source | PubChem | |
URL | https://pubchem.ncbi.nlm.nih.gov | |
Description | Data deposited in or computed by PubChem | |
Canonical SMILES |
CC(=O)ON1C(=O)C2=CC=CC=C2C1=O | |
Source | PubChem | |
URL | https://pubchem.ncbi.nlm.nih.gov | |
Description | Data deposited in or computed by PubChem | |
Molecular Formula |
C10H7NO4 | |
Source | PubChem | |
URL | https://pubchem.ncbi.nlm.nih.gov | |
Description | Data deposited in or computed by PubChem | |
DSSTOX Substance ID |
DTXSID50295404 | |
Record name | N-acetoxyphthalimide | |
Source | EPA DSSTox | |
URL | https://comptox.epa.gov/dashboard/DTXSID50295404 | |
Description | DSSTox provides a high quality public chemistry resource for supporting improved predictive toxicology. | |
Molecular Weight |
205.17 g/mol | |
Source | PubChem | |
URL | https://pubchem.ncbi.nlm.nih.gov | |
Description | Data deposited in or computed by PubChem | |
CAS No. |
17720-64-8 | |
Record name | 2-(Acetyloxy)-1H-isoindole-1,3(2H)-dione | |
Source | CAS Common Chemistry | |
URL | https://commonchemistry.cas.org/detail?cas_rn=17720-64-8 | |
Description | CAS Common Chemistry is an open community resource for accessing chemical information. Nearly 500,000 chemical substances from CAS REGISTRY cover areas of community interest, including common and frequently regulated chemicals, and those relevant to high school and undergraduate chemistry classes. This chemical information, curated by our expert scientists, is provided in alignment with our mission as a division of the American Chemical Society. | |
Explanation | The data from CAS Common Chemistry is provided under a CC-BY-NC 4.0 license, unless otherwise stated. | |
Record name | NSC 101749 | |
Source | ChemIDplus | |
URL | https://pubchem.ncbi.nlm.nih.gov/substance/?source=chemidplus&sourceid=0017720648 | |
Description | ChemIDplus is a free, web search system that provides access to the structure and nomenclature authority files used for the identification of chemical substances cited in National Library of Medicine (NLM) databases, including the TOXNET system. | |
Record name | NSC101749 | |
Source | DTP/NCI | |
URL | https://dtp.cancer.gov/dtpstandard/servlet/dwindex?searchtype=NSC&outputformat=html&searchlist=101749 | |
Description | The NCI Development Therapeutics Program (DTP) provides services and resources to the academic and private-sector research communities worldwide to facilitate the discovery and development of new cancer therapeutic agents. | |
Explanation | Unless otherwise indicated, all text within NCI products is free of copyright and may be reused without our permission. Credit the National Cancer Institute as the source. | |
Record name | N-acetoxyphthalimide | |
Source | EPA DSSTox | |
URL | https://comptox.epa.gov/dashboard/DTXSID50295404 | |
Description | DSSTox provides a high quality public chemistry resource for supporting improved predictive toxicology. | |
Record name | 1,3-dioxo-2,3-dihydro-1H-isoindol-2-yl acetate | |
Source | European Chemicals Agency (ECHA) | |
URL | https://echa.europa.eu/information-on-chemicals | |
Description | The European Chemicals Agency (ECHA) is an agency of the European Union which is the driving force among regulatory authorities in implementing the EU's groundbreaking chemicals legislation for the benefit of human health and the environment as well as for innovation and competitiveness. | |
Explanation | Use of the information, documents and data from the ECHA website is subject to the terms and conditions of this Legal Notice, and subject to other binding limitations provided for under applicable law, the information, documents and data made available on the ECHA website may be reproduced, distributed and/or used, totally or in part, for non-commercial purposes provided that ECHA is acknowledged as the source: "Source: European Chemicals Agency, http://echa.europa.eu/". Such acknowledgement must be included in each copy of the material. ECHA permits and encourages organisations and individuals to create links to the ECHA website under the following cumulative conditions: Links can only be made to webpages that provide a link to the Legal Notice page. | |
Retrosynthesis Analysis
AI-Powered Synthesis Planning: Our tool employs the Template_relevance Pistachio, Template_relevance Bkms_metabolic, Template_relevance Pistachio_ringbreaker, Template_relevance Reaxys, Template_relevance Reaxys_biocatalysis model, leveraging a vast database of chemical reactions to predict feasible synthetic routes.
One-Step Synthesis Focus: Specifically designed for one-step synthesis, it provides concise and direct routes for your target compounds, streamlining the synthesis process.
Accurate Predictions: Utilizing the extensive PISTACHIO, BKMS_METABOLIC, PISTACHIO_RINGBREAKER, REAXYS, REAXYS_BIOCATALYSIS database, our tool offers high-accuracy predictions, reflecting the latest in chemical research and data.
Strategy Settings
Precursor scoring | Relevance Heuristic |
---|---|
Min. plausibility | 0.01 |
Model | Template_relevance |
Template Set | Pistachio/Bkms_metabolic/Pistachio_ringbreaker/Reaxys/Reaxys_biocatalysis |
Top-N result to add to graph | 6 |
Feasible Synthetic Routes
Haftungsausschluss und Informationen zu In-Vitro-Forschungsprodukten
Bitte beachten Sie, dass alle Artikel und Produktinformationen, die auf BenchChem präsentiert werden, ausschließlich zu Informationszwecken bestimmt sind. Die auf BenchChem zum Kauf angebotenen Produkte sind speziell für In-vitro-Studien konzipiert, die außerhalb lebender Organismen durchgeführt werden. In-vitro-Studien, abgeleitet von dem lateinischen Begriff "in Glas", beinhalten Experimente, die in kontrollierten Laborumgebungen unter Verwendung von Zellen oder Geweben durchgeführt werden. Es ist wichtig zu beachten, dass diese Produkte nicht als Arzneimittel oder Medikamente eingestuft sind und keine Zulassung der FDA für die Vorbeugung, Behandlung oder Heilung von medizinischen Zuständen, Beschwerden oder Krankheiten erhalten haben. Wir müssen betonen, dass jede Form der körperlichen Einführung dieser Produkte in Menschen oder Tiere gesetzlich strikt untersagt ist. Es ist unerlässlich, sich an diese Richtlinien zu halten, um die Einhaltung rechtlicher und ethischer Standards in Forschung und Experiment zu gewährleisten.