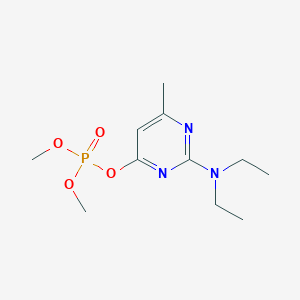
pirimiphos-methyl-oxon
Übersicht
Beschreibung
pirimiphos-methyl-oxon is an organophosphate compound widely used as an insecticide. It is a derivative of pirimiphos-methyl, which is known for its effectiveness in controlling a variety of insect pests in stored products and agricultural settings . The compound is characterized by its ability to inhibit acetylcholinesterase, an enzyme essential for the proper functioning of the nervous system in insects .
Vorbereitungsmethoden
Synthetic Routes and Reaction Conditions
pirimiphos-methyl-oxon is synthesized through a multi-step process. The initial step involves the reaction of N,N-diethylguanidine with ethyl acetoacetate to form a pyrimidine ring. This intermediate is then reacted with dimethyl chlorothiophosphate to produce pirimiphos-methyl . The oxon derivative is obtained by further oxidation of pirimiphos-methyl using an oxidizing agent such as hydrogen peroxide or m-chloroperbenzoic acid under controlled conditions .
Industrial Production Methods
Industrial production of pirimiphos methyl oxon follows similar synthetic routes but on a larger scale. The process involves stringent control of reaction conditions to ensure high yield and purity of the final product. The use of continuous flow reactors and automated systems helps in maintaining consistent quality and efficiency in production .
Analyse Chemischer Reaktionen
Metabolic Activation of Pirimiphos-Methyl to Pirimiphos-Methyl-Oxon
PMO is generated via cytochrome P450-mediated oxidative desulfuration of pirimiphos-methyl (PM), converting the parent compound’s thiophosphate (P=S) group to an oxon (P=O) group . This activation enhances its reactivity toward biological targets:
Reaction:
Kinetics : In a human overdose case, PMO reached peak plasma concentration 18 hours post-ingestion and exhibited a prolonged half-life (~80 hours) .
-
Toxicological Significance : The oxon form is 100–1,000× more potent than PM in inhibiting AChE due to its electrophilic phosphorus center .
AChE Inhibition and Phosphorylation Mechanism
PMO exerts toxicity by covalently phosphorylating serine residues in AChE’s catalytic triad, irreversibly blocking acetylcholine hydrolysis:
Reaction:
Aging : Over time, the phosphorylated enzyme undergoes dealkylation (“aging”), rendering it resistant to reactivation .
-
Reactivation Potential : Oximes (e.g., obidoxime) can regenerate active AChE by cleaving the phosphoryl-PMO adduct if administered before aging .
Table 1 : Key parameters of PMO-mediated AChE inhibition
Parameter | Value |
---|---|
Peak plasma PMO concentration | 18 hours post-exposure |
Time to 50% AChE activity | ~90 hours |
Reactivation window | <24–48 hours (pre-aging) |
Environmental Degradation Pathways
PMO undergoes hydrolysis and enzymatic degradation in environmental matrices, though persistence is notable:
Primary Reactions :
-
Hydrolysis :
-
Microbial Metabolism :
-
Soil ba
-
Wissenschaftliche Forschungsanwendungen
Agricultural Applications
Pirimiphos-methyl is widely employed as an insecticide in agriculture, particularly for the protection of stored grains and food crops. Its oxidative metabolite, PMO, plays a crucial role in studying pesticide residues and their impact on food safety.
Insect Control
- Effectiveness : PMO is effective against a variety of pests, including Coleoptera (e.g., maize weevil) and Diptera species. Studies indicate that it can significantly reduce pest populations when applied to stored products .
- Resistance Studies : Research has identified resistance to PMO among Anopheles mosquitoes in West Africa, highlighting the need for continuous monitoring and adaptation of pest control strategies .
Clinical Applications
Pirimiphos-methyl and its oxon derivative are also pertinent in clinical toxicology due to their potential for human exposure and poisoning.
Toxicological Studies
- Case Reports : A notable case involved severe intoxication from PMO, leading to cholinergic crisis symptoms. Treatment involved the use of atropine and obidoxime to reactivate acetylcholinesterase (AChE), demonstrating the clinical relevance of PMO in poisoning cases .
- Biochemical Effects : Research indicates that exposure to sub-lethal concentrations of pirimiphos-methyl can lead to significant changes in liver and kidney weights in animal models, suggesting potential health risks associated with environmental exposure .
Environmental Monitoring
PMO is utilized as a standard for contaminant analysis in environmental studies. Its detection is crucial for assessing pesticide residues in food products and ecological systems.
Residue Analysis
- Detection Methods : Analytical techniques such as gas chromatography have been developed to quantify PMO residues in agricultural products. These methods help ensure compliance with safety standards and regulations .
- Environmental Impact Assessments : Studies have shown that PMO can persist in the environment, necessitating regular monitoring to mitigate its ecological impact .
Data Tables
The following tables summarize key findings related to the applications of pirimiphos-methyl-oxon:
Wirkmechanismus
pirimiphos-methyl-oxon exerts its effects by inhibiting acetylcholinesterase, an enzyme responsible for breaking down acetylcholine in the nervous system. The inhibition leads to an accumulation of acetylcholine, causing continuous stimulation of nerve cells, which eventually results in paralysis and death of the insect . The molecular targets include the active site of acetylcholinesterase, where the compound binds and prevents the enzyme from functioning properly .
Vergleich Mit ähnlichen Verbindungen
Similar Compounds
Pirimiphos-methyl: The parent compound, which is less potent than its oxon derivative.
Pyrimiphos-ethyl: A related insecticide with ethoxy groups instead of methoxy groups.
Malathion: Another organophosphate insecticide with a similar mechanism of action.
Uniqueness
pirimiphos-methyl-oxon is unique due to its high potency and effectiveness in inhibiting acetylcholinesterase compared to its parent compound and other similar insecticides . Its ability to act at lower concentrations makes it a valuable tool in pest control and scientific research .
Biologische Aktivität
Pirimiphos-methyl-oxon (PMO) is an organophosphorus compound that serves as a metabolite of the insecticide pirimiphos-methyl. This compound is significant due to its biological activity, particularly its effects on acetylcholinesterase (AChE) inhibition, which leads to cholinergic toxicity. This article delves into the biological activity of PMO, supported by case studies, research findings, and data tables.
Pirimiphos-methyl (PM) is a phosphorothioate used primarily as an insecticide. Upon metabolism, it converts to PMO, which is more toxic and acts as a potent inhibitor of AChE. The mechanism involves the phosphorylation of serine residues in the active site of AChE, leading to the accumulation of acetylcholine in synaptic clefts. This results in overstimulation of both muscarinic and nicotinic receptors, causing symptoms characteristic of cholinergic crisis .
Biological Activity and Toxicity
The biological activity of PMO can be assessed through its effects on various biological systems. Notably:
- Acute Toxicity : PMO exhibits high acute toxicity in mammals and birds, with significant interspecies variability in hydrolytic activity. This variability suggests that certain species may be more susceptible to PMO than others due to differences in metabolic pathways .
- Cholinergic Crisis : In cases of severe poisoning, such as documented in a clinical report, patients exhibited symptoms consistent with cholinergic toxicity, including respiratory distress and neurological impairment. The treatment involved the administration of atropine and oxime reactivators like obidoxime .
Case Study 1: Severe Intoxication
A 63-year-old male ingested ethanol and PM with suicidal intent. He displayed significant cholinergic symptoms upon admission, with AChE activity markedly inhibited. Treatment included continuous infusion of atropine and obidoxime. Notably, despite treatment, the patient experienced complications such as pneumonia and sepsis, highlighting the severe impact of PMO on health .
Case Study 2: Avian Species Response
Research involving various avian species revealed low hydrolytic activity against PMO. This finding indicates that birds have a higher susceptibility to PMO toxicity compared to mammals. The study compared PTE activities across species, showing that lower PTE levels correlate with increased vulnerability to organophosphate oxons like PMO .
Research Findings
Recent studies have quantified the persistence and biological activity of pirimiphos-methyl under varying environmental conditions:
- Persistence : The biological activity of PM was assessed at different grain temperatures during spraying. Results indicated that higher temperatures could affect the degradation rate and effectiveness of PM as an insecticide .
- Metabolism Studies : Investigations into the metabolic pathways revealed that cytochrome P450 enzymes play a crucial role in converting PM to its more toxic form, PMO. This metabolic activation underscores the importance of understanding species-specific responses to PMO exposure .
Data Tables
Eigenschaften
IUPAC Name |
[2-(diethylamino)-6-methylpyrimidin-4-yl] dimethyl phosphate | |
---|---|---|
Source | PubChem | |
URL | https://pubchem.ncbi.nlm.nih.gov | |
Description | Data deposited in or computed by PubChem | |
InChI |
InChI=1S/C11H20N3O4P/c1-6-14(7-2)11-12-9(3)8-10(13-11)18-19(15,16-4)17-5/h8H,6-7H2,1-5H3 | |
Source | PubChem | |
URL | https://pubchem.ncbi.nlm.nih.gov | |
Description | Data deposited in or computed by PubChem | |
InChI Key |
QPBHUVHLWZEFRS-UHFFFAOYSA-N | |
Source | PubChem | |
URL | https://pubchem.ncbi.nlm.nih.gov | |
Description | Data deposited in or computed by PubChem | |
Canonical SMILES |
CCN(CC)C1=NC(=CC(=N1)OP(=O)(OC)OC)C | |
Source | PubChem | |
URL | https://pubchem.ncbi.nlm.nih.gov | |
Description | Data deposited in or computed by PubChem | |
Molecular Formula |
C11H20N3O4P | |
Source | PubChem | |
URL | https://pubchem.ncbi.nlm.nih.gov | |
Description | Data deposited in or computed by PubChem | |
Molecular Weight |
289.27 g/mol | |
Source | PubChem | |
URL | https://pubchem.ncbi.nlm.nih.gov | |
Description | Data deposited in or computed by PubChem | |
Retrosynthesis Analysis
AI-Powered Synthesis Planning: Our tool employs the Template_relevance Pistachio, Template_relevance Bkms_metabolic, Template_relevance Pistachio_ringbreaker, Template_relevance Reaxys, Template_relevance Reaxys_biocatalysis model, leveraging a vast database of chemical reactions to predict feasible synthetic routes.
One-Step Synthesis Focus: Specifically designed for one-step synthesis, it provides concise and direct routes for your target compounds, streamlining the synthesis process.
Accurate Predictions: Utilizing the extensive PISTACHIO, BKMS_METABOLIC, PISTACHIO_RINGBREAKER, REAXYS, REAXYS_BIOCATALYSIS database, our tool offers high-accuracy predictions, reflecting the latest in chemical research and data.
Strategy Settings
Precursor scoring | Relevance Heuristic |
---|---|
Min. plausibility | 0.01 |
Model | Template_relevance |
Template Set | Pistachio/Bkms_metabolic/Pistachio_ringbreaker/Reaxys/Reaxys_biocatalysis |
Top-N result to add to graph | 6 |
Feasible Synthetic Routes
Haftungsausschluss und Informationen zu In-Vitro-Forschungsprodukten
Bitte beachten Sie, dass alle Artikel und Produktinformationen, die auf BenchChem präsentiert werden, ausschließlich zu Informationszwecken bestimmt sind. Die auf BenchChem zum Kauf angebotenen Produkte sind speziell für In-vitro-Studien konzipiert, die außerhalb lebender Organismen durchgeführt werden. In-vitro-Studien, abgeleitet von dem lateinischen Begriff "in Glas", beinhalten Experimente, die in kontrollierten Laborumgebungen unter Verwendung von Zellen oder Geweben durchgeführt werden. Es ist wichtig zu beachten, dass diese Produkte nicht als Arzneimittel oder Medikamente eingestuft sind und keine Zulassung der FDA für die Vorbeugung, Behandlung oder Heilung von medizinischen Zuständen, Beschwerden oder Krankheiten erhalten haben. Wir müssen betonen, dass jede Form der körperlichen Einführung dieser Produkte in Menschen oder Tiere gesetzlich strikt untersagt ist. Es ist unerlässlich, sich an diese Richtlinien zu halten, um die Einhaltung rechtlicher und ethischer Standards in Forschung und Experiment zu gewährleisten.