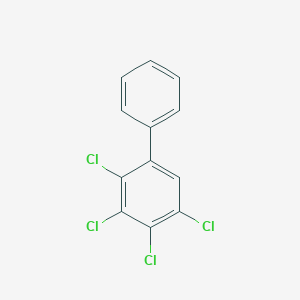
2,3,4,5-Tetrachlorobiphenyl
Übersicht
Beschreibung
2,3,4,5-Tetrachlorobiphenyl (CAS: 33284-53-6; IUPAC name: 1,1′-biphenyl-2,3,4,5-tetrachloride), also referred to as PCB 61, is a tetra-chlorinated biphenyl with chlorine atoms substituted at the 2, 3, 4, and 5 positions on one benzene ring. Its molecular formula is C₁₂H₆Cl₄, with a molecular weight of 291.99 g/mol . PCB 61 is a persistent organic pollutant (POP) commonly studied for its environmental behavior, microbial dechlorination kinetics, and interactions in biological systems .
Vorbereitungsmethoden
Direct Chlorination of Biphenyl
The foundational method for synthesizing 2,3,4,5-tetrachlorobiphenyl involves the direct chlorination of biphenyl under carefully regulated conditions. This process employs chlorine gas (Cl₂) in the presence of catalysts such as ferric chloride (FeCl₃) or aluminum chloride (AlCl₃) to achieve regioselective chlorination .
Reaction Mechanism and Conditions
The reaction proceeds via electrophilic aromatic substitution, where chlorine atoms replace hydrogen atoms on the biphenyl ring. The positioning of chlorine atoms at the 2, 3, 4, and 5 positions is controlled by adjusting:
-
Temperature : Optimal chlorination occurs between 40–60°C. Higher temperatures favor ortho/para substitution, while lower temperatures reduce side reactions .
-
Catalyst Loading : FeCl₃ at 5–10 wt% relative to biphenyl ensures efficient chlorination without over-substitution .
-
Chlorine Flow Rate : A gradual introduction of Cl₂ (0.5–1.0 L/min) prevents exothermic runaway reactions .
Industrial-Scale Production
Historically, batch reactors with reflux condensers were used to synthesize PCBs at scale. Post-chlorination, fractional distillation or column chromatography isolates this compound from other congeners . Industrial yields for tetra-ortho-substituted PCBs typically range from 60–75%, though specific data for this congener remain proprietary .
Multi-Step Synthesis via Ullmann Coupling
An alternative route involves the Ullmann coupling of pre-chlorinated benzene derivatives. This method offers greater control over substitution patterns but requires complex intermediates.
Synthetic Pathway
-
Preparation of 2,3,4-Trichlorobromobenzene :
Bromination of 1,2,3-trichlorobenzene using Br₂ in the presence of FeBr₃ yields 2,3,4-trichlorobromobenzene . -
Coupling with Chlorinated Benzene :
A copper-catalyzed Ullmann reaction between 2,3,4-trichlorobromobenzene and 1,2-dichlorobenzene forms the biphenyl backbone . -
Final Chlorination :
Residual unsubstituted positions are chlorinated using Cl₂/FeCl₃ to achieve the target compound .
Table 1: Key Reaction Parameters for Ullmann Coupling
Step | Reagents/Conditions | Yield (%) | Purity (%) |
---|---|---|---|
Bromination | Br₂, FeBr₃, 110°C, 6 h | 82 | 95 |
Ullmann Coupling | Cu powder, DMF, 150°C, 24 h | 68 | 89 |
Final Chlorination | Cl₂, FeCl₃, 50°C, 8 h | 74 | 97 |
Catalytic Dechlorination of Higher Chlorinated Congeners
Recent advances leverage reductive dechlorination to synthesize this compound from pentachlorobiphenyls. This method aligns with green chemistry principles by reducing waste.
Microbial Dechlorination
Dehalobium chlorocoercia DF-1, a dehalorespiring bacterium, selectively removes chlorine atoms from pentachlorobiphenyls under anaerobic conditions . Key parameters include:
Chemical Reductive Methods
Palladium-catalyzed hydrodechlorination using H₂ gas achieves similar results in hours rather than months:
Purity and Isomer Control
Achieving >99% purity requires advanced separation techniques:
Chromatographic Methods
-
HPLC : Reverse-phase C18 columns with acetonitrile/water mobile phases resolve PCB congeners .
-
GC-MS : Quantifies trace impurities (<0.1%) using DB-5MS columns .
Crystallization
Recrystallization from ligroine (petroleum ether) enhances purity to 99.5%, with a documented melting point of 104°C .
Analyse Chemischer Reaktionen
Types of Reactions
2,3,4,5-Tetrachlorobiphenyl undergoes several types of chemical reactions, including:
Reductive Dechlorination: This reaction involves the removal of chlorine atoms from the biphenyl ring.
Oxidation: The compound can undergo oxidative degradation, especially in the presence of strong oxidizing agents.
Substitution: Chlorine atoms on the biphenyl ring can be substituted with other functional groups under appropriate reaction conditions.
Common Reagents and Conditions
Reductive Dechlorination: Common reagents include electron donors such as hydrogen or organic acids.
Oxidation: Strong oxidizing agents such as potassium permanganate or ozone can be used to oxidize this compound.
Substitution: Various nucleophiles can be used to substitute chlorine atoms on the biphenyl ring.
Major Products Formed
Reductive Dechlorination: The major products include lower chlorinated biphenyls such as 2,3,5-Trichlorobiphenyl.
Oxidation: The major products include various oxidized derivatives of the biphenyl ring.
Substitution: The major products depend on the nature of the substituent introduced into the biphenyl ring.
Wissenschaftliche Forschungsanwendungen
2,3,4,5-Tetrachlorobiphenyl has been extensively studied for its environmental impact and potential health hazards. Some of its scientific research applications include:
Environmental Studies: Research on the persistence, bioaccumulation, and degradation of polychlorinated biphenyls in various environmental matrices.
Toxicology: Studies on the toxic effects of polychlorinated biphenyls on human health and wildlife.
Bioremediation: Research on the use of microbial communities to degrade polychlorinated biphenyls in contaminated environments.
Analytical Chemistry: Development of analytical methods for the detection and quantification of polychlorinated biphenyls in environmental samples.
Wirkmechanismus
The mechanism of action of 2,3,4,5-Tetrachlorobiphenyl involves its interaction with various molecular targets and pathways. It is known to:
Disrupt Endocrine Function: Polychlorinated biphenyls can interfere with the normal functioning of the endocrine system by mimicking or blocking hormones.
Induce Oxidative Stress: The compound can generate reactive oxygen species, leading to oxidative damage to cells and tissues.
Affect Gene Expression: Polychlorinated biphenyls can alter the expression of genes involved in various biological processes.
Vergleich Mit ähnlichen Verbindungen
Physicochemical Properties
PCB 61’s chlorine substitution pattern significantly influences its polarity and hydrophobicity compared to other tetra- and pentachlorobiphenyls:
Compound | Chlorine Positions | Polarity (σ-profile) | ΔGhydr (kcal/mol) | Log Kow (Estimated) |
---|---|---|---|---|
PCB 61 | 2,3,4,5 | Less polar | -1.2* | ~6.0–6.5† |
2,2',6,6'-Tetrachlorobiphenyl | 2,2',6,6' | More polar | -2.4* | ~6.8–7.2† |
3,3',4,4'-Tetrachlorobiphenyl (PCB 77) | 3,3',4,4' | Coplanar, highly polar | N/A | ~6.5–7.0‡ |
2,3,3',4'-Tetrachlorobiphenyl (PCB 66) | 2,3,3',4' | Moderately polar | N/A | ~6.2–6.7† |
Notes:
- *PCB 61 exhibits 1.2 kcal/mol lower free energy of hydration than 2,2',6,6'-tetrachlorobiphenyl, indicating reduced hydrophobicity .
- †Estimated Log Kow (octanol-water partition coefficient) based on structural analogs .
- ‡PCB 77 (coplanar) has higher Log Kow due to planar structure favoring lipid solubility .
Environmental Persistence and Degradation
Dechlorination Pathways and Rates:
- PCB 61 : Undergoes reductive dechlorination primarily via Dehalococcoides -like bacteria in anaerobic sediments. Key pathways include meta - and para -chlorine removal, with degradation rates influenced by aqueous-phase concentration thresholds (e.g., 3.4 × 10⁻³ nM) and sediment amendments (e.g., iron oxides enhance rates by 30–50%) .
- PCB 77: Degradation is slower due to coplanar structure, requiring specialized microbial consortia. Limited data on threshold levels .
- 2,2',6,6'-Tetrachlorobiphenyl : Higher persistence in aerobic environments due to steric hindrance from ortho-chlorines .
Key Factors Affecting Degradation:
Compound | Degradation Rate | Microorganisms | Enhancing Factors |
---|---|---|---|
PCB 61 | Moderate | Dehalococcoides spp. | Iron oxides, surfactants |
PCB 77 | Slow | Uncharacterized consortia | Bioelectrochemical systems |
PCB 66 | Moderate | Mixed sediment communities | Organic carbon amendments |
Bioaccumulation and Toxicity
Bioaccumulation Potential:
- PCB 61: Limited experimental data, but structural analogs (e.g., 3,3’,4,5-tetrachlorobiphenyl) show Log BMFL (biomagnification factor in fish) values (~-2.52) lower than other tetra-PCBs (range: 0.22–0.80) . This suggests reduced biomagnification due to lower hydrophobicity.
- PCB 77 : Higher bioaccumulation (Log BMFL ~1.5–2.0) due to coplanarity and affinity for lipid tissues .
Toxicity Profiles:
- PCB 61: Induces oxidative stress in Oryza sativa (rice), activating CYP450s, GSTs, and phenylpropanoid biosynthesis pathways within 0.5–12 hours of exposure .
- PCB 77 : Causes developmental toxicity in mice (e.g., reduced striatal dopamine) and dioxin-like effects via aryl hydrocarbon receptor activation .
- 2,2',6,6'-Tetrachlorobiphenyl : Lower acute toxicity but chronic effects due to environmental persistence .
Microbial Interactions
- PCB 61: Supported by phylogenetically similar Dehalococcoides populations across diverse sediments (estuarine, marine, riverine), with dechlorination activity sustained at nanomolar concentrations .
- PCB 81 (3,4,4',5-tetrachlorobiphenyl) : Requires distinct dechlorination pathways, often involving Chloroflexi species .
Biologische Aktivität
2,3,4,5-Tetrachlorobiphenyl (PCB 61) is one of the polychlorinated biphenyl (PCB) congeners that has garnered significant attention due to its environmental persistence and potential biological impacts. This article provides a comprehensive overview of its biological activity, focusing on its toxicity, degradation mechanisms, and the microbial communities involved in its bioremediation.
This compound is characterized by four chlorine atoms attached to a biphenyl structure. This configuration contributes to its stability and resistance to degradation in the environment. PCBs are widely recognized as environmental pollutants due to their lipophilicity and ability to bioaccumulate in the food chain.
Toxicological Effects
Research indicates that this compound exhibits various toxicological effects on biological systems:
Microbial Degradation Mechanisms
Microbial communities play a crucial role in the bioremediation of PCB-contaminated environments. The following mechanisms have been identified for the degradation of this compound:
- Reductive Dechlorination : Anaerobic bacteria such as Dehalococcoides have been shown to effectively dechlorinate PCB 61. In laboratory settings, cultures enriched from various sediments demonstrated the ability to remove chlorines from PCB 61 through reductive dechlorination processes .
- Bioelectrochemical Systems : Recent studies have explored the use of bioelectrochemical reactors (BERs) enhanced with iron oxides to improve the dechlorination rates of PCB 61. The presence of dissimilatory iron-reducing bacteria (DIRB) such as Geobacter and Desulfovibrio significantly increased PCB transformation rates .
Case Studies
Several case studies illustrate the biological activity of PCB 61:
- Sediment Microcosm Experiments : In a controlled study using sediment microcosms from Baltimore Harbor, researchers observed that adding PCB 61 resulted in significant changes in microbial community composition. The presence of fatty acids or elemental iron as electron donors facilitated the growth of PCB-degrading bacteria .
- Field Studies in Contaminated Sites : Field investigations have shown that sediments contaminated with PCBs harbor diverse microbial populations capable of degrading these compounds. For example, studies indicated that Dehalococcoides-like populations were statistically correlated with the removal of doubly flanked chlorines from PCB 61 across different sediment types .
Data Tables
The following table summarizes key findings related to the biological activity and degradation pathways of this compound:
Q & A
Basic Research Questions
Q. How can 2,3,4,5-tetrachlorobiphenyl be reliably identified and quantified in environmental samples?
- Methodological Answer : Use gas chromatography-mass spectrometry (GC-MS) with electron capture detection (ECD) for high sensitivity. Calibrate with certified reference standards (e.g., 100 µg/mL in isooctane) to ensure accuracy. Confirm retention times using isomer-specific standards, as co-elution with structurally similar tetrachlorobiphenyls (e.g., 2,3,4,6- or 2,3',4,5-tetrachlorobiphenyl) is common . For sediment or biota samples, employ Soxhlet extraction followed by silica gel cleanup to remove interfering lipids .
Q. What are the key physicochemical properties of this compound relevant to environmental persistence?
- Methodological Answer : Determine its octanol-water partition coefficient (log Kow ≈ 6.4) to predict bioaccumulation potential . Measure vapor pressure (e.g., 1.2 × 10<sup>-4</sup> Pa at 25°C) and aqueous solubility (<0.1 mg/L) to model atmospheric transport and leaching behavior. Use quantitative structure-activity relationship (QSAR) tools to estimate degradation half-lives in soil (>1 year) .
Q. How does the chlorination pattern of this compound influence its analytical and toxicological profile?
- Methodological Answer : Compare its reactivity with other tetrachlorobiphenyl isomers (e.g., 2,3',4,5- or 3,3',4,4'-tetrachlorobiphenyl) using molecular docking studies or in vitro assays. The ortho-chlorine absence in this compound reduces steric hindrance, enhancing binding affinity to aryl hydrocarbon receptors (AhR) compared to non-planar congeners .
Advanced Research Questions
Q. What microbial consortia are effective in degrading this compound under anaerobic conditions?
- Methodological Answer : Enrich sediment microcosms with Dehalococcoides-like bacteria, which mediate reductive dechlorination. Monitor chlorine removal patterns via GC-ECD: this compound typically undergoes meta-dechlorination to trichlorobiphenyls (e.g., 2,3,5- or 2,4,5-trichlorobiphenyl). Optimize redox conditions (Eh < -200 mV) and provide hydrogen as an electron donor .
Q. How can hydroxylated and methoxylated metabolites of this compound be characterized in microbial degradation studies?
- Methodological Answer : Use liquid chromatography-tandem mass spectrometry (LC-MS/MS) with electrospray ionization (ESI) to detect polar metabolites. Synthesize reference standards (e.g., 4′-hydroxy-2,3,4,5-tetrachlorobiphenyl) for quantification. For methoxylated derivatives, employ methylation derivatization followed by GC-MS analysis .
Q. What statistical approaches resolve contradictions in sorption data for this compound across different microplastic polymers?
- Methodological Answer : Apply multivariate regression to account for polymer-specific variables (e.g., crystallinity, surface area). For example, polyethylene (log Kd ≈ 5.7) shows higher sorption than polypropylene (log Kd ≈ 5.2) due to hydrophobic interactions. Normalize data using organic carbon content (e.g., Koc) to enable cross-study comparisons .
Q. How do isomer-specific differences in chlorination patterns affect the design of PCB remediation strategies?
- Methodological Answer : Prioritize isomers based on persistence and toxicity. For this compound, focus on anaerobic bioremediation due to its susceptibility to reductive dechlorination. In contrast, planar congeners (e.g., 3,3',4,4'-tetrachlorobiphenyl) require oxidative methods like UV/Fenton treatment .
Eigenschaften
IUPAC Name |
1,2,3,4-tetrachloro-5-phenylbenzene | |
---|---|---|
Source | PubChem | |
URL | https://pubchem.ncbi.nlm.nih.gov | |
Description | Data deposited in or computed by PubChem | |
InChI |
InChI=1S/C12H6Cl4/c13-9-6-8(7-4-2-1-3-5-7)10(14)12(16)11(9)15/h1-6H | |
Source | PubChem | |
URL | https://pubchem.ncbi.nlm.nih.gov | |
Description | Data deposited in or computed by PubChem | |
InChI Key |
HLQDGCWIOSOMDP-UHFFFAOYSA-N | |
Source | PubChem | |
URL | https://pubchem.ncbi.nlm.nih.gov | |
Description | Data deposited in or computed by PubChem | |
Canonical SMILES |
C1=CC=C(C=C1)C2=CC(=C(C(=C2Cl)Cl)Cl)Cl | |
Source | PubChem | |
URL | https://pubchem.ncbi.nlm.nih.gov | |
Description | Data deposited in or computed by PubChem | |
Molecular Formula |
C12H6Cl4 | |
Source | PubChem | |
URL | https://pubchem.ncbi.nlm.nih.gov | |
Description | Data deposited in or computed by PubChem | |
DSSTOX Substance ID |
DTXSID5074135 | |
Record name | 2,3,4,5-Tetrachlorobiphenyl | |
Source | EPA DSSTox | |
URL | https://comptox.epa.gov/dashboard/DTXSID5074135 | |
Description | DSSTox provides a high quality public chemistry resource for supporting improved predictive toxicology. | |
Molecular Weight |
292.0 g/mol | |
Source | PubChem | |
URL | https://pubchem.ncbi.nlm.nih.gov | |
Description | Data deposited in or computed by PubChem | |
CAS No. |
33284-53-6 | |
Record name | 2,3,4,5-Tetrachlorobiphenyl | |
Source | CAS Common Chemistry | |
URL | https://commonchemistry.cas.org/detail?cas_rn=33284-53-6 | |
Description | CAS Common Chemistry is an open community resource for accessing chemical information. Nearly 500,000 chemical substances from CAS REGISTRY cover areas of community interest, including common and frequently regulated chemicals, and those relevant to high school and undergraduate chemistry classes. This chemical information, curated by our expert scientists, is provided in alignment with our mission as a division of the American Chemical Society. | |
Explanation | The data from CAS Common Chemistry is provided under a CC-BY-NC 4.0 license, unless otherwise stated. | |
Record name | 2,3,4,5-Tetrachlorobiphenyl | |
Source | ChemIDplus | |
URL | https://pubchem.ncbi.nlm.nih.gov/substance/?source=chemidplus&sourceid=0033284536 | |
Description | ChemIDplus is a free, web search system that provides access to the structure and nomenclature authority files used for the identification of chemical substances cited in National Library of Medicine (NLM) databases, including the TOXNET system. | |
Record name | 2,3,4,5-Tetrachlorobiphenyl | |
Source | EPA DSSTox | |
URL | https://comptox.epa.gov/dashboard/DTXSID5074135 | |
Description | DSSTox provides a high quality public chemistry resource for supporting improved predictive toxicology. | |
Record name | 2,3,4,5-TETRACHLOROBIPHENYL | |
Source | FDA Global Substance Registration System (GSRS) | |
URL | https://gsrs.ncats.nih.gov/ginas/app/beta/substances/392EXD3SC9 | |
Description | The FDA Global Substance Registration System (GSRS) enables the efficient and accurate exchange of information on what substances are in regulated products. Instead of relying on names, which vary across regulatory domains, countries, and regions, the GSRS knowledge base makes it possible for substances to be defined by standardized, scientific descriptions. | |
Explanation | Unless otherwise noted, the contents of the FDA website (www.fda.gov), both text and graphics, are not copyrighted. They are in the public domain and may be republished, reprinted and otherwise used freely by anyone without the need to obtain permission from FDA. Credit to the U.S. Food and Drug Administration as the source is appreciated but not required. | |
Retrosynthesis Analysis
AI-Powered Synthesis Planning: Our tool employs the Template_relevance Pistachio, Template_relevance Bkms_metabolic, Template_relevance Pistachio_ringbreaker, Template_relevance Reaxys, Template_relevance Reaxys_biocatalysis model, leveraging a vast database of chemical reactions to predict feasible synthetic routes.
One-Step Synthesis Focus: Specifically designed for one-step synthesis, it provides concise and direct routes for your target compounds, streamlining the synthesis process.
Accurate Predictions: Utilizing the extensive PISTACHIO, BKMS_METABOLIC, PISTACHIO_RINGBREAKER, REAXYS, REAXYS_BIOCATALYSIS database, our tool offers high-accuracy predictions, reflecting the latest in chemical research and data.
Strategy Settings
Precursor scoring | Relevance Heuristic |
---|---|
Min. plausibility | 0.01 |
Model | Template_relevance |
Template Set | Pistachio/Bkms_metabolic/Pistachio_ringbreaker/Reaxys/Reaxys_biocatalysis |
Top-N result to add to graph | 6 |
Feasible Synthetic Routes
Haftungsausschluss und Informationen zu In-Vitro-Forschungsprodukten
Bitte beachten Sie, dass alle Artikel und Produktinformationen, die auf BenchChem präsentiert werden, ausschließlich zu Informationszwecken bestimmt sind. Die auf BenchChem zum Kauf angebotenen Produkte sind speziell für In-vitro-Studien konzipiert, die außerhalb lebender Organismen durchgeführt werden. In-vitro-Studien, abgeleitet von dem lateinischen Begriff "in Glas", beinhalten Experimente, die in kontrollierten Laborumgebungen unter Verwendung von Zellen oder Geweben durchgeführt werden. Es ist wichtig zu beachten, dass diese Produkte nicht als Arzneimittel oder Medikamente eingestuft sind und keine Zulassung der FDA für die Vorbeugung, Behandlung oder Heilung von medizinischen Zuständen, Beschwerden oder Krankheiten erhalten haben. Wir müssen betonen, dass jede Form der körperlichen Einführung dieser Produkte in Menschen oder Tiere gesetzlich strikt untersagt ist. Es ist unerlässlich, sich an diese Richtlinien zu halten, um die Einhaltung rechtlicher und ethischer Standards in Forschung und Experiment zu gewährleisten.