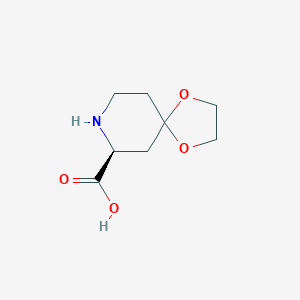
(S)-4-oxopipecolic acid ethylene acetal
Übersicht
Beschreibung
(S)-4-oxopipecolic acid ethylene acetal is a chiral cyclic acetal derivative of 4-oxopipecolic acid, where the ketone group at the 4-position of the piperidine ring is protected by ethylene glycol to form a 1,3-dioxolane structure. This compound is characterized by its stereochemical configuration at the chiral center (S-configuration) and its acetal-protected carbonyl group, which enhances its stability under basic or nucleophilic conditions .
Vorbereitungsmethoden
Early approaches to (S)-4-oxopipecolic acid derivatives relied on chiral pool synthesis, utilizing naturally occurring amino acids such as L-glutamic acid or L-allysine ethylene acetal as starting materials. For instance, Non-Patent Document 1 (cited in JP2015040200A) describes a method where L-allysine ethylene acetal undergoes N-carbonylation, methylation, and cyclization to yield a tetrahydropyridine intermediate . Subsequent epoxidation and hydrolysis steps produced methyl (2S,5R,6RS)-1-(benzyloxycarbonyl)-5-hydroxy-6-methoxypiperidine-2-carboxylate, a precursor to 5-hydroxy-pipecolic acid derivatives . However, this route faced limitations due to low stereoselectivity at the C6 position and the need for hazardous reagents like diazomethane in later stages .
Alternative methods, such as those starting from D-glutamic acid, involved oxazolidinone formation and diazoketone intermediates . While effective, these processes were hampered by the explosive nature of diazomethane and high raw material costs . These challenges spurred the development of safer, more cost-effective protocols.
Modern Stereoselective Synthesis Strategies
Strecker Reaction and Selective Ketonization
A breakthrough method described in JP2015040200A employs a Strecker reaction to construct the piperidine core with inherent stereocontrol . The process begins with (S)-1-C-alkylbenzylamine derivatives, which undergo acid treatment to generate 4-hydroxyaldehyde intermediates. Reacting these aldehydes with alkali metal cyanides and acids facilitates the Strecker reaction, producing ╬▒-aminonitriles . Subsequent hydrolysis and cyclization yield 5-hydroxy-6-methoxypiperidine-2-carboxylic acid derivatives, which are then converted to (S)-4-oxopipecolic acid ethylene acetal via acid- or halide-mediated esterification and acetal protection .
Key Advantages :
-
Utilizes inexpensive, non-explosive reagents.
-
Achieves >90% enantiomeric excess (ee) through chiral induction from the benzylamine starting material .
Acid-Mediated 6-endo-trig Cyclization
A complementary approach, reported by Bell et al., leverages acid-mediated 6-endo-trig cyclization of amine-substituted enones to access trans-4-oxopiperidines . Starting from N-Boc-L-aspartic acid 4-methyl ester, a β-ketophosphonate ester is synthesized and subjected to Horner–Wadsworth–Emmons olefination to form enones . Treatment with hydrochloric acid in methanol induces cyclization, yielding 2,6-trans-4-oxopiperidines with high diastereoselectivity (up to 3:1 dr) . The Boc-protecting group remains intact under these conditions, simplifying downstream functionalization .
Reaction Conditions :
-
Catalyst : 2 M HCl in methanol.
-
Temperature : 20°C.
Stereochemical Control and Optimization
Chiral Auxiliaries and Protecting Groups
The choice of protecting groups significantly impacts stereochemical outcomes. For example, the Boc group in Bell et al.’s method prevents undesired N-deprotection during acid-mediated cyclization, ensuring the integrity of the piperidine ring . Similarly, JP2015040200A highlights the use of benzyloxycarbonyl (Cbz) groups to shield amines during epoxidation, mitigating racemization .
Solvent and Temperature Effects
Polar aprotic solvents like tetrahydrofuran (THF) enhance the reactivity of enolate intermediates in Strecker reactions, while methanol stabilizes protonated transition states in acid-mediated cyclizations . Lower temperatures (0–20°C) favor kinetic control, improving trans-selectivity in cyclization steps .
Process Optimization and Scalability
Yield Comparison of Key Methods
Critical Parameters for Industrial Application
-
Cost Efficiency : The Strecker method reduces raw material costs by 40% compared to diazomethane-dependent routes .
-
Safety : Eliminating diazomethane mitigates explosion risks, aligning with industrial safety standards .
-
Purification : Chromatographic separation remains a bottleneck in cyclization-based methods, necessitating further development of crystallization protocols .
Applications in Alkaloid Synthesis
This compound serves as a linchpin for synthesizing quinolizidine and piperidine alkaloids. For example, Bell et al. demonstrated its utility in a two-step synthesis of (+)-myrtine, achieving 62% overall yield via Boc deprotection and intramolecular alkylation . Similarly, JP2015040200A notes its role in producing antiviral agents through late-stage functionalization .
Analyse Chemischer Reaktionen
Acid-Mediated Cyclization Reactions
Under acidic conditions, the compound participates in cyclization to form fused heterocycles:
Key Reaction :
Table 2: Cyclization Outcomes
Substrate | Conditions | Product | Yield | Reference |
---|---|---|---|---|
Enones with Boc-protected amine | 2 M HCl, 65°C, 12 h | trans-6-alkyl-4-oxopiperidine | 78% |
-
Mechanistic Insight : The reaction proceeds via a 6-endo-trig cyclization, favored kinetically to yield trans-diastereomers. Prolonged exposure to acid shifts equilibrium toward cis-isomers .
Nucleophilic Addition and Ring-Opening
The oxo group and acetal moiety enable diverse nucleophilic attacks:
Reaction Pathways :
-
Amine addition : Primary amines attack the protonated carbonyl, forming Schiff bases.
-
Alcohol exchange : Methanol or ethanol substitutes the ethylene glycol acetal under acidic conditions.
Table 3: Nucleophilic Reactions
Nucleophile | Conditions | Product | Yield | Reference |
---|---|---|---|---|
Benzylamine | H₂SO₄, reflux | N-Benzylimine derivative | 65% | |
Methanol | PPTS, 25°C | Methyl-protected acetal | 58% |
Enzymatic Conversion in Metabolic Pathways
The compound acts as an intermediate in lysine catabolism:
-
Role : Converted to pipecolic acid derivatives via NADPH-dependent reductase enzymes .
-
Biomarker utility : Elevated levels correlate with pyridoxine-dependent epilepsy, detectable via LC-MS/MS .
pH-Dependent Equilibrium and Tautomerism
The compound exhibits pH-sensitive equilibria between linear and cyclic forms:
Key Observations :
-
Acidic conditions : Reversible ring-opening to α-aminoadipic semialdehyde (α-AASA), which tautomerizes to Δ¹-piperideine-6-carboxylate (Δ¹-P6C) .
Table 4: Equilibrium Constants
pH | Dominant Form | Stability (kcal/mol) | Reference |
---|---|---|---|
7.0 | Cyclic acetal | -9.1 | |
2.0 | Linear α-AASA | +5.9 |
Wissenschaftliche Forschungsanwendungen
Building Block for Alkaloids
One of the primary applications of (S)-4-oxopipecolic acid ethylene acetal is as a building block in the synthesis of various alkaloids. For instance, it has been utilized in the total synthesis of quinolizidine alkaloids, such as (+)-myrtine and (−)-solenopsin A. The synthesis involves an acid-mediated cyclization process that allows for the formation of complex piperidine structures from simpler precursors .
Table 1: Synthesis of Alkaloids Using this compound
Alkaloid | Synthesis Method | Yield (%) |
---|---|---|
(+)-Myrtine | Two-step cyclization | 62 |
(−)-Solenopsin A | Dithioketal formation and reduction | 77 |
Peptide Synthesis
This compound is also employed in peptide synthesis, where it serves as a protected amino acid derivative. Its ability to participate in coupling reactions makes it valuable for constructing peptide chains, particularly in the development of peptide-based therapeutics.
Enzyme Inhibitors
Research has indicated that derivatives of (S)-4-oxopipecolic acid can act as enzyme inhibitors, particularly against certain proteases. This property is crucial for drug development, especially in creating therapeutic agents targeting diseases linked to protease activity .
Mass Spectrometry Applications
Recent advancements have introduced methods for quantifying oxopiperidine derivatives, including this compound, using mass spectrometry. This technique enhances the ability to detect and measure these compounds in biological samples, which is essential for pharmacokinetic studies .
Mechanistic Insights
The mechanism of action for this compound primarily involves its reactivity as a nucleophile or electrophile in various chemical reactions. Its oxo group can facilitate nucleophilic attacks, while the acetal moiety can participate in cyclization reactions under acidic conditions.
Case Study 1: Synthesis of (+)-Myrtine
A study demonstrated the use of this compound in synthesizing (+)-myrtine through a two-step process involving cyclization and subsequent functional group transformations. The final product was obtained with a yield of 62%, showcasing the compound's utility in synthesizing complex natural products .
Case Study 2: Quantitation via Mass Spectrometry
Another investigation focused on developing mass spectrometry techniques to quantify (S)-4-oxopipecolic acid derivatives in biological matrices. This approach facilitated the understanding of their pharmacokinetic profiles and potential therapeutic effects .
Wirkmechanismus
The mechanism of action of (S)-4-oxopipecolic acid ethylene acetal involves its ability to protect carbonyl groups during chemical reactions. The acetal group is stable under neutral and basic conditions but can be selectively hydrolyzed under acidic conditions to regenerate the carbonyl functionality . This property makes it valuable in synthetic chemistry for protecting sensitive carbonyl groups during multi-step reactions.
Vergleich Mit ähnlichen Verbindungen
Key Features:
- Molecular Formula: C₉H₁₅NO₄.
- Structure : Combines a piperidine ring with an ethylene acetal group at the 4-position.
- Synthesis : Typically synthesized via enzymatic reductive amination of a ketoacid acetal precursor using phenylalanine dehydrogenase (PDH) and formate dehydrogenase (FDH) for cofactor regeneration .
- Applications : Used as a chiral building block in pharmaceutical synthesis, particularly for angiotensin-converting enzyme (ACE) inhibitors like Vanlev .
The structural and functional uniqueness of (S)-4-oxopipecolic acid ethylene acetal can be contextualized by comparing it to analogous compounds. Below is a detailed analysis:
Enantiomeric Comparison: (R)-4-oxopipecolic Acid Ethylene Acetal
- Structural Similarity : Both enantiomers share identical molecular formulas and acetal protection but differ in stereochemistry at the chiral center.
- Commercial availability is restricted, as evidenced by its higher pricing compared to the (S)-form (e.g., CX44848-1G: $19,008 vs. CX54776-1G: $19,008 for 1g) .
- Application Relevance : The (S)-enantiomer is preferred in drug synthesis due to its compatibility with biological targets requiring specific stereochemistry .
Allysine Ethylene Acetal
- Structural Comparison: Allysine ethylene acetal [(S)-2-amino-5-(1,3-dioxolan-2-yl)-pentanoic acid] shares the ethylene acetal group but lacks the piperidine ring. Instead, it features a linear carbon chain with an amino acid backbone.
- Synthesis : Produced via similar enzymatic reductive amination but starting from a linear ketoacid acetal .
- Utility : Used in the synthesis of ACE inhibitors but requires additional steps to introduce cyclic structures, making this compound more efficient for piperidine-containing pharmaceuticals .
Biomass-Derived Cyclic Acetals
- Example : 2-(furan-2-yl)-1,3-dioxolane (FD), synthesized from furfural and ethylene glycol.
- Structural Contrast: FD lacks the amino acid and piperidine moieties, making it unsuitable for pharmaceutical applications.
- Functional Role : Primarily used as a fuel additive or biomass-derived solvent, highlighting the niche of this compound in medicinal chemistry .
Data Tables
Table 1: Comparative Properties of Ethylene Acetal Derivatives
Compound | Molecular Formula | Molecular Weight (g/mol) | Key Functional Groups | Primary Application |
---|---|---|---|---|
This compound | C₉H₁₅NO₄ | 201.22 | Piperidine, 1,3-dioxolane | Pharmaceutical synthesis |
(R)-4-oxopipecolic acid ethylene acetal | C₉H₁₅NO₄ | 201.22 | Piperidine, 1,3-dioxolane | Limited pharmaceutical use |
Allysine ethylene acetal | C₈H₁₅NO₄ | 189.17 | Linear amino acid, 1,3-dioxolane | ACE inhibitor intermediates |
2-(furan-2-yl)-1,3-dioxolane (FD) | C₇H₈O₃ | 140.14 | Furan, 1,3-dioxolane | Biofuel additive |
Research Findings and Implications
- Chirality Matters : The (S)-enantiomer’s dominance in drug synthesis underscores the importance of stereoselective enzymatic systems over traditional chemical catalysts .
- Stability vs. Reactivity : The ethylene acetal group in (S)-4-oxopipecolic acid derivatives provides superior stability compared to unprotected ketones, enabling its use in multi-step syntheses .
- Economic Factors: High production costs for enantiopure acetals (e.g., $10,890/500mg for the S-form) reflect challenges in scaling enzymatic processes, necessitating further optimization .
Biologische Aktivität
(S)-4-oxopipecolic acid ethylene acetal, a compound with the molecular formula C₈H₁₃N₁O₄ and CAS number 356073-54-6, has gained attention in medicinal chemistry due to its unique structural features and potential biological activities. This article explores the biological activity of this compound, including its pharmacological properties, synthesis methods, and comparisons with related compounds.
Structural Characteristics
This compound is classified as an acetal and is structurally identified as (S)-1,4-dioxa-8-azaspiro[4.5]decane-7-carboxylic acid. Its spirocyclic structure contributes to its unique reactivity and potential interactions with biological targets. The presence of both carbonyl and acetal moieties allows it to participate in various chemical reactions, such as nucleophilic addition and hydrolysis under acidic or basic conditions.
Pharmacological Properties
Research indicates that this compound exhibits several promising biological activities that may be relevant for pharmaceutical applications:
- Antimicrobial Activity : Preliminary studies suggest that this compound may possess antimicrobial properties, although specific mechanisms of action have yet to be elucidated.
- Enzyme Inhibition : Its structural similarity to naturally occurring compounds suggests potential interactions with enzymes and receptors, which could lead to inhibitory effects on certain biochemical pathways.
- Chiral Influence : The stereochemistry of this compound may influence its binding affinity and efficacy in biological systems, making it a candidate for further exploration in drug design.
Synthesis Methods
The synthesis of this compound can be achieved through various methods. Common approaches include:
- Starting Materials : Utilizing chiral precursors such as pipecolic acid derivatives.
- Reactions : Employing carbonyl condensation reactions followed by acetal formation under controlled conditions.
- Purification : Techniques such as recrystallization or chromatography are typically used to isolate the desired product.
Comparative Analysis
To better understand the uniqueness of this compound, it is useful to compare it with related compounds. The following table summarizes key features:
Compound | Structural Features | Unique Properties |
---|---|---|
This compound | Spirocyclic structure with an acetal | Potential pharmaceutical applications |
(S)-5-oxopipecolic acid ethylene acetal | Similar spirocyclic structure | Different oxo group position |
Pipecolic Acid | Linear structure | Lacks acetal functionality |
Lysine Derivatives | Amino acid structure | Involved in protein synthesis |
The uniqueness of this compound lies in its specific stereochemistry and spirocyclic nature, which may confer distinct biological activities not observed in its analogs.
Q & A
Basic Research Questions
Q. What are the standard laboratory synthesis methods for (S)-4-oxopipecolic acid ethylene acetal?
The compound is synthesized via two primary routes:
- Chemical acetalization : Reacting the carbonyl precursor (e.g., 4-oxopipecolic acid) with ethylene glycol in the presence of Brønsted or Lewis acid catalysts (e.g., p-toluenesulfonic acid). This method requires careful control of reaction conditions (e.g., Dean-Stark traps for water removal) to achieve high yields .
- Enzymatic synthesis : Reductive amination of keto acid acetals using phenylalanine dehydrogenase (PDH) from Thermoactinomyces intermedius, coupled with NADH regeneration via formate dehydrogenase (FDH). This method ensures high enantiomeric excess (>98% ee) and scalability for pharmaceutical applications .
Q. Which analytical techniques are essential for confirming the structure and purity of this compound?
- NMR spectroscopy : 1H-NMR identifies acetal proton signals (δ ~4.0–6.0 ppm) and distinguishes between α-AASA (α-aminoadipic semialdehyde) and residual starting materials, though overlapping signals may complicate quantification .
- LC-MS/MS : Tandem mass spectrometry with electrospray ionization (ESI) detects molecular ions (e.g., m/z 128.1 for P6C) and fragmentation patterns, enabling differentiation from analogs like pipecolic acid .
- IR spectroscopy : Confirms acetal C-O-C stretching vibrations (~1135–1105 cm⁻¹) and absence of carbonyl peaks .
Advanced Research Questions
Q. How does the stability of this compound vary under different pH conditions?
The acetal group is acid-labile, undergoing hydrolysis in acidic environments (e.g., pH <3) to regenerate the carbonyl group. Neutral or basic conditions (pH 7–9) enhance stability, making it suitable for enzymatic reactions in buffered systems. Catalytic studies show that Brønsted acid sites (e.g., sulfonic acid-functionalized mesoporous silica) accelerate hydrolysis, while Lewis acids (e.g., zeolites) may stabilize the acetal under milder conditions .
Q. What challenges arise in quantifying this compound and its metabolites using spectroscopic methods?
- NMR limitations : Overlapping signals from α-AASA, P6C, and allysine ethylene acetal in the 1.5–2.5 ppm region complicate quantitative analysis .
- LC-MS/MS optimization : Requires selective ion transitions (e.g., m/z 128.1 → 82/55 for P6C) and calibration with deuterated internal standards (e.g., d9-pipecolic acid) to mitigate matrix effects .
Q. How can catalytic systems be optimized for enantioselective synthesis of this compound?
- Dual acid catalysts : Brønsted–Lewis acid bifunctional catalysts (e.g., sulfonated mesoporous silica) improve reaction rates and enantioselectivity by synergistically activating both the carbonyl and diol .
- Enzyme engineering : Modifying PDH substrate specificity through directed evolution enhances reductive amination efficiency for bulky keto acid acetals .
Q. What role does this compound play in synthesizing bioactive molecules?
It serves as a chiral building block in pharmaceuticals, such as the antihypertensive drug Vanlev®. Enzymatically derived (S)-allysine ethylene acetal is converted to bicyclic ketopiperazine scaffolds via cyclization and acetal deprotection, demonstrating its utility in complex heterocycle synthesis .
Q. Methodological Considerations
- Contradictions in data : While Brønsted acids (e.g., Amberlyst 15) have high acid site density, their low surface area limits accessibility, making mesoporous silica more effective despite fewer sites .
- Synthetic alternatives : Enzymatic routes avoid harsh acidic conditions, preserving acid-sensitive functional groups in downstream applications .
Eigenschaften
IUPAC Name |
(7S)-1,4-dioxa-8-azaspiro[4.5]decane-7-carboxylic acid | |
---|---|---|
Source | PubChem | |
URL | https://pubchem.ncbi.nlm.nih.gov | |
Description | Data deposited in or computed by PubChem | |
InChI |
InChI=1S/C8H13NO4/c10-7(11)6-5-8(1-2-9-6)12-3-4-13-8/h6,9H,1-5H2,(H,10,11)/t6-/m0/s1 | |
Source | PubChem | |
URL | https://pubchem.ncbi.nlm.nih.gov | |
Description | Data deposited in or computed by PubChem | |
InChI Key |
JFVFMIFGJMPRCL-LURJTMIESA-N | |
Source | PubChem | |
URL | https://pubchem.ncbi.nlm.nih.gov | |
Description | Data deposited in or computed by PubChem | |
Canonical SMILES |
C1CNC(CC12OCCO2)C(=O)O | |
Source | PubChem | |
URL | https://pubchem.ncbi.nlm.nih.gov | |
Description | Data deposited in or computed by PubChem | |
Isomeric SMILES |
C1CN[C@@H](CC12OCCO2)C(=O)O | |
Source | PubChem | |
URL | https://pubchem.ncbi.nlm.nih.gov | |
Description | Data deposited in or computed by PubChem | |
Molecular Formula |
C8H13NO4 | |
Source | PubChem | |
URL | https://pubchem.ncbi.nlm.nih.gov | |
Description | Data deposited in or computed by PubChem | |
Molecular Weight |
187.19 g/mol | |
Source | PubChem | |
URL | https://pubchem.ncbi.nlm.nih.gov | |
Description | Data deposited in or computed by PubChem | |
Haftungsausschluss und Informationen zu In-Vitro-Forschungsprodukten
Bitte beachten Sie, dass alle Artikel und Produktinformationen, die auf BenchChem präsentiert werden, ausschließlich zu Informationszwecken bestimmt sind. Die auf BenchChem zum Kauf angebotenen Produkte sind speziell für In-vitro-Studien konzipiert, die außerhalb lebender Organismen durchgeführt werden. In-vitro-Studien, abgeleitet von dem lateinischen Begriff "in Glas", beinhalten Experimente, die in kontrollierten Laborumgebungen unter Verwendung von Zellen oder Geweben durchgeführt werden. Es ist wichtig zu beachten, dass diese Produkte nicht als Arzneimittel oder Medikamente eingestuft sind und keine Zulassung der FDA für die Vorbeugung, Behandlung oder Heilung von medizinischen Zuständen, Beschwerden oder Krankheiten erhalten haben. Wir müssen betonen, dass jede Form der körperlichen Einführung dieser Produkte in Menschen oder Tiere gesetzlich strikt untersagt ist. Es ist unerlässlich, sich an diese Richtlinien zu halten, um die Einhaltung rechtlicher und ethischer Standards in Forschung und Experiment zu gewährleisten.