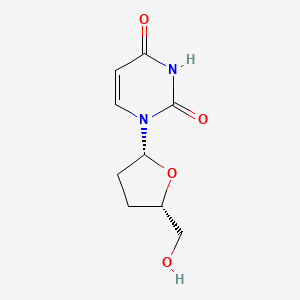
2',3'-Dideoxyuridine
Übersicht
Beschreibung
2',3'-Dideoxyuridine (ddU) is a synthetic nucleoside analog of the natural nucleoside thymidine. It is an important component of antiviral drugs, and is used as an inhibitor of viral replication in a variety of infectious diseases. ddU has been studied extensively in the laboratory and clinical settings, and has been found to be an effective inhibitor of viral replication in HIV, influenza, and herpes simplex virus (HSV) infections.
Wissenschaftliche Forschungsanwendungen
Antiviral Research
2’,3’-Dideoxyuridine is used as a research tool in antiviral studies . It’s a nucleoside analogue, which means it can inhibit viral replication by incorporating into viral DNA and causing premature termination.
Anticancer Studies
In addition to its antiviral properties, 2’,3’-Dideoxyuridine is also used in anticancer studies . It can be incorporated into the DNA of rapidly dividing cancer cells, disrupting their replication process and leading to cell death.
Synthesis of Derivatives
The compound is used in the synthesis of its derivatives like 2’,3’-didehydro-2’,3’-dideoxyuridine (d4U) and 2’,3’-dideoxyuridine phosphoramidate 'ProTide’ . These derivatives are then evaluated against HIV-1 and HIV-2 .
Molecular Modeling Studies
2’,3’-Dideoxyuridine and its derivatives are used in molecular modeling studies to investigate their second phosphorylation process . This helps in understanding the mechanism of action of these compounds.
Wirkmechanismus
Target of Action
2’,3’-Dideoxyuridine is a purine nucleoside analog . Its primary targets are indolent lymphoid malignancies . These targets play a crucial role in the replication and survival of these cells.
Mode of Action
2’,3’-Dideoxyuridine interacts with its targets by inhibiting DNA synthesis . This interaction results in the disruption of the replication process, leading to the death of the targeted cells .
Biochemical Pathways
The primary biochemical pathway affected by 2’,3’-Dideoxyuridine is the DNA synthesis pathway . By inhibiting this pathway, 2’,3’-Dideoxyuridine prevents the replication of the targeted cells, leading to their death .
Pharmacokinetics
It is known that the compound is eliminated mainly by renal excretion . The oral bioavailability of 2’,3’-Dideoxyuridine is approximately 41% . The compound’s ADME properties and their impact on bioavailability are still under investigation.
Result of Action
The primary result of 2’,3’-Dideoxyuridine’s action is the death of the targeted cells . This is achieved through the inhibition of DNA synthesis, which prevents the replication of the cells . Additionally, 2’,3’-Dideoxyuridine can induce apoptosis, or programmed cell death .
Eigenschaften
IUPAC Name |
1-[(2R,5S)-5-(hydroxymethyl)oxolan-2-yl]pyrimidine-2,4-dione | |
---|---|---|
Source | PubChem | |
URL | https://pubchem.ncbi.nlm.nih.gov | |
Description | Data deposited in or computed by PubChem | |
InChI |
InChI=1S/C9H12N2O4/c12-5-6-1-2-8(15-6)11-4-3-7(13)10-9(11)14/h3-4,6,8,12H,1-2,5H2,(H,10,13,14)/t6-,8+/m0/s1 | |
Source | PubChem | |
URL | https://pubchem.ncbi.nlm.nih.gov | |
Description | Data deposited in or computed by PubChem | |
InChI Key |
BTOTXLJHDSNXMW-POYBYMJQSA-N | |
Source | PubChem | |
URL | https://pubchem.ncbi.nlm.nih.gov | |
Description | Data deposited in or computed by PubChem | |
Canonical SMILES |
C1CC(OC1CO)N2C=CC(=O)NC2=O | |
Source | PubChem | |
URL | https://pubchem.ncbi.nlm.nih.gov | |
Description | Data deposited in or computed by PubChem | |
Isomeric SMILES |
C1C[C@@H](O[C@@H]1CO)N2C=CC(=O)NC2=O | |
Source | PubChem | |
URL | https://pubchem.ncbi.nlm.nih.gov | |
Description | Data deposited in or computed by PubChem | |
Molecular Formula |
C9H12N2O4 | |
Source | PubChem | |
URL | https://pubchem.ncbi.nlm.nih.gov | |
Description | Data deposited in or computed by PubChem | |
DSSTOX Substance ID |
DTXSID90208558 | |
Record name | 2',3'-Dideoxyuridine | |
Source | EPA DSSTox | |
URL | https://comptox.epa.gov/dashboard/DTXSID90208558 | |
Description | DSSTox provides a high quality public chemistry resource for supporting improved predictive toxicology. | |
Molecular Weight |
212.20 g/mol | |
Source | PubChem | |
URL | https://pubchem.ncbi.nlm.nih.gov | |
Description | Data deposited in or computed by PubChem | |
CAS RN |
5983-09-5 | |
Record name | 2',3'-Dideoxyuridine | |
Source | ChemIDplus | |
URL | https://pubchem.ncbi.nlm.nih.gov/substance/?source=chemidplus&sourceid=0005983095 | |
Description | ChemIDplus is a free, web search system that provides access to the structure and nomenclature authority files used for the identification of chemical substances cited in National Library of Medicine (NLM) databases, including the TOXNET system. | |
Record name | 2',3'-Dideoxyuridine | |
Source | EPA DSSTox | |
URL | https://comptox.epa.gov/dashboard/DTXSID90208558 | |
Description | DSSTox provides a high quality public chemistry resource for supporting improved predictive toxicology. | |
Retrosynthesis Analysis
AI-Powered Synthesis Planning: Our tool employs the Template_relevance Pistachio, Template_relevance Bkms_metabolic, Template_relevance Pistachio_ringbreaker, Template_relevance Reaxys, Template_relevance Reaxys_biocatalysis model, leveraging a vast database of chemical reactions to predict feasible synthetic routes.
One-Step Synthesis Focus: Specifically designed for one-step synthesis, it provides concise and direct routes for your target compounds, streamlining the synthesis process.
Accurate Predictions: Utilizing the extensive PISTACHIO, BKMS_METABOLIC, PISTACHIO_RINGBREAKER, REAXYS, REAXYS_BIOCATALYSIS database, our tool offers high-accuracy predictions, reflecting the latest in chemical research and data.
Strategy Settings
Precursor scoring | Relevance Heuristic |
---|---|
Min. plausibility | 0.01 |
Model | Template_relevance |
Template Set | Pistachio/Bkms_metabolic/Pistachio_ringbreaker/Reaxys/Reaxys_biocatalysis |
Top-N result to add to graph | 6 |
Feasible Synthetic Routes
Q & A
Q1: What is the primary mechanism of action of 2',3'-dideoxyuridine against retroviruses like HIV?
A1: this compound exerts its antiviral effect by first being phosphorylated intracellularly to its active metabolite, this compound-5'-triphosphate (ddUTP) [, ]. ddUTP then acts as a potent and selective inhibitor of the reverse transcriptase (RT) enzyme of retroviruses like HIV [, , , ]. It competes with the natural substrate, deoxythymidine triphosphate (dTTP), for incorporation into the growing viral DNA chain [, ]. Due to the lack of a 3'-hydroxyl group, ddUTP terminates further DNA chain elongation, ultimately blocking viral replication [, , ].
Q2: Why is this compound itself considered inactive against HIV in cells, despite the potency of its triphosphate form?
A2: Although ddUTP is a potent inhibitor of HIV reverse transcriptase, ddUrd itself displays poor anti-HIV activity in cells [, ]. This is attributed to its inefficient intracellular conversion to the active triphosphate form, primarily due to its low affinity for cellular nucleoside kinases responsible for the initial phosphorylation step [, ].
Q3: What novel metabolic pathways have been discovered for this compound and its analogues?
A4: Research has revealed a previously unrecognized metabolic pathway for this compound and similar analogues involving the formation of 5'-O-diphosphohexose derivatives []. Specifically, this compound-5'-O-diphosphoglucose and this compound-5'-O-diphospho-N-acetylglucosamine were identified in human peripheral blood mononuclear cells and bone marrow cells exposed to radiolabeled this compound []. These findings suggest unique metabolic features of this compound that may contribute to its distinct pharmacological profile.
Q4: What is the molecular formula and molecular weight of this compound?
A4: The molecular formula of this compound is C9H12N2O5, and its molecular weight is 228.20 g/mol.
Q5: How do structural modifications of the this compound scaffold affect its antiviral activity?
A6: Modifications at the 5-position of the uracil ring have been explored, with varying effects on activity: * 5-Alkyl substitutions: Small alkyl groups like methyl (thymidine) and ethyl generally retain or even enhance potency [, , ]. * 5-Halogens: Halogen substitutions, particularly chlorine (FddClUrd) at the 5-position, have demonstrated potent and selective anti-HIV-1 activity [, , ]. * 5-Alkoxymethyl groups: These modifications did not show significant activity against HIV-1 []. * 5-o-Carboranyl: This bulky, lipophilic substitution resulted in weak anti-HIV activity []. * 6-Benzyl group: When combined with a 1-[(2-hydroxyethoxy)methyl] substituent, this modification yielded potent anti-HIV-1 activity [].
Q6: What is the impact of modifications at the 3'-position on the activity of this compound analogues?
A7: The 3'-position is crucial for activity. Replacing the 3'-hydroxyl with: * Azido (AzddU): Retains potent anti-HIV activity, but shows cell type-dependent metabolism [, ]. * Fluoro (FddUrd): Generally enhances potency and selectivity against HIV [, ]. * Amino: Results in loss of significant antiviral activity []. * Isocyano: Leads to loss of anti-HIV activity and increased cytotoxicity compared to the azido analogue []. * Triazol-1-yl: Does not show significant anti-HIV activity [].
Q7: What is the significance of the 5'-hydroxyl group in this compound for its antiviral activity?
A8: The 5'-hydroxyl group is essential for the phosphorylation of this compound to its active triphosphate form []. Modifications at this position generally lead to a significant reduction or complete loss of antiviral activity, highlighting its critical role in the drug's mechanism of action [, ].
Q8: What is the in vitro antiviral activity of this compound and its analogues?
A10: The provided research focuses on the activity of this compound derivatives rather than the parent compound itself. Notably: * 3'-Azido-2',3'-dideoxyuridine (AzddU): Demonstrates potent inhibition of HIV-1 replication in human peripheral blood mononuclear cells, comparable to AZT [, , , ]. * 3'-Fluoro-2',3'-dideoxyuridine (FddUrd) and its 5-halogen derivatives: Exhibit potent anti-HIV-1 activity in MT4 cells, with FddClUrd showing selectivity comparable to AZT []. * 2,5'-Anhydro analogues of AzddU and AZT: Display significant anti-HIV-1 activity, although generally lower than their parent compounds []. * 3'-Amino-2',3'-dideoxy-5-fluorouridine: Shows activity against adenovirus and some Gram-positive bacteria but not against HIV [].
Q9: What strategies have been explored to improve the delivery of this compound monophosphate into cells?
A12: Due to the poor cellular uptake of this compound monophosphate (ddUMP), researchers have explored the use of membrane-permeable prodrugs []. One approach involves masking the phosphate group with lipophilic pivaloyloxymethyl (piv) groups, creating bis(pivaloyloxymethyl) this compound 5'-monophosphate (piv2-ddUMP) []. This prodrug successfully delivered ddUMP into human T cells, leading to the formation of active di- and triphosphate metabolites and exhibiting anti-HIV-1 activity []. This “masked nucleotide” strategy holds promise for overcoming the limitations of poor cellular uptake associated with nucleotide analogues.
Haftungsausschluss und Informationen zu In-Vitro-Forschungsprodukten
Bitte beachten Sie, dass alle Artikel und Produktinformationen, die auf BenchChem präsentiert werden, ausschließlich zu Informationszwecken bestimmt sind. Die auf BenchChem zum Kauf angebotenen Produkte sind speziell für In-vitro-Studien konzipiert, die außerhalb lebender Organismen durchgeführt werden. In-vitro-Studien, abgeleitet von dem lateinischen Begriff "in Glas", beinhalten Experimente, die in kontrollierten Laborumgebungen unter Verwendung von Zellen oder Geweben durchgeführt werden. Es ist wichtig zu beachten, dass diese Produkte nicht als Arzneimittel oder Medikamente eingestuft sind und keine Zulassung der FDA für die Vorbeugung, Behandlung oder Heilung von medizinischen Zuständen, Beschwerden oder Krankheiten erhalten haben. Wir müssen betonen, dass jede Form der körperlichen Einführung dieser Produkte in Menschen oder Tiere gesetzlich strikt untersagt ist. Es ist unerlässlich, sich an diese Richtlinien zu halten, um die Einhaltung rechtlicher und ethischer Standards in Forschung und Experiment zu gewährleisten.