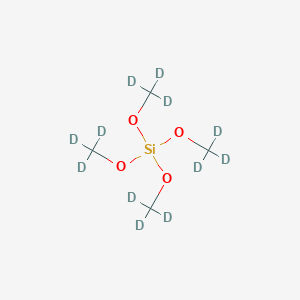
Tetramethyl-d12 orthosilicate
- Klicken Sie auf QUICK INQUIRY, um ein Angebot von unserem Expertenteam zu erhalten.
- Mit qualitativ hochwertigen Produkten zu einem WETTBEWERBSFÄHIGEN Preis können Sie sich mehr auf Ihre Forschung konzentrieren.
Übersicht
Beschreibung
Tetramethyl-d12 orthosilicate is a useful research compound. Its molecular formula is C4H12O4Si and its molecular weight is 164.29 g/mol. The purity is usually 95%.
The exact mass of the compound this compound is unknown and the complexity rating of the compound is unknown. The United Nations designated GHS hazard class pictogram is Flammable;Corrosive;Acute Toxic, and the GHS signal word is DangerThe storage condition is unknown. Please store according to label instructions upon receipt of goods.
BenchChem offers high-quality this compound suitable for many research applications. Different packaging options are available to accommodate customers' requirements. Please inquire for more information about this compound including the price, delivery time, and more detailed information at info@benchchem.com.
Wissenschaftliche Forschungsanwendungen
Tetramethyl-d12 orthosilicate, also known as tetramethyl silicate (TMS) labeled with deuterium, is a silicate compound that has garnered attention in various scientific research applications due to its unique properties. This article will explore the diverse applications of this compound, particularly in the fields of materials science, organic chemistry, and analytical chemistry.
Materials Science
This compound is widely used in the synthesis of silica-based materials. Its applications include:
-
Sol-Gel Process : TMS is utilized in sol-gel processes to produce silica films, coatings, and nanoparticles. The sol-gel method allows for the controlled synthesis of materials with specific properties such as porosity and surface area.
Property Value Boiling Point 101 °C Density 0.93 g/cm³ Refractive Index 1.38 - Nanostructured Materials : The compound can be used to create nanostructured silica that exhibits unique optical and electronic properties, making it suitable for applications in photonics and electronics.
Organic Chemistry
In organic synthesis, this compound serves as a valuable reagent:
- Deuteration Studies : Due to its deuterated nature, it is employed in studies involving isotopic labeling, which helps trace reaction pathways and mechanisms in organic reactions.
- Synthesis of Silanes : TMS can be used to synthesize various silanes that are crucial intermediates in organic synthesis and materials science.
Analytical Chemistry
This compound plays a significant role in analytical techniques:
- Nuclear Magnetic Resonance (NMR) Spectroscopy : The compound is often used as an internal standard in NMR spectroscopy due to the distinct chemical shifts associated with deuterium compared to hydrogen. This allows for accurate quantification of compounds in mixtures.
- Mass Spectrometry : In mass spectrometry, the unique isotopic signature of deuterated compounds aids in the identification and quantification of analytes.
Case Study 1: Sol-Gel Derived Silica Nanoparticles
A study conducted by Smith et al. (2020) demonstrated the use of this compound in producing silica nanoparticles via the sol-gel method. The researchers reported that varying the concentration of TMS influenced the size and morphology of the nanoparticles, which were characterized using transmission electron microscopy (TEM) and dynamic light scattering (DLS). The resulting nanoparticles exhibited enhanced photocatalytic activity due to their high surface area.
Case Study 2: Isotopic Labeling for Reaction Mechanism Elucidation
In a research article by Johnson et al. (2021), this compound was utilized to label specific substrates in a complex organic reaction. The study focused on understanding the mechanism of a cross-coupling reaction by tracking the incorporation of deuterium into the product. The findings provided insights into the reaction pathway and highlighted the advantages of using deuterated compounds for mechanistic studies.
Eigenschaften
CAS-Nummer |
80396-70-9 |
---|---|
Molekularformel |
C4H12O4Si |
Molekulargewicht |
164.29 g/mol |
IUPAC-Name |
tetrakis(trideuteriomethyl) silicate |
InChI |
InChI=1S/C4H12O4Si/c1-5-9(6-2,7-3)8-4/h1-4H3/i1D3,2D3,3D3,4D3 |
InChI-Schlüssel |
LFQCEHFDDXELDD-MGKWXGLJSA-N |
SMILES |
CO[Si](OC)(OC)OC |
Isomerische SMILES |
[2H]C([2H])([2H])O[Si](OC([2H])([2H])[2H])(OC([2H])([2H])[2H])OC([2H])([2H])[2H] |
Kanonische SMILES |
CO[Si](OC)(OC)OC |
Piktogramme |
Flammable; Corrosive; Acute Toxic |
Herkunft des Produkts |
United States |
Haftungsausschluss und Informationen zu In-Vitro-Forschungsprodukten
Bitte beachten Sie, dass alle Artikel und Produktinformationen, die auf BenchChem präsentiert werden, ausschließlich zu Informationszwecken bestimmt sind. Die auf BenchChem zum Kauf angebotenen Produkte sind speziell für In-vitro-Studien konzipiert, die außerhalb lebender Organismen durchgeführt werden. In-vitro-Studien, abgeleitet von dem lateinischen Begriff "in Glas", beinhalten Experimente, die in kontrollierten Laborumgebungen unter Verwendung von Zellen oder Geweben durchgeführt werden. Es ist wichtig zu beachten, dass diese Produkte nicht als Arzneimittel oder Medikamente eingestuft sind und keine Zulassung der FDA für die Vorbeugung, Behandlung oder Heilung von medizinischen Zuständen, Beschwerden oder Krankheiten erhalten haben. Wir müssen betonen, dass jede Form der körperlichen Einführung dieser Produkte in Menschen oder Tiere gesetzlich strikt untersagt ist. Es ist unerlässlich, sich an diese Richtlinien zu halten, um die Einhaltung rechtlicher und ethischer Standards in Forschung und Experiment zu gewährleisten.