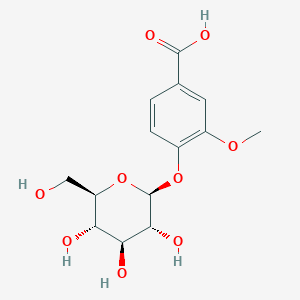
Vanillic acid glucoside
Übersicht
Beschreibung
Vanillic acid glucoside (4-O-β-D-glucopyranosylvanillic acid) is a phenolic compound formed by the glycosylation of vanillic acid (4-hydroxy-3-methoxybenzoic acid) with a glucose moiety via a β-glycosidic bond. It is a key intermediate in the phenylpropanoid pathway, particularly in the biosynthesis of vanillin and related metabolites in plants such as Capsicum frutescens and Vanilla planifolia . Its presence has been confirmed in diverse plant species, including Marrubium vulgare, Lespedeza cuneata, and Helichrysum italicum, often identified through advanced analytical techniques like LC-MS/MS and NMR .
This compound exhibits multiple bioactivities, including antioxidant, antidiabetic, and antimicrobial properties . Additionally, its role in mitigating oxidative stress and inflammation has been documented in both in vitro and in vivo models .
Vorbereitungsmethoden
Synthetic Routes and Reaction Conditions
The synthesis of Vanillic acid glucoside typically involves the glycosylation of 3-methoxybenzoic acid with a suitable glucopyranosyl donor. The reaction is often catalyzed by an acid or enzyme to facilitate the formation of the glycosidic bond. The reaction conditions may include:
Temperature: Typically carried out at room temperature or slightly elevated temperatures.
Solvent: Common solvents include methanol, ethanol, or water.
Catalyst: Acid catalysts such as hydrochloric acid or sulfuric acid, or enzymatic catalysts like glucosidases.
Industrial Production Methods
Industrial production of this compound may involve large-scale extraction from natural sources or chemical synthesis using optimized reaction conditions to ensure high yield and purity. The process may include:
Extraction: Isolation from plant materials using solvents like ethanol or methanol.
Purification: Techniques such as crystallization, chromatography, or recrystallization to obtain the pure compound.
Analyse Chemischer Reaktionen
Types of Reactions
Vanillic acid glucoside undergoes various chemical reactions, including:
Oxidation: The phenolic group can be oxidized to form quinones.
Reduction: The carboxylic acid group can be reduced to form alcohols.
Substitution: The methoxy group can undergo nucleophilic substitution reactions.
Common Reagents and Conditions
Oxidation: Reagents such as potassium permanganate or hydrogen peroxide under acidic or basic conditions.
Reduction: Reagents like lithium aluminum hydride or sodium borohydride.
Substitution: Nucleophiles such as hydroxide ions or amines.
Major Products Formed
Oxidation: Formation of quinones.
Reduction: Formation of alcohol derivatives.
Substitution: Formation of substituted benzoic acid derivatives.
Wissenschaftliche Forschungsanwendungen
Treatment of Autoimmune Diseases
Vanillic acid glucoside has been identified as a promising compound for the treatment of systemic autoimmune diseases. A patent describes its use as an immunosuppressant to treat conditions such as systemic lupus erythematosus (SLE), rheumatoid arthritis, and systemic sclerosis. In pharmacological evaluations, this compound demonstrated significant immunosuppressive activity by inhibiting T lymphocyte proliferation and reducing the levels of anti-ds-DNA antibodies in mouse models of SLE .
Table 1: Effects of this compound on Autoimmune Disease Markers
Parameter | Control Group | This compound (1.1 mg/kg) | This compound (3.3 mg/kg) | Prednisolone (5 mg/kg) |
---|---|---|---|---|
T Lymphocyte Proliferation (%) | 100 | 29 | 57 | 48 |
Anti-ds-DNA Antibody Levels | High | Low | Moderate | Low |
Antiglycation Activity
Research indicates that this compound exhibits significant antiglycation properties, which can be beneficial in managing diabetes and related complications. In vitro studies showed that vanillic acid effectively inhibited glycation of bovine serum albumin (BSA), demonstrating an IC50 value of 45.53 mM . This property is crucial as glycation is implicated in various chronic diseases, including diabetes and neurodegenerative disorders.
Table 2: Antiglycation Activity of Vanillic Acid
Concentration (mM) | Glycation Inhibition (%) |
---|---|
0 | 0 |
50 | 25 |
100 | 45 |
Neuroprotective Effects
This compound has shown potential neuroprotective effects against neuroinflammation induced by lipopolysaccharides (LPS). Studies demonstrated that treatment with vanillic acid significantly reduced markers of inflammation and oxidative stress in mouse models, suggesting its utility in preventing neurodegenerative diseases such as Alzheimer's .
Table 3: Neuroprotective Effects of Vanillic Acid
Treatment | RAGE Expression Reduction (%) | GFAP Activation Reduction (%) |
---|---|---|
Control | - | - |
LPS Only | - | - |
Vanillic Acid + LPS | 60 | 50 |
Food Science Applications
In food science, this compound is recognized for its role as a flavoring agent and preservative due to its antioxidant properties. It can inhibit lipid peroxidation and enhance the shelf life of food products by preventing oxidative damage .
Table 4: Antioxidant Activity of this compound in Food Preservation
Concentration (mg/L) | Lipid Peroxidation Inhibition (%) |
---|---|
0 | 0 |
50 | 30 |
100 | 60 |
Wirkmechanismus
The mechanism of action of Vanillic acid glucoside involves its interaction with various molecular targets and pathways. The phenolic glucoside structure allows it to:
Antioxidant Activity: Scavenge free radicals and reduce oxidative stress by donating hydrogen atoms.
Antimicrobial Activity: Disrupt microbial cell membranes and inhibit enzyme activity.
Anti-inflammatory Activity: Modulate inflammatory pathways by inhibiting pro-inflammatory cytokines and enzymes.
Vergleich Mit ähnlichen Verbindungen
Structural and Functional Comparison with Similar Glucosides
Vanillic acid glucoside belongs to a broader class of phenolic acid glucosides, which share structural similarities but differ in substitution patterns and biological efficacy. Below is a detailed comparison with analogous compounds:
Structural Features
Compound | Molecular Formula | Key Substitutions | Glycosidic Linkage |
---|---|---|---|
This compound | C₁₄H₁₈O₉ | 4-hydroxy, 3-methoxy, glucoside | β-1,4 linkage |
Ferulic acid glucoside | C₁₆H₂₀O₉ | 4-hydroxy, 3-methoxy, propenoic acid | β-1,4 linkage |
p-Coumaric acid glucoside | C₁₅H₁₈O₈ | 4-hydroxy, propenoic acid | β-1,4 linkage |
Syringic acid glucoside | C₁₅H₂₀O₁₀ | 4-hydroxy, 3,5-dimethoxy | β-1,4 linkage |
Ellagic acid glucoside | C₂₀H₁₆O₁₃ | Two lactone rings, hydroxylated | β-1,4 linkage |
Key Insights :
- This compound is distinguished by its single methoxy group at the C3 position, unlike syringic acid glucoside (3,5-dimethoxy) .
- Ferulic acid glucoside contains a propenoic acid side chain, enhancing its radical-scavenging capacity compared to this compound .
Key Insights :
- Ferulic acid glucoside outperforms this compound in antidiabetic assays due to its higher affinity for α-amylase .
- Ellagic acid glucoside shows superior antioxidant activity, attributed to its polyphenolic structure .
Quantitative Occurrence in Natural Sources
Key Insights :
- This compound levels in C. frutescens callus are significantly higher in genetically modified tissues (89.29 µg/g vs. 6.63 µg/g in controls) .
- Its concentration in Lespedeza cuneata is comparable to other antidiabetic phenolics like rosmarinic acid glucoside .
Pharmacological and Industrial Relevance
- Antidiabetic Potential: this compound inhibits α-glucosidase (-9.99 kcal/mol docking score) but is less potent than trans-o-coumaric acid glucoside (-10.3 kcal/mol) .
- Bioaccessibility : Free vanillic acid exhibits 105% bioaccessibility in in vitro models, while its glucoside form requires enzymatic hydrolysis for optimal absorption .
- Industrial Applications : Used as a natural preservative in food and cosmetics due to its stability and antioxidant properties .
Biologische Aktivität
Vanillic acid glucoside, also known as vanillic acid 4-β-D-glucoside, is a phenolic compound that has garnered attention due to its diverse biological activities. This article explores the various effects of this compound, including its antioxidant, anti-inflammatory, and antimicrobial properties, as well as its potential therapeutic applications.
Chemical Structure and Properties
This compound is a hydrolyzable tannin characterized by a glucose moiety linked to vanillic acid. Its chemical formula is and it has a molecular weight of 232.24 g/mol. The compound is soluble in water and exhibits weak acidity, making it an interesting candidate for various biological studies.
1. Antioxidant Activity
This compound demonstrates significant antioxidant properties, which are crucial in combating oxidative stress. Antioxidants neutralize free radicals, thereby preventing cellular damage. Studies have shown that this compound can enhance the activity of endogenous antioxidant enzymes such as superoxide dismutase (SOD) and catalase, contributing to its protective effects against oxidative damage in cells.
2. Anti-inflammatory Effects
Research indicates that this compound can modulate inflammatory responses. A study highlighted its ability to inhibit the expression of pro-inflammatory cytokines such as TNF-α and IL-6 in lipopolysaccharide (LPS)-induced models. This suggests potential applications in managing inflammatory diseases, particularly those associated with neurodegeneration and chronic inflammation.
3. Neuroprotective Properties
This compound has been investigated for its neuroprotective effects against neuroinflammation and neurodegeneration. In animal studies, it was found to mitigate LPS-induced neuroinflammation by reducing the expression of the receptor for advanced glycation end products (RAGE) and associated markers of neuroinflammation such as GFAP and Iba-1. These findings suggest that this compound may be beneficial in treating neurodegenerative diseases like Alzheimer's disease by enhancing memory performance and synaptic integrity .
4. Antimicrobial Activity
The antimicrobial properties of this compound have been documented against various pathogens. Notably, it exhibits potent antibacterial activity against carbapenem-resistant Enterobacter cloacae (CREC). Studies demonstrate that this compound disrupts bacterial cell membranes, leading to decreased intracellular ATP levels and altered membrane potential, which ultimately results in cell death . This property positions it as a potential natural preservative in food systems.
Case Studies
The following table summarizes key findings from various studies on the biological activities of this compound:
The mechanisms underlying the biological activities of this compound involve several pathways:
- Antioxidant Mechanism: this compound scavenges free radicals directly and upregulates antioxidant enzyme expression.
- Anti-inflammatory Pathway: It inhibits NF-κB signaling pathways, leading to reduced cytokine production.
- Neuroprotective Mechanism: By modulating RAGE signaling and reducing oxidative stress, it protects neurons from degeneration.
- Antimicrobial Action: It alters membrane permeability in bacteria, leading to cell lysis and death.
Q & A
Q. What are the primary natural sources of vanillic acid glucoside, and how can extraction efficiency be optimized?
This compound is predominantly found in plant by-products, such as mango peels (16.68 ± 0.42 mg/g) and seeds (15.91 ± 0.77 mg/g), as demonstrated in Mangifera indica L. tissues . Extraction optimization requires solvent selection (e.g., methanol-water mixtures), temperature control (40–60°C), and ultrasound-assisted techniques to enhance yield while preserving structural integrity. Quantification via HPLC with diode-array detection (DAD) is recommended for accurate profiling .
Q. What validated methods exist for synthesizing this compound and confirming its structure?
Chemical synthesis involves oxidizing vanillin-O-protected glucoside using potassium permanganate (KMnO₄), followed by deacetylation. Structural validation employs ¹H- and ¹³C-NMR spectroscopy, comparing chemical shifts to published data (e.g., δ 167.03 ppm for the carboxylic acid group and δ 100–110 ppm for glucoside anomeric protons) . Purity assessment via LC-MS or thin-layer chromatography (TLC) is critical to avoid by-products .
Q. How can advanced analytical techniques resolve challenges in detecting this compound in complex matrices?
Ultra-performance liquid chromatography coupled with tandem mass spectrometry (UPLC-MS/MS) is optimal for detecting this compound in biological samples (e.g., human urine post-bilberry consumption). Key parameters include:
- Column : C18 reversed-phase (2.1 × 100 mm, 1.7 µm).
- Mobile phase : 0.1% formic acid in water/acetonitrile.
- Fragmentation ions : m/z 167.0345 (aglycone) and m/z 123.0450 (demethylated fragment) .
Method validation should include recovery rates (>85%) and limits of detection (LOD < 0.1 µg/mL) .
Q. What molecular mechanisms underlie the bioactivity of this compound in pharmacological studies?
This compound exhibits antioxidant and anti-inflammatory properties via modulation of NF-κB and MAPK pathways. In vitro assays (e.g., DPPH radical scavenging and lipoxygenase inhibition) show dose-dependent activity (IC₅₀: 10–50 µM). Network pharmacology models further identify interactions with TNF-α and COX-2, suggesting therapeutic potential in metabolic disorders .
Q. How can metabolic pathways of this compound in humans be traced using isotopic labeling?
Stable isotope labeling (e.g., ¹³C-glucose) combined with high-resolution mass spectrometry (HRMS) tracks its metabolism. Post-ingestion, glucoside hydrolysis by β-glucosidase releases vanillic acid, which undergoes phase II conjugation (glucuronidation/sulfation) in the liver. Metabolomics studies in urine reveal transient peaks (retention time: 11.07 min) corresponding to intact glucoside and its metabolites .
Q. What genetic engineering strategies enhance this compound production in microbial systems?
Strain engineering in E. coli or S. cerevisiae involves overexpression of UDP-glucosyltransferases (UGTs) and shikimate pathway genes (e.g., aroG). CRISPR-Cas9-mediated knockout of competing pathways (e.g., lignin degradation) increases titers by 3–5 fold. Fed-batch fermentation with optimized carbon/nitrogen ratios achieves yields >1 g/L .
Q. How can contradictory reports on this compound’s phytotoxicity versus bioactivity be reconciled?
Phytotoxicity (e.g., growth inhibition in Arabidopsis thaliana at 100 µM) may arise from reactive oxygen species (ROS) overproduction, while bioactivity in mammals involves ROS scavenging. Comparative studies using standardized protocols (e.g., OECD guidelines for plant assays vs. cell culture models) are essential. Dose-response curves and species-specific enzyme activities (e.g., peroxidases) should be analyzed .
Q. What methodological challenges arise in quantifying this compound across plant tissues?
Matrix interference (e.g., polyphenols in olive leaves) and low natural abundance (<19 mg/kg dry weight) necessitate solid-phase extraction (SPE) for purification. Internal standards (e.g., syringic acid-d₃) improve accuracy. Inter-laboratory variability can be minimized using certified reference materials (CRMs) and harmonized LC-MS protocols .
Eigenschaften
IUPAC Name |
3-methoxy-4-[(2S,3R,4S,5S,6R)-3,4,5-trihydroxy-6-(hydroxymethyl)oxan-2-yl]oxybenzoic acid | |
---|---|---|
Source | PubChem | |
URL | https://pubchem.ncbi.nlm.nih.gov | |
Description | Data deposited in or computed by PubChem | |
InChI |
InChI=1S/C14H18O9/c1-21-8-4-6(13(19)20)2-3-7(8)22-14-12(18)11(17)10(16)9(5-15)23-14/h2-4,9-12,14-18H,5H2,1H3,(H,19,20)/t9-,10-,11+,12-,14-/m1/s1 | |
Source | PubChem | |
URL | https://pubchem.ncbi.nlm.nih.gov | |
Description | Data deposited in or computed by PubChem | |
InChI Key |
JYFOSWJYZIVJPO-YGEZULPYSA-N | |
Source | PubChem | |
URL | https://pubchem.ncbi.nlm.nih.gov | |
Description | Data deposited in or computed by PubChem | |
Canonical SMILES |
COC1=C(C=CC(=C1)C(=O)O)OC2C(C(C(C(O2)CO)O)O)O | |
Source | PubChem | |
URL | https://pubchem.ncbi.nlm.nih.gov | |
Description | Data deposited in or computed by PubChem | |
Isomeric SMILES |
COC1=C(C=CC(=C1)C(=O)O)O[C@H]2[C@@H]([C@H]([C@@H]([C@H](O2)CO)O)O)O | |
Source | PubChem | |
URL | https://pubchem.ncbi.nlm.nih.gov | |
Description | Data deposited in or computed by PubChem | |
Molecular Formula |
C14H18O9 | |
Source | PubChem | |
URL | https://pubchem.ncbi.nlm.nih.gov | |
Description | Data deposited in or computed by PubChem | |
DSSTOX Substance ID |
DTXSID80556358 | |
Record name | 4-(beta-D-Glucopyranosyloxy)-3-methoxybenzoic acid | |
Source | EPA DSSTox | |
URL | https://comptox.epa.gov/dashboard/DTXSID80556358 | |
Description | DSSTox provides a high quality public chemistry resource for supporting improved predictive toxicology. | |
Molecular Weight |
330.29 g/mol | |
Source | PubChem | |
URL | https://pubchem.ncbi.nlm.nih.gov | |
Description | Data deposited in or computed by PubChem | |
CAS No. |
32142-31-7 | |
Record name | 4-(beta-D-Glucopyranosyloxy)-3-methoxybenzoic acid | |
Source | EPA DSSTox | |
URL | https://comptox.epa.gov/dashboard/DTXSID80556358 | |
Description | DSSTox provides a high quality public chemistry resource for supporting improved predictive toxicology. | |
Retrosynthesis Analysis
AI-Powered Synthesis Planning: Our tool employs the Template_relevance Pistachio, Template_relevance Bkms_metabolic, Template_relevance Pistachio_ringbreaker, Template_relevance Reaxys, Template_relevance Reaxys_biocatalysis model, leveraging a vast database of chemical reactions to predict feasible synthetic routes.
One-Step Synthesis Focus: Specifically designed for one-step synthesis, it provides concise and direct routes for your target compounds, streamlining the synthesis process.
Accurate Predictions: Utilizing the extensive PISTACHIO, BKMS_METABOLIC, PISTACHIO_RINGBREAKER, REAXYS, REAXYS_BIOCATALYSIS database, our tool offers high-accuracy predictions, reflecting the latest in chemical research and data.
Strategy Settings
Precursor scoring | Relevance Heuristic |
---|---|
Min. plausibility | 0.01 |
Model | Template_relevance |
Template Set | Pistachio/Bkms_metabolic/Pistachio_ringbreaker/Reaxys/Reaxys_biocatalysis |
Top-N result to add to graph | 6 |
Feasible Synthetic Routes
Haftungsausschluss und Informationen zu In-Vitro-Forschungsprodukten
Bitte beachten Sie, dass alle Artikel und Produktinformationen, die auf BenchChem präsentiert werden, ausschließlich zu Informationszwecken bestimmt sind. Die auf BenchChem zum Kauf angebotenen Produkte sind speziell für In-vitro-Studien konzipiert, die außerhalb lebender Organismen durchgeführt werden. In-vitro-Studien, abgeleitet von dem lateinischen Begriff "in Glas", beinhalten Experimente, die in kontrollierten Laborumgebungen unter Verwendung von Zellen oder Geweben durchgeführt werden. Es ist wichtig zu beachten, dass diese Produkte nicht als Arzneimittel oder Medikamente eingestuft sind und keine Zulassung der FDA für die Vorbeugung, Behandlung oder Heilung von medizinischen Zuständen, Beschwerden oder Krankheiten erhalten haben. Wir müssen betonen, dass jede Form der körperlichen Einführung dieser Produkte in Menschen oder Tiere gesetzlich strikt untersagt ist. Es ist unerlässlich, sich an diese Richtlinien zu halten, um die Einhaltung rechtlicher und ethischer Standards in Forschung und Experiment zu gewährleisten.