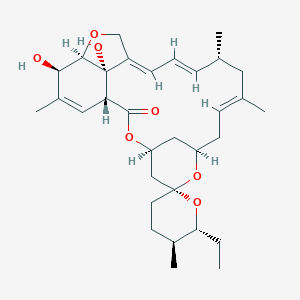
Milbemycin A4
Übersicht
Beschreibung
Milbemycin A4 belongs to a group of 16-membered macrolide antibiotics. It is produced by several Streptomyces species, including Streptomyces bingchenggensis. These compounds exhibit excellent acaricidal, insecticidal, and anthelmintic activities. Due to their low toxicity in mammals, milbemycins and their derivatives find widespread use in agriculture, medicine, and veterinary fields .
Vorbereitungsmethoden
Die synthetischen Wege für Milbemycin A4 umfassen komplexe biosynthetische Pfade. Industrielle Produktionsverfahren basieren in der Regel auf Fermentationsprozessen unter Verwendung von Milbemycin-produzierenden Stämmen. Spezifische Reaktionsbedingungen und Stammbildungstechniken wie zufällige Mutagenese, Stoffwechseltechnik und kombinatorische Biosynthese tragen zur Produktion von Milbemycinen und ihren Derivaten bei .
Analyse Chemischer Reaktionen
Milbemycin A4 unterliegt verschiedenen Reaktionen, darunter Oxidation, Reduktion und Substitution. Häufige Reagenzien und Bedingungen, die bei diesen Reaktionen verwendet werden, tragen zur Bildung von Hauptprodukten bei. Detaillierte Studien zu diesen Reaktionen sind unerlässlich, um Produktionsprozesse zu optimieren und die Ausbeute zu erhöhen.
Wissenschaftliche Forschungsanwendungen
Milbemycin A4 hat vielfältige Anwendungen:
Akarizide und Insektizide: Zur Bekämpfung von Milben und Insekten in der Landwirtschaft.
Anthelmintika: Wirksam gegen parasitäre Würmer.
Einzigartige Wirkungsweise: Milbemycine zielen auf das Nervensystem von Insekten, wodurch sie sich von anderen Verbindungen unterscheiden.
5. Wirkmechanismus
This compound entfaltet seine Wirkung durch Bindung an Glutamat-gesteuerte Chloridkanäle. Diese Interaktion stört den Ionenfluss, was zu Lähmung und Tod bei Parasiten führt. Das Verständnis dieses Mechanismus ist entscheidend für die Optimierung der therapeutischen Anwendung.
Wirkmechanismus
Milbemycin A4 exerts its effects by binding to glutamate-gated chloride channels. This interaction disrupts ion flow, leading to paralysis and death in parasites. Understanding this mechanism is crucial for optimizing therapeutic use.
Vergleich Mit ähnlichen Verbindungen
Milbemycin A4 weist Ähnlichkeiten mit Avermectinen auf, einer anderen Gruppe von Makrolid-Antibiotika. Seine einzigartige Wirkungsweise und sein günstiges Umweltprofil heben es hervor. Zu ähnlichen Verbindungen gehören Milbemycin A3 und andere Milbemycin-Derivate .
Biologische Aktivität
Milbemycin A4 is a potent compound belonging to the milbemycin family, which exhibits significant biological activity against various pathogens, particularly in the context of antifungal and antiparasitic applications. This article explores the biological activity of this compound, focusing on its mechanisms of action, efficacy against specific pathogens, and recent research findings.
This compound functions primarily as an inhibitor of drug efflux in pathogenic fungi, particularly in Candida glabrata. The compound enhances the susceptibility of resistant strains to azole antifungals by blocking the action of ATP-binding cassette (ABC) transporters such as CgCDR1 and PDH1. These transporters are responsible for the efflux of azole drugs, which is a major mechanism of resistance in fungal pathogens. Studies have shown that this compound can decrease the minimum inhibitory concentration (MIC) of fluconazole and voriconazole by fourfold in clinical isolates of C. glabrata .
Table 1: Effect of this compound on Azole Susceptibility
Pathogen | Azole Drug | MIC Reduction (fold) | Concentration of this compound (µg/ml) |
---|---|---|---|
C. glabrata | Fluconazole | 4 | 2.5 |
C. glabrata | Voriconazole | 4 | 2.5 |
Efficacy Against Fungal Pathogens
Research indicates that this compound not only enhances the efficacy of existing antifungals but also possesses intrinsic antifungal properties. In a study involving 28 clinical isolates of C. glabrata, this compound demonstrated significant inhibition of drug efflux, thereby increasing azole susceptibility and potentially leading to improved treatment outcomes for patients with drug-resistant infections .
Case Study: Clinical Isolates
In a study conducted by Silva et al., this compound was tested on various clinical isolates of C. glabrata. The results showed that at a concentration of 2.5 µg/ml, this compound effectively blocked the efflux of radiolabeled fluconazole and rhodamine 6G in azole-resistant strains, confirming its role as an efflux inhibitor . This effect was not observed in strains with deleted efflux pumps, indicating that its action is transporter-specific.
Synergistic Effects with Other Antifungals
This compound has been reported to exhibit synergistic effects when used in combination with other antifungal agents. For instance, studies have shown that it can enhance the efficacy of azoles against Candida albicans and Candida krusei, suggesting a potential for broader application in antifungal therapy .
Antiparasitic Activity
Beyond its antifungal properties, this compound also shows significant activity against various parasites. It has been utilized as a veterinary drug to combat nematodes and ectoparasites in livestock and pets. The compound's mechanism involves disrupting neuromuscular function in parasites, leading to paralysis and death .
Eigenschaften
IUPAC Name |
(1R,4S,5'S,6R,6'R,8R,10E,13R,14E,16E,20R,21R,24S)-6'-ethyl-21,24-dihydroxy-5',11,13,22-tetramethylspiro[3,7,19-trioxatetracyclo[15.6.1.14,8.020,24]pentacosa-10,14,16,22-tetraene-6,2'-oxane]-2-one | |
---|---|---|
Source | PubChem | |
URL | https://pubchem.ncbi.nlm.nih.gov | |
Description | Data deposited in or computed by PubChem | |
InChI |
InChI=1S/C32H46O7/c1-6-27-21(4)12-13-31(39-27)17-25-16-24(38-31)11-10-20(3)14-19(2)8-7-9-23-18-36-29-28(33)22(5)15-26(30(34)37-25)32(23,29)35/h7-10,15,19,21,24-29,33,35H,6,11-14,16-18H2,1-5H3/b8-7+,20-10+,23-9+/t19-,21-,24+,25-,26-,27+,28+,29+,31+,32+/m0/s1 | |
Source | PubChem | |
URL | https://pubchem.ncbi.nlm.nih.gov | |
Description | Data deposited in or computed by PubChem | |
InChI Key |
VOZIAWLUULBIPN-LRBNAKOISA-N | |
Source | PubChem | |
URL | https://pubchem.ncbi.nlm.nih.gov | |
Description | Data deposited in or computed by PubChem | |
Canonical SMILES |
CCC1C(CCC2(O1)CC3CC(O2)CC=C(CC(C=CC=C4COC5C4(C(C=C(C5O)C)C(=O)O3)O)C)C)C | |
Source | PubChem | |
URL | https://pubchem.ncbi.nlm.nih.gov | |
Description | Data deposited in or computed by PubChem | |
Isomeric SMILES |
CC[C@@H]1[C@H](CC[C@@]2(O1)C[C@@H]3C[C@H](O2)C/C=C(/C[C@H](/C=C/C=C/4\CO[C@H]5[C@@]4([C@@H](C=C([C@H]5O)C)C(=O)O3)O)C)\C)C | |
Source | PubChem | |
URL | https://pubchem.ncbi.nlm.nih.gov | |
Description | Data deposited in or computed by PubChem | |
Molecular Formula |
C32H46O7 | |
Source | PubChem | |
URL | https://pubchem.ncbi.nlm.nih.gov | |
Description | Data deposited in or computed by PubChem | |
DSSTOX Substance ID |
DTXSID4052760 | |
Record name | Milbemectin A4 | |
Source | EPA DSSTox | |
URL | https://comptox.epa.gov/dashboard/DTXSID4052760 | |
Description | DSSTox provides a high quality public chemistry resource for supporting improved predictive toxicology. | |
Molecular Weight |
542.7 g/mol | |
Source | PubChem | |
URL | https://pubchem.ncbi.nlm.nih.gov | |
Description | Data deposited in or computed by PubChem | |
CAS No. |
51596-11-3 | |
Record name | Milbemycin A4 | |
Source | CAS Common Chemistry | |
URL | https://commonchemistry.cas.org/detail?cas_rn=51596-11-3 | |
Description | CAS Common Chemistry is an open community resource for accessing chemical information. Nearly 500,000 chemical substances from CAS REGISTRY cover areas of community interest, including common and frequently regulated chemicals, and those relevant to high school and undergraduate chemistry classes. This chemical information, curated by our expert scientists, is provided in alignment with our mission as a division of the American Chemical Society. | |
Explanation | The data from CAS Common Chemistry is provided under a CC-BY-NC 4.0 license, unless otherwise stated. | |
Record name | Milbemycin A4 | |
Source | ChemIDplus | |
URL | https://pubchem.ncbi.nlm.nih.gov/substance/?source=chemidplus&sourceid=0051596113 | |
Description | ChemIDplus is a free, web search system that provides access to the structure and nomenclature authority files used for the identification of chemical substances cited in National Library of Medicine (NLM) databases, including the TOXNET system. | |
Record name | Milbemectin A4 | |
Source | EPA DSSTox | |
URL | https://comptox.epa.gov/dashboard/DTXSID4052760 | |
Description | DSSTox provides a high quality public chemistry resource for supporting improved predictive toxicology. | |
Record name | MILBEMYCIN A4 | |
Source | FDA Global Substance Registration System (GSRS) | |
URL | https://gsrs.ncats.nih.gov/ginas/app/beta/substances/9BSH89E55Y | |
Description | The FDA Global Substance Registration System (GSRS) enables the efficient and accurate exchange of information on what substances are in regulated products. Instead of relying on names, which vary across regulatory domains, countries, and regions, the GSRS knowledge base makes it possible for substances to be defined by standardized, scientific descriptions. | |
Explanation | Unless otherwise noted, the contents of the FDA website (www.fda.gov), both text and graphics, are not copyrighted. They are in the public domain and may be republished, reprinted and otherwise used freely by anyone without the need to obtain permission from FDA. Credit to the U.S. Food and Drug Administration as the source is appreciated but not required. | |
Retrosynthesis Analysis
AI-Powered Synthesis Planning: Our tool employs the Template_relevance Pistachio, Template_relevance Bkms_metabolic, Template_relevance Pistachio_ringbreaker, Template_relevance Reaxys, Template_relevance Reaxys_biocatalysis model, leveraging a vast database of chemical reactions to predict feasible synthetic routes.
One-Step Synthesis Focus: Specifically designed for one-step synthesis, it provides concise and direct routes for your target compounds, streamlining the synthesis process.
Accurate Predictions: Utilizing the extensive PISTACHIO, BKMS_METABOLIC, PISTACHIO_RINGBREAKER, REAXYS, REAXYS_BIOCATALYSIS database, our tool offers high-accuracy predictions, reflecting the latest in chemical research and data.
Strategy Settings
Precursor scoring | Relevance Heuristic |
---|---|
Min. plausibility | 0.01 |
Model | Template_relevance |
Template Set | Pistachio/Bkms_metabolic/Pistachio_ringbreaker/Reaxys/Reaxys_biocatalysis |
Top-N result to add to graph | 6 |
Feasible Synthetic Routes
Q1: What is the primary target of Milbemycin A4 and how does it exert its effect?
A1: this compound primarily targets glutamate-gated chloride channels (GluCls) in invertebrates like mites and insects. [] It acts as an agonist, binding to these channels and causing prolonged channel opening. This leads to an influx of chloride ions, hyperpolarization of nerve and muscle cells, and ultimately paralysis and death of the target organism. []
Q2: What is the molecular formula and weight of this compound?
A2: The molecular formula of this compound is C48H72O14 and its molecular weight is 873.07 g/mol.
Q3: Is there any spectroscopic data available for this compound?
A3: Yes, various spectroscopic techniques have been employed to characterize this compound. This includes Nuclear Magnetic Resonance (NMR) spectroscopy, Infrared (IR) spectroscopy, and Mass Spectrometry (MS). [] These techniques provide detailed information about the compound's structure and properties.
Q4: How do structural modifications of this compound influence its acaricidal activity?
A4: Research has demonstrated that even subtle structural modifications can significantly impact this compound's acaricidal activity. For instance, introducing a methyl group at the 24a position (24a-methylthis compound) or the 25b position (25b-methylthis compound) led to compounds with enhanced activity compared to the parent this compound. [, ] Other modifications like epoxidation of the 8,9-double bond and subsequent acylation at the 5-O position also resulted in improved activity against the two-spotted spider mite (Tetranychus urticae). [] Conversely, certain modifications, like those at the C-5 position, can diminish the anthelmintic activity, indicating the importance of this hydroxyl group for biological activity. []
Q5: Are there any studies on the synthesis of this compound derivatives with improved activity?
A5: Yes, numerous studies have focused on synthesizing this compound derivatives with enhanced activity. For instance, researchers have synthesized 13-alkoxymilbemycin derivatives, with the 13β-phenethyloxy derivatives exhibiting potent anthelmintic activity, even surpassing Ivermectin in some cases. [] Similarly, 5-keto-5-oxime derivatives of this compound have demonstrated higher efficacy against microfilariae compared to the parent compound. []
Q6: Are there any known resistance mechanisms to this compound in target organisms?
A6: Yes, resistance to this compound has emerged in several pest species, including the two-spotted spider mite (Tetranychus urticae). A key mechanism involves mutations in the glutamate-gated chloride channel (GluCl) subunits, particularly a G326E substitution in the TuGluCl3 subunit. This mutation significantly reduces the sensitivity of the channel to this compound, contributing to resistance. []
Q7: Which microorganisms are known to produce this compound?
A7: Streptomyces hygroscopicus subsp. aureolacrimosus is a well-known producer of this compound. [, , ] Additionally, Streptomyces sp. BB47 has been identified as a potential source of this compound, with its production being activated by the introduction of a functional bldA gene. []
Q8: Can microorganisms be used to modify this compound and generate novel derivatives?
A8: Yes, several studies have explored the microbial conversion of this compound to generate novel derivatives with potentially improved or altered activities. Various microorganisms, including Cunninghamella echinulata ATCC 9244, Circinella umbellata SANK 44272, Amycolata autotrophica subsp. amethystina ATCC 35204, and Syncephalastrum racemosum, have been shown to hydroxylate this compound at different positions. [, , , ] These microbial transformations provide a valuable tool for diversifying the chemical structure of this compound and exploring its structure-activity relationships.
Haftungsausschluss und Informationen zu In-Vitro-Forschungsprodukten
Bitte beachten Sie, dass alle Artikel und Produktinformationen, die auf BenchChem präsentiert werden, ausschließlich zu Informationszwecken bestimmt sind. Die auf BenchChem zum Kauf angebotenen Produkte sind speziell für In-vitro-Studien konzipiert, die außerhalb lebender Organismen durchgeführt werden. In-vitro-Studien, abgeleitet von dem lateinischen Begriff "in Glas", beinhalten Experimente, die in kontrollierten Laborumgebungen unter Verwendung von Zellen oder Geweben durchgeführt werden. Es ist wichtig zu beachten, dass diese Produkte nicht als Arzneimittel oder Medikamente eingestuft sind und keine Zulassung der FDA für die Vorbeugung, Behandlung oder Heilung von medizinischen Zuständen, Beschwerden oder Krankheiten erhalten haben. Wir müssen betonen, dass jede Form der körperlichen Einführung dieser Produkte in Menschen oder Tiere gesetzlich strikt untersagt ist. Es ist unerlässlich, sich an diese Richtlinien zu halten, um die Einhaltung rechtlicher und ethischer Standards in Forschung und Experiment zu gewährleisten.