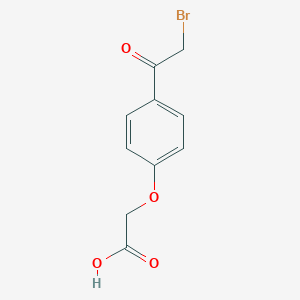
PTP-Inhibitor III
Übersicht
Beschreibung
PTP Inhibitor III is a cell-permeable compound that inhibits a broad range of protein tyrosine phosphatases. Protein tyrosine phosphatases are enzymes that remove phosphate groups from phosphorylated tyrosine residues on proteins, playing a crucial role in regulating cellular signaling pathways. PTP Inhibitor III is particularly significant in research due to its ability to modulate these pathways, making it a valuable tool in the study of various diseases, including cancer and diabetes .
Wissenschaftliche Forschungsanwendungen
PTP-Inhibitor III hat eine breite Palette an Anwendungen in der wissenschaftlichen Forschung, darunter:
Chemie: Wird als Werkzeug verwendet, um die Hemmung von Proteintyrosinphosphatasen und ihre Rolle in zellulären Signalwegen zu untersuchen.
Biologie: Hilft beim Verständnis der Regulation zellulärer Prozesse wie Zellwachstum, -differenzierung und -apoptose.
Medizin: Wird auf seine potenziellen therapeutischen Anwendungen bei der Behandlung von Krankheiten wie Krebs, Diabetes und Autoimmunerkrankungen untersucht, indem die Aktivität von Proteintyrosinphosphatasen moduliert wird
Industrie: Wird bei der Entwicklung neuer Medikamente und therapeutischer Mittel eingesetzt, die auf Proteintyrosinphosphatasen abzielen.
5. Wirkmechanismus
This compound wirkt als photoreversibler kovalenter Inhibitor von Proteintyrosinphosphatasen. Er bindet an die katalytische Domäne des Enzyms und zielt dabei speziell auf das aktive Zentrum des Cysteinrests. Diese Bindung ist reversibel mit Bestrahlung bei 350 nm, wodurch eine kontrollierte Hemmung des Enzyms ermöglicht wird. Die Hemmung von Proteintyrosinphosphatasen durch this compound führt zu veränderten zellulären Signalwegen, die Prozesse wie Zellwachstum und -differenzierung beeinflussen .
Ähnliche Verbindungen:
Vanadiumverbindungen: Diese Verbindungen hemmen ebenfalls Proteintyrosinphosphatasen, jedoch durch andere Mechanismen, die häufig die Nachahmung von Phosphatgruppen beinhalten.
Bisperoxo (1,10-Phenanthrolin)oxidovanadat (V): Ein weiterer Inhibitor, der auf Proteintyrosinphosphatasen abzielt, jedoch mit einer unterschiedlichen chemischen Struktur und Wirkungsweise.
Bis (Maltolat)oxidovanadium (IV): Bekannt für sein Potenzial bei der Behandlung von Diabetes und Krebs durch Hemmung von Proteintyrosinphosphatasen.
Einzigartigkeit von this compound: this compound ist einzigartig aufgrund seines photoreversiblen kovalenten Hemmmechanismus, der eine präzise Kontrolle über die Enzymaktivität ermöglicht. Dieses Merkmal macht es besonders wertvoll in Forschungsumgebungen, in denen die zeitliche Kontrolle der Enzyminhibition entscheidend ist.
Wirkmechanismus
Target of Action
PTP Inhibitor III, also known as 2-[4-(2-bromoacetyl)phenoxy]acetic Acid or 4-(bromoacetyl)phenoxyacetic acid, primarily targets Protein Tyrosine Phosphatases (PTPs) . PTPs are enzymes that remove phosphate groups from phosphorylated tyrosine residues on proteins . They play a crucial role in cellular signaling and are involved in various physiological processes .
Mode of Action
PTP Inhibitor III acts as an inhibitor of PTPs . It binds to the active site of the PTPs, thereby preventing them from dephosphorylating their substrate proteins . This inhibition can alter the phosphorylation state of various proteins, affecting the signaling pathways they are involved in .
Biochemical Pathways
The inhibition of PTPs by PTP Inhibitor III affects several biochemical pathways. PTPs are involved in numerous signaling pathways, including those related to cell growth, differentiation, and metabolism . By inhibiting PTPs, PTP Inhibitor III can potentially affect these pathways, leading to changes in cellular functions .
Pharmacokinetics
The effectiveness of any drug is influenced by its pharmacokinetic properties, which determine the drug’s bioavailability, or the extent and rate at which the active moiety (drug or metabolite) enters systemic circulation, thereby accessing the site of action .
Result of Action
The inhibition of PTPs by PTP Inhibitor III can lead to various molecular and cellular effects. For instance, it can alter the phosphorylation state of proteins, affecting their activity and the cellular functions they regulate . This can have potential therapeutic implications in diseases where PTPs are known to play a role, such as cancer, diabetes, and autoimmune disorders .
Action Environment
The action, efficacy, and stability of PTP Inhibitor III can be influenced by various environmental factors. These can include the presence of other molecules, pH, temperature, and the specific cellular environment . .
Biochemische Analyse
Biochemical Properties
PTP Inhibitor III plays a significant role in biochemical reactions. It binds the catalytic domain of SHP-1 and covalently reacts with free thiols . This reaction is reversible with irradiation . It inhibits a broad range of PTPs , which are enzymes that remove phosphate from a substrate, resulting in a phosphate ion and a hydroxyl group on the substrate .
Cellular Effects
PTP Inhibitor III has profound effects on various types of cells and cellular processes. It influences cell function by balancing the activation of signaling proteins in vascular cells . This helps keep the cells functioning in a normal state .
Molecular Mechanism
At the molecular level, PTP Inhibitor III exerts its effects through binding interactions with biomolecules and enzyme inhibition. It binds covalently to the active site of the enzyme . This binding interaction is crucial for its inhibitory action on PTPs .
Metabolic Pathways
PTP Inhibitor III is involved in metabolic pathways that involve PTPs
Vorbereitungsmethoden
Synthetic Routes and Reaction Conditions: PTP Inhibitor III, formally known as 2-[4-(2-bromoacetyl)phenoxy]-acetic acid, is synthesized through a series of chemical reactionsThe reaction conditions often require the use of solvents like dimethylformamide or dimethyl sulfoxide and catalysts to facilitate the reactions .
Industrial Production Methods: While specific industrial production methods for PTP Inhibitor III are not extensively documented, the general approach involves scaling up the laboratory synthesis process. This includes optimizing reaction conditions to ensure high yield and purity, as well as implementing safety measures to handle the reactive intermediates and final product.
Analyse Chemischer Reaktionen
Arten von Reaktionen: PTP-Inhibitor III durchläuft verschiedene Arten von chemischen Reaktionen, darunter:
Oxidation: Die Verbindung kann unter bestimmten Bedingungen oxidiert werden, wodurch sich ihre chemische Struktur und möglicherweise ihre inhibitorische Aktivität verändern.
Reduktion: Reduktionsreaktionen können das Bromatom modifizieren und die Reaktivität der Verbindung beeinflussen.
Häufige Reagenzien und Bedingungen:
Oxidation: Häufige Oxidationsmittel sind Wasserstoffperoxid und Kaliumpermanganat.
Reduktion: Reduktionsmittel wie Natriumborhydrid oder Lithiumaluminiumhydrid werden häufig verwendet.
Substitution: Nukleophile Reagenzien wie Amine oder Thiole können verwendet werden, um das Bromatom zu ersetzen.
Hauptprodukte, die gebildet werden: Die Hauptprodukte, die aus diesen Reaktionen entstehen, hängen von den verwendeten spezifischen Reagenzien und Bedingungen ab. Beispielsweise können Substitutionsreaktionen eine Vielzahl von Derivaten ergeben, bei denen das Bromatom durch verschiedene funktionelle Gruppen ersetzt wird.
Vergleich Mit ähnlichen Verbindungen
Vanadium Compounds: These compounds also inhibit protein tyrosine phosphatases but through different mechanisms, often involving the mimicry of phosphate groups.
Bisperoxo (1,10-phenanthroline)oxidovanadate (V): Another inhibitor that targets protein tyrosine phosphatases, but with a distinct chemical structure and mode of action.
Bis (maltolato)oxidovanadium (IV): Known for its potential in treating diabetes and cancer by inhibiting protein tyrosine phosphatases.
Uniqueness of PTP Inhibitor III: PTP Inhibitor III is unique due to its photoreversible covalent inhibition mechanism, allowing for precise control over enzyme activity. This feature makes it particularly valuable in research settings where temporal control of enzyme inhibition is crucial.
Eigenschaften
IUPAC Name |
2-[4-(2-bromoacetyl)phenoxy]acetic acid | |
---|---|---|
Source | PubChem | |
URL | https://pubchem.ncbi.nlm.nih.gov | |
Description | Data deposited in or computed by PubChem | |
InChI |
InChI=1S/C10H9BrO4/c11-5-9(12)7-1-3-8(4-2-7)15-6-10(13)14/h1-4H,5-6H2,(H,13,14) | |
Source | PubChem | |
URL | https://pubchem.ncbi.nlm.nih.gov | |
Description | Data deposited in or computed by PubChem | |
InChI Key |
RLSUZPAAQRBGTM-UHFFFAOYSA-N | |
Source | PubChem | |
URL | https://pubchem.ncbi.nlm.nih.gov | |
Description | Data deposited in or computed by PubChem | |
Canonical SMILES |
C1=CC(=CC=C1C(=O)CBr)OCC(=O)O | |
Source | PubChem | |
URL | https://pubchem.ncbi.nlm.nih.gov | |
Description | Data deposited in or computed by PubChem | |
Molecular Formula |
C10H9BrO4 | |
Source | PubChem | |
URL | https://pubchem.ncbi.nlm.nih.gov | |
Description | Data deposited in or computed by PubChem | |
Molecular Weight |
273.08 g/mol | |
Source | PubChem | |
URL | https://pubchem.ncbi.nlm.nih.gov | |
Description | Data deposited in or computed by PubChem | |
Synthesis routes and methods
Procedure details
Retrosynthesis Analysis
AI-Powered Synthesis Planning: Our tool employs the Template_relevance Pistachio, Template_relevance Bkms_metabolic, Template_relevance Pistachio_ringbreaker, Template_relevance Reaxys, Template_relevance Reaxys_biocatalysis model, leveraging a vast database of chemical reactions to predict feasible synthetic routes.
One-Step Synthesis Focus: Specifically designed for one-step synthesis, it provides concise and direct routes for your target compounds, streamlining the synthesis process.
Accurate Predictions: Utilizing the extensive PISTACHIO, BKMS_METABOLIC, PISTACHIO_RINGBREAKER, REAXYS, REAXYS_BIOCATALYSIS database, our tool offers high-accuracy predictions, reflecting the latest in chemical research and data.
Strategy Settings
Precursor scoring | Relevance Heuristic |
---|---|
Min. plausibility | 0.01 |
Model | Template_relevance |
Template Set | Pistachio/Bkms_metabolic/Pistachio_ringbreaker/Reaxys/Reaxys_biocatalysis |
Top-N result to add to graph | 6 |
Feasible Synthetic Routes
Q1: How does PTP Inhibitor III modulate TRPV1 activity in the context of inflammatory pain?
A: PTP Inhibitor III is a non-selective inhibitor of protein tyrosine phosphatases (PTPs). [] Research suggests that it indirectly enhances the activity of TRPV1, a receptor heavily implicated in pain signaling. The mechanism involves inhibiting the activity of SHP-1, a specific PTP that normally dephosphorylates and downregulates TRPV1. By inhibiting SHP-1, PTP Inhibitor III allows for increased tyrosine phosphorylation of TRPV1, leading to its sensitization and amplified pain signaling. [] This effect was observed both in vitro, using cultured DRG neurons, and in vivo, where intrathecal administration of PTP Inhibitor III induced thermal hyperalgesia in rats. [] Importantly, this hyperalgesia was effectively blocked by pre-treatment with TRPV1 antagonists, confirming the involvement of TRPV1 in this pathway. []
Q2: What is the significance of studying SHP-1 and its interplay with PTP Inhibitor III in inflammatory pain models?
A: Research indicates that SHP-1 plays a crucial role in regulating TRPV1 activity and, consequently, pain sensation. [] In the provided study, CFA-induced inflammatory pain in rats led to an increase in SHP-1 expression in DRG neurons, alongside increased TRPV1 expression and phosphorylation. [] This suggests a compensatory mechanism where SHP-1 upregulation attempts to dampen the increased TRPV1 activity and alleviate excessive pain. Further supporting this, overexpressing SHP-1 in DRG neurons actually reduced CFA-induced inflammatory pain in the rat model. [] Therefore, studying the interactions between SHP-1 and PTP Inhibitor III can provide valuable insights into the complex mechanisms of inflammatory pain and potentially identify novel therapeutic targets.
Haftungsausschluss und Informationen zu In-Vitro-Forschungsprodukten
Bitte beachten Sie, dass alle Artikel und Produktinformationen, die auf BenchChem präsentiert werden, ausschließlich zu Informationszwecken bestimmt sind. Die auf BenchChem zum Kauf angebotenen Produkte sind speziell für In-vitro-Studien konzipiert, die außerhalb lebender Organismen durchgeführt werden. In-vitro-Studien, abgeleitet von dem lateinischen Begriff "in Glas", beinhalten Experimente, die in kontrollierten Laborumgebungen unter Verwendung von Zellen oder Geweben durchgeführt werden. Es ist wichtig zu beachten, dass diese Produkte nicht als Arzneimittel oder Medikamente eingestuft sind und keine Zulassung der FDA für die Vorbeugung, Behandlung oder Heilung von medizinischen Zuständen, Beschwerden oder Krankheiten erhalten haben. Wir müssen betonen, dass jede Form der körperlichen Einführung dieser Produkte in Menschen oder Tiere gesetzlich strikt untersagt ist. Es ist unerlässlich, sich an diese Richtlinien zu halten, um die Einhaltung rechtlicher und ethischer Standards in Forschung und Experiment zu gewährleisten.