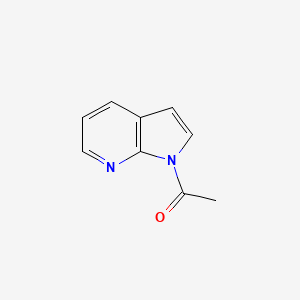
1-Acetyl-7-azaindole
Übersicht
Beschreibung
1-Acetyl-7-azaindole, also known as 1-acetyl-1H-pyrrolo[2,3-b]pyridine-3-carbonitrile, is a heterocyclic compound. Its chemical structure consists of a pyrrolopyridine ring with an acetyl group (CH₃CO-) attached at position 1. The azaindole moiety contributes to its unique properties and potential applications .
Synthesis Analysis
The synthesis of this compound involves various methods, including cyclization reactions. Researchers have explored both traditional and novel synthetic routes. These approaches often utilize readily available starting materials and aim for high yields and purity. Further studies are needed to optimize the synthesis for large-scale production .
Molecular Structure Analysis
The molecular structure of this compound plays a crucial role in its biological activity. The acetyl group enhances its lipophilicity, affecting its solubility and membrane permeability. The pyrrolopyridine scaffold contributes to its binding interactions with biological targets .
Chemical Reactions Analysis
This compound can participate in various chemical reactions, such as nucleophilic substitutions, cyclizations, and functional group transformations. Researchers have explored its reactivity with different electrophiles and nucleophiles. These reactions can lead to the synthesis of derivatives with modified properties .
Physical And Chemical Properties Analysis
Wissenschaftliche Forschungsanwendungen
1. Chemical Reactivity and Synthesis:
- 7-Azaindole reacts with benzophenone to produce an oxetane via cycloaddition, demonstrating its potential in chemical synthesis and reactivity studies (Nakano & Santana, 1976).
- Microwave-assisted synthesis methods have been developed for creating 7-azaindoles, indicating its versatility as a building block in medicinal chemistry (Schirok, 2006).
2. Biological Probes and Imaging:
- 7-Azaindole derivatives, including 1-acetyl-7-azaindole, are extensively investigated for uses in biological probes and imaging, highlighting their importance in biochemistry and molecular biology (Zhao & Wang, 2010).
3. Antioxidant and Enzyme Inhibition Properties:
- Research shows that 7-azaindole-based oxadiazoles, which include derivatives of this compound, exhibit antioxidant activity and α-glucosidase inhibition potential, suggesting their potential in therapeutic applications (İzgi et al., 2022).
4. Photodynamic Therapy:
- The 1-methyl-7-azaindole derivative shows potential for photodynamic therapy in cancer treatment due to its ability to bind DNA and generate cytotoxic effects upon UVA irradiation (Pracharova et al., 2016).
5. Drug Discovery and Development:
- Advances in the functionalization of 7-azaindoles, a category that includes this compound, have been critical in drug discovery, especially for developing pharmacophores for various therapeutic targets (Kannaboina et al., 2020).
Wirkmechanismus
The mechanism of action of 1-Acetyl-7-azaindole depends on its specific biological target. It may act as a kinase inhibitor, modulating signaling pathways involved in cellular processes. Further studies are necessary to elucidate its precise mode of action and identify specific protein kinases it interacts with .
Safety and Hazards
Eigenschaften
IUPAC Name |
1-pyrrolo[2,3-b]pyridin-1-ylethanone | |
---|---|---|
Source | PubChem | |
URL | https://pubchem.ncbi.nlm.nih.gov | |
Description | Data deposited in or computed by PubChem | |
InChI |
InChI=1S/C9H8N2O/c1-7(12)11-6-4-8-3-2-5-10-9(8)11/h2-6H,1H3 | |
Source | PubChem | |
URL | https://pubchem.ncbi.nlm.nih.gov | |
Description | Data deposited in or computed by PubChem | |
InChI Key |
AIMNNNATIMKKJX-UHFFFAOYSA-N | |
Source | PubChem | |
URL | https://pubchem.ncbi.nlm.nih.gov | |
Description | Data deposited in or computed by PubChem | |
Canonical SMILES |
CC(=O)N1C=CC2=C1N=CC=C2 | |
Source | PubChem | |
URL | https://pubchem.ncbi.nlm.nih.gov | |
Description | Data deposited in or computed by PubChem | |
Molecular Formula |
C9H8N2O | |
Source | PubChem | |
URL | https://pubchem.ncbi.nlm.nih.gov | |
Description | Data deposited in or computed by PubChem | |
DSSTOX Substance ID |
DTXSID80484344 | |
Record name | 1-acetyl-7-azaindole | |
Source | EPA DSSTox | |
URL | https://comptox.epa.gov/dashboard/DTXSID80484344 | |
Description | DSSTox provides a high quality public chemistry resource for supporting improved predictive toxicology. | |
Molecular Weight |
160.17 g/mol | |
Source | PubChem | |
URL | https://pubchem.ncbi.nlm.nih.gov | |
Description | Data deposited in or computed by PubChem | |
CAS No. |
53277-42-2 | |
Record name | 1-acetyl-7-azaindole | |
Source | EPA DSSTox | |
URL | https://comptox.epa.gov/dashboard/DTXSID80484344 | |
Description | DSSTox provides a high quality public chemistry resource for supporting improved predictive toxicology. | |
Retrosynthesis Analysis
AI-Powered Synthesis Planning: Our tool employs the Template_relevance Pistachio, Template_relevance Bkms_metabolic, Template_relevance Pistachio_ringbreaker, Template_relevance Reaxys, Template_relevance Reaxys_biocatalysis model, leveraging a vast database of chemical reactions to predict feasible synthetic routes.
One-Step Synthesis Focus: Specifically designed for one-step synthesis, it provides concise and direct routes for your target compounds, streamlining the synthesis process.
Accurate Predictions: Utilizing the extensive PISTACHIO, BKMS_METABOLIC, PISTACHIO_RINGBREAKER, REAXYS, REAXYS_BIOCATALYSIS database, our tool offers high-accuracy predictions, reflecting the latest in chemical research and data.
Strategy Settings
Precursor scoring | Relevance Heuristic |
---|---|
Min. plausibility | 0.01 |
Model | Template_relevance |
Template Set | Pistachio/Bkms_metabolic/Pistachio_ringbreaker/Reaxys/Reaxys_biocatalysis |
Top-N result to add to graph | 6 |
Feasible Synthetic Routes
Haftungsausschluss und Informationen zu In-Vitro-Forschungsprodukten
Bitte beachten Sie, dass alle Artikel und Produktinformationen, die auf BenchChem präsentiert werden, ausschließlich zu Informationszwecken bestimmt sind. Die auf BenchChem zum Kauf angebotenen Produkte sind speziell für In-vitro-Studien konzipiert, die außerhalb lebender Organismen durchgeführt werden. In-vitro-Studien, abgeleitet von dem lateinischen Begriff "in Glas", beinhalten Experimente, die in kontrollierten Laborumgebungen unter Verwendung von Zellen oder Geweben durchgeführt werden. Es ist wichtig zu beachten, dass diese Produkte nicht als Arzneimittel oder Medikamente eingestuft sind und keine Zulassung der FDA für die Vorbeugung, Behandlung oder Heilung von medizinischen Zuständen, Beschwerden oder Krankheiten erhalten haben. Wir müssen betonen, dass jede Form der körperlichen Einführung dieser Produkte in Menschen oder Tiere gesetzlich strikt untersagt ist. Es ist unerlässlich, sich an diese Richtlinien zu halten, um die Einhaltung rechtlicher und ethischer Standards in Forschung und Experiment zu gewährleisten.