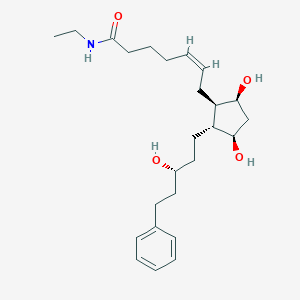
Latanoprost-Ethylamid
Übersicht
Beschreibung
Latanoprost ethyl amide is a prostanoid.
Wissenschaftliche Forschungsanwendungen
Augenspannungsreduzierende Aktivität
Latanoprost-Ethylamid ist bekannt für seine augenspannungsreduzierende Aktivität, die für die Behandlung von Erkrankungen wie Offenwinkelglaukom und Augenhochdruck entscheidend ist. Es funktioniert als alternatives Prostaglandin-Pro-Drug zur Senkung des Augeninnendrucks .
Wimpernwachstumsstimulation
Studien haben gezeigt, dass Latanoprost das Wimpernwachstum stimulieren kann, was eine Off-Label-Anwendung für this compound sein könnte. Diese Anwendung nutzt die Nebenwirkungen, die bei Glaukombehandlungen berichtet wurden .
Irispigmentierung
Latanoprost wurde mit der Verursachung von Iris- oder Lidpigmentierung als Nebenwirkung in Verbindung gebracht, die für therapeutische oder kosmetische Anwendungen in Betracht gezogen werden kann .
Pharmakokinetische Studien
Die Forschung hat gezeigt, dass Rinder- und menschliches Hornhautgewebe this compound in seine freie Säureform umwandeln kann, wenn auch mit einer langsameren Rate als andere Prostaglandin-Analoga. Diese Eigenschaft ist wichtig für das Verständnis seiner Pharmakokinetik und potenziellen therapeutischen Wirkungen .
Nanopartikel/Nanocomposite-Arzneimittelverabreichung
This compound könnte in Nanopartikel- oder Nanocomposite-Arzneimittelverabreichungssystemen verwendet werden, insbesondere in ophthalmischen Anwendungen, bei denen natürliche Reize die Freisetzung des Arzneimittels für nachhaltige therapeutische Wirkungen auslösen können .
Quantitative Analyse in Augenimplantaten und -gewebe
Es besteht das Potenzial für die Verwendung von this compound bei der Entwicklung und Validierung analytischer Methoden zur Quantifizierung von Prostaglandinspiegeln in Augenimplantaten und -gewebe .
Wirkmechanismus
Target of Action
Latanoprost ethyl amide (Lat-NEt) is a latanoprost analog, which is a prodrug analog of prostaglandin F2 alpha . The primary target of Lat-NEt is the prostaglandin F receptor . This receptor plays a crucial role in the regulation of intraocular pressure.
Mode of Action
Latanoprost ethyl amide acts as a selective agonist at the prostaglandin F receptor . By binding to this receptor, it increases the permeability of the sclera to aqueous fluid . This interaction results in an increase in the outflow of aqueous fluid from the eyes through the uveoscleral tract .
Biochemical Pathways
The biochemical pathway primarily affected by Lat-NEt is the prostaglandin synthesis pathway . Prostaglandins are a group of physiologically active lipid compounds that play key roles in a variety of biological processes. In the context of Lat-NEt, the increased outflow of aqueous fluid from the eyes is a downstream effect of its action on the prostaglandin F receptor .
Pharmacokinetics
Latanoprost ethyl amide is a prodrug, meaning it is converted into its active form in the body. Studies have shown that bovine and human corneal tissue converts the N-ethyl amides of various prostaglandins to the free acids . This conversion rate is about 2.5 μg/g corneal tissue/hr . This slow hydrolysis results in a much slower pharmacokinetics of the prostaglandin N-amides .
Result of Action
The primary result of Lat-NEt’s action is the reduction of elevated intraocular pressure . This is achieved through its effect on the prostaglandin F receptor, leading to an increase in the outflow of aqueous fluid from the eyes . This makes it an effective treatment for conditions like open-angle glaucoma or ocular hypertension .
Action Environment
The action of Lat-NEt can be influenced by various environmental factors. For instance, the conversion of Lat-NEt to its active form can be affected by the specific conditions within the corneal tissue . .
Biochemische Analyse
Biochemical Properties
Latanoprost ethyl amide plays a significant role in biochemical reactions, particularly in the context of ocular physiology. It interacts with various enzymes, proteins, and other biomolecules. One of the primary interactions is with the prostaglandin F receptor (FP receptor), which is a G protein-coupled receptor. Upon binding to the FP receptor, latanoprost ethyl amide induces a series of intracellular signaling cascades that lead to the reduction of intraocular pressure. Additionally, it is known to interact with enzymes involved in the hydrolysis of prostaglandin esters, converting them to their active free acid forms .
Cellular Effects
Latanoprost ethyl amide exerts its effects on various types of cells, particularly those in the ocular tissues. It influences cell function by modulating cell signaling pathways, gene expression, and cellular metabolism. In ocular cells, latanoprost ethyl amide activates the FP receptor, leading to increased outflow of aqueous humor through the uveoscleral pathway. This results in a reduction of intraocular pressure, which is beneficial for patients with glaucoma. Additionally, it has been observed to affect the expression of genes involved in extracellular matrix remodeling, further contributing to its therapeutic effects .
Molecular Mechanism
The molecular mechanism of action of latanoprost ethyl amide involves its binding to the FP receptor on the surface of ocular cells. This binding triggers a cascade of intracellular events, including the activation of phospholipase C (PLC) and the subsequent release of inositol trisphosphate (IP3) and diacylglycerol (DAG). These second messengers lead to the release of calcium ions from intracellular stores and the activation of protein kinase C (PKC). The overall effect is the relaxation of the ciliary muscle and increased uveoscleral outflow of aqueous humor, thereby reducing intraocular pressure .
Temporal Effects in Laboratory Settings
In laboratory settings, the effects of latanoprost ethyl amide have been studied over various time frames. The compound is relatively stable, with a degradation rate that allows for sustained activity over extended periods. In vitro studies have shown that latanoprost ethyl amide maintains its efficacy in reducing intraocular pressure for several hours after administration. Long-term studies in vivo have demonstrated that the compound continues to exert its ocular hypotensive effects without significant loss of potency over time .
Dosage Effects in Animal Models
The effects of latanoprost ethyl amide vary with different dosages in animal models. At lower doses, the compound effectively reduces intraocular pressure without causing significant adverse effects. At higher doses, some toxic effects have been observed, including ocular irritation and inflammation. It is essential to determine the optimal dosage to maximize therapeutic benefits while minimizing potential side effects .
Metabolic Pathways
Latanoprost ethyl amide is involved in several metabolic pathways, primarily related to its conversion to the active free acid form. Enzymes such as esterases play a crucial role in this conversion process. The compound’s interaction with these enzymes ensures its activation and subsequent therapeutic effects. Additionally, latanoprost ethyl amide may influence metabolic flux and metabolite levels within ocular tissues, contributing to its overall efficacy .
Transport and Distribution
Within cells and tissues, latanoprost ethyl amide is transported and distributed through various mechanisms. It interacts with specific transporters and binding proteins that facilitate its movement across cellular membranes. The compound’s localization and accumulation in ocular tissues are critical for its therapeutic action. Studies have shown that latanoprost ethyl amide is efficiently taken up by ocular cells, ensuring its sustained activity .
Subcellular Localization
Latanoprost ethyl amide exhibits specific subcellular localization, which is essential for its activity and function. It is primarily localized in the cytoplasm and associated with intracellular organelles such as the endoplasmic reticulum and Golgi apparatus. Targeting signals and post-translational modifications play a role in directing latanoprost ethyl amide to these compartments, where it can exert its effects on cellular processes .
Eigenschaften
IUPAC Name |
(Z)-7-[(1R,2R,3R,5S)-3,5-dihydroxy-2-[(3R)-3-hydroxy-5-phenylpentyl]cyclopentyl]-N-ethylhept-5-enamide | |
---|---|---|
Source | PubChem | |
URL | https://pubchem.ncbi.nlm.nih.gov | |
Description | Data deposited in or computed by PubChem | |
InChI |
InChI=1S/C25H39NO4/c1-2-26-25(30)13-9-4-3-8-12-21-22(24(29)18-23(21)28)17-16-20(27)15-14-19-10-6-5-7-11-19/h3,5-8,10-11,20-24,27-29H,2,4,9,12-18H2,1H3,(H,26,30)/b8-3-/t20-,21+,22+,23-,24+/m0/s1 | |
Source | PubChem | |
URL | https://pubchem.ncbi.nlm.nih.gov | |
Description | Data deposited in or computed by PubChem | |
InChI Key |
KNXVTDOCIZLXFW-SQACBOECSA-N | |
Source | PubChem | |
URL | https://pubchem.ncbi.nlm.nih.gov | |
Description | Data deposited in or computed by PubChem | |
Canonical SMILES |
CCNC(=O)CCCC=CCC1C(CC(C1CCC(CCC2=CC=CC=C2)O)O)O | |
Source | PubChem | |
URL | https://pubchem.ncbi.nlm.nih.gov | |
Description | Data deposited in or computed by PubChem | |
Isomeric SMILES |
CCNC(=O)CCC/C=C\C[C@H]1[C@H](C[C@H]([C@@H]1CC[C@H](CCC2=CC=CC=C2)O)O)O | |
Source | PubChem | |
URL | https://pubchem.ncbi.nlm.nih.gov | |
Description | Data deposited in or computed by PubChem | |
Molecular Formula |
C25H39NO4 | |
Source | PubChem | |
URL | https://pubchem.ncbi.nlm.nih.gov | |
Description | Data deposited in or computed by PubChem | |
DSSTOX Substance ID |
DTXSID601111765 | |
Record name | (5Z)-7-[(1R,2R,3R,5S)-3,5-Dihydroxy-2-[(3R)-3-hydroxy-5-phenylpentyl]cyclopentyl]-N-ethyl-5-heptenamide | |
Source | EPA DSSTox | |
URL | https://comptox.epa.gov/dashboard/DTXSID601111765 | |
Description | DSSTox provides a high quality public chemistry resource for supporting improved predictive toxicology. | |
Molecular Weight |
417.6 g/mol | |
Source | PubChem | |
URL | https://pubchem.ncbi.nlm.nih.gov | |
Description | Data deposited in or computed by PubChem | |
CAS No. |
607351-44-0 | |
Record name | (5Z)-7-[(1R,2R,3R,5S)-3,5-Dihydroxy-2-[(3R)-3-hydroxy-5-phenylpentyl]cyclopentyl]-N-ethyl-5-heptenamide | |
Source | CAS Common Chemistry | |
URL | https://commonchemistry.cas.org/detail?cas_rn=607351-44-0 | |
Description | CAS Common Chemistry is an open community resource for accessing chemical information. Nearly 500,000 chemical substances from CAS REGISTRY cover areas of community interest, including common and frequently regulated chemicals, and those relevant to high school and undergraduate chemistry classes. This chemical information, curated by our expert scientists, is provided in alignment with our mission as a division of the American Chemical Society. | |
Explanation | The data from CAS Common Chemistry is provided under a CC-BY-NC 4.0 license, unless otherwise stated. | |
Record name | (5Z)-7-[(1R,2R,3R,5S)-3,5-Dihydroxy-2-[(3R)-3-hydroxy-5-phenylpentyl]cyclopentyl]-N-ethyl-5-heptenamide | |
Source | EPA DSSTox | |
URL | https://comptox.epa.gov/dashboard/DTXSID601111765 | |
Description | DSSTox provides a high quality public chemistry resource for supporting improved predictive toxicology. | |
Retrosynthesis Analysis
AI-Powered Synthesis Planning: Our tool employs the Template_relevance Pistachio, Template_relevance Bkms_metabolic, Template_relevance Pistachio_ringbreaker, Template_relevance Reaxys, Template_relevance Reaxys_biocatalysis model, leveraging a vast database of chemical reactions to predict feasible synthetic routes.
One-Step Synthesis Focus: Specifically designed for one-step synthesis, it provides concise and direct routes for your target compounds, streamlining the synthesis process.
Accurate Predictions: Utilizing the extensive PISTACHIO, BKMS_METABOLIC, PISTACHIO_RINGBREAKER, REAXYS, REAXYS_BIOCATALYSIS database, our tool offers high-accuracy predictions, reflecting the latest in chemical research and data.
Strategy Settings
Precursor scoring | Relevance Heuristic |
---|---|
Min. plausibility | 0.01 |
Model | Template_relevance |
Template Set | Pistachio/Bkms_metabolic/Pistachio_ringbreaker/Reaxys/Reaxys_biocatalysis |
Top-N result to add to graph | 6 |
Feasible Synthetic Routes
Haftungsausschluss und Informationen zu In-Vitro-Forschungsprodukten
Bitte beachten Sie, dass alle Artikel und Produktinformationen, die auf BenchChem präsentiert werden, ausschließlich zu Informationszwecken bestimmt sind. Die auf BenchChem zum Kauf angebotenen Produkte sind speziell für In-vitro-Studien konzipiert, die außerhalb lebender Organismen durchgeführt werden. In-vitro-Studien, abgeleitet von dem lateinischen Begriff "in Glas", beinhalten Experimente, die in kontrollierten Laborumgebungen unter Verwendung von Zellen oder Geweben durchgeführt werden. Es ist wichtig zu beachten, dass diese Produkte nicht als Arzneimittel oder Medikamente eingestuft sind und keine Zulassung der FDA für die Vorbeugung, Behandlung oder Heilung von medizinischen Zuständen, Beschwerden oder Krankheiten erhalten haben. Wir müssen betonen, dass jede Form der körperlichen Einführung dieser Produkte in Menschen oder Tiere gesetzlich strikt untersagt ist. Es ist unerlässlich, sich an diese Richtlinien zu halten, um die Einhaltung rechtlicher und ethischer Standards in Forschung und Experiment zu gewährleisten.