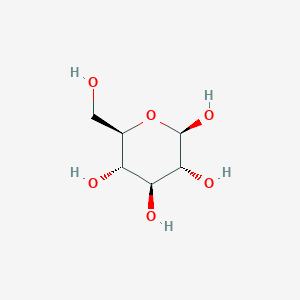
beta-D-Glucose
Übersicht
Beschreibung
Beta-D-Glucose is a form of glucose, a simple sugar that serves as a primary source of energy for living organisms . It is naturally occurring and is found in fruits and other parts of plants in its free state . This compound is a D-Glucopyranose with beta configuration at the anomeric centre . It has a role as an epitope and a mouse metabolite .
Synthesis Analysis
The synthesis of this compound is connected to the way of extraction from the natural source . Various studies have reported the relationship between the molecular structure of this compound and its functionality . The structural characteristics of this compound, including specific glycosidic linkages, monosaccharide compositions, molecular weight, and chain conformation, were reported to affect its physiochemical and biological properties .Molecular Structure Analysis
This compound contains total 24 bond(s); 12 non-H bond(s), 1 rotatable bond(s), 1 six-membered ring(s), 5 hydroxyl group(s), 1 primary alcohol(s), 3 secondary alcohol(s), and 1 ether(s) (aliphatic) . The two-dimensional graphical representation of these isomeric glucose structures shows that the this compound has (1-hydroxyl) and (4-hydroxyl) orientations on the same side .Chemical Reactions Analysis
This compound has been reported to have various chemical reactions. For instance, the products released from the digestion of purified this compound are mainly cellobiosyl- (1→3)-beta-D-Glucose and cellotriosyl- (1→3)-beta-D-Glucose, with smaller amounts of cellotetraosyl- (1→3)-beta-D-Glucose and cellopentaosyl- (1→3)-beta-D-Glucose .Physical and Chemical Properties Analysis
The high molecular weight (Mw) and high viscosity of this compound are responsible for its hypocholesterolemic and hypoglycemic properties . The inherent gel-forming property and high viscosity of this compound lead to the production of low-fat foods with improved textural properties .Wissenschaftliche Forschungsanwendungen
Biokraftstoffproduktion
Beta-D-Glucose ist entscheidend für die Saccharifizierung von lignocellulosehaltiger Biomasse, einem wichtigen Schritt bei der Herstellung von Bioethanol, einem erneuerbaren Energieträger. Enzyme wie Beta-Glucosidasen (BGLs) zersetzen Cellulose in Glucose, die dann zu Ethanol fermentiert werden kann .
Lebensmittel- und Getränkeindustrie
In der Lebensmittelindustrie verbessern BGLs die Aromen bei der Wein- und Saftproduktion, indem sie Glucoside in aromatische Verbindungen aufspalten. Sie werden auch verwendet, um die Verdauung von Cellulose in Tierfutter zu verbessern und so zu einer besseren Nährstoffaufnahme beizutragen .
Pharmazeutische Anwendungen
This compound-Derivate werden in Pharmazeutika aufgrund ihrer enzymatischen Aktivität eingesetzt, die bei Medikamentenabgabesystemen und der Synthese komplexer Moleküle helfen kann .
Entgiftungsprozesse
BGLs spielen eine Rolle bei der Entgiftung cyanogener Glucoside in Nahrungspflanzen, wodurch sie für den Verzehr sicherer werden und das Risiko einer Cyanidvergiftung verringert wird .
Altpapierrecycling
Die enzymatische Aktivität von BGLs wird beim Altpapierrecycling genutzt, um Cellulosefasern abzubauen und so den Prozess der Papieraufbereitung zu unterstützen und zu nachhaltigen Abfallmanagementpraktiken beizutragen .
Textilindustrie
In der Textilindustrie werden Cellulasen, darunter Beta-Glucosidasen, verwendet, um die Oberflächeneigenschaften von Stoffen zu modifizieren, die Weichheit zu verbessern und gewünschte Effekte wie Stone-Washed-Looks zu erzielen, ohne aggressive Chemikalien verwenden zu müssen .
Waschmittelindustrie
This compound-Enzyme werden Waschmitteln zugesetzt, um Flecken und Schmutz auf Stoffen abzubauen, die Reinigungseffizienz zu verbessern und die Umweltbelastung zu reduzieren, indem das Waschen bei niedrigeren Temperaturen ermöglicht wird .
Biochemische Forschung
This compound wird als Substrat in biochemischen Assays verwendet, um die Enzymkinetik und Stoffwechselwege zu untersuchen und so Einblicke in zelluläre Prozesse und Krankheitsmechanismen zu gewinnen .
Wirkmechanismus
Target of Action
Beta-D-Glucose, a naturally occurring compound found in fruits and other parts of plants , interacts with various targets in living organisms. It is known to interact with several enzymes such as DNA, Chondroitinase-B, Endoglucanase 5A, Lactase-like protein, Glycogen phosphorylase, Xylose isomerase, Glucokinase, Ferrichrome-iron receptor, and Hexokinase-1 . These enzymes play crucial roles in various biochemical processes, including energy production, metabolism, and cellular function .
Mode of Action
This compound’s mode of action is primarily through its interaction with these enzymes. For instance, Glucokinase, an enzyme found in the liver and beta cells of the pancreas, catalyzes the initial step in the utilization of glucose at physiological glucose concentration . This compound is also known to interact with Hexokinase-1, another key enzyme involved in the glycolytic pathway . The interaction of this compound with these enzymes leads to various biochemical changes within the cell .
Biochemical Pathways
This compound plays a significant role in the glycolytic pathway, also known as the Meyerhoff, Embden, Parnas pathway . This pathway involves the anaerobic conversion of glucose to pyruvate, providing the cell with ATP under anaerobic conditions and supplying precursors for the biosynthesis of proteins, lipids, nucleic acids, and polysaccharides . The enzymes involved in glycolysis, including Hexokinase and Phosphofructokinase 1 (PFK 1), are regulated by small molecules, and this compound plays a crucial role in this regulation .
Pharmacokinetics
While specific pharmacokinetic data on this compound is limited, it is known that the compound exhibits dose-proportional pharmacokinetics without apparent accumulation in the body . This suggests that this compound is metabolized and excreted efficiently, impacting its bioavailability.
Result of Action
The primary result of this compound’s action is the provision of energy for living organisms . By participating in glycolysis, this compound contributes to the production of ATP, the primary energy currency of the cell . Additionally, the interaction of this compound with various enzymes can lead to changes in cellular function and metabolism .
Action Environment
The action of this compound is influenced by various environmental factors. For instance, the activity of this compound-degrading enzymes can be affected by factors such as temperature and pH . Additionally, the presence of other molecules can impact the action of this compound. For example, in the presence of certain microbial structures, plant Beta-glucanases can hydrolyze Beta-glucans, triggering the activation of global responses .
Biochemische Analyse
Biochemical Properties
Beta-D-Glucose plays a crucial role in various biochemical reactions. It is the primary source of energy for cells and is metabolized through glycolysis. This compound interacts with various enzymes, such as hexokinase and glucokinase, which phosphorylate glucose to glucose-6-phosphate, the first step in most glucose metabolism pathways .
Cellular Effects
This compound impacts various types of cells and cellular processes. It influences cell function by providing the energy needed for cellular processes. It also impacts cell signaling pathways, gene expression, and cellular metabolism. For instance, high levels of this compound can lead to increased insulin production in beta cells of the pancreas .
Molecular Mechanism
The molecular mechanism of this compound involves its conversion to glucose-6-phosphate by the action of hexokinase or glucokinase. This is a crucial step as it keeps glucose inside the cell and makes it available for various metabolic pathways .
Temporal Effects in Laboratory Settings
In laboratory settings, this compound shows stability over time. Its effects on cellular function can vary depending on the concentration and duration of exposure .
Dosage Effects in Animal Models
In animal models, the effects of this compound can vary with different dosages. High doses of glucose can lead to hyperglycemia and insulin resistance, while low doses may not provide sufficient energy for normal cellular function .
Metabolic Pathways
This compound is involved in several metabolic pathways, including glycolysis, gluconeogenesis, and the pentose phosphate pathway. It interacts with various enzymes in these pathways, such as hexokinase, phosphofructokinase, and glucose-6-phosphate dehydrogenase .
Transport and Distribution
This compound is transported into cells via glucose transporters (GLUTs). Once inside the cell, it is phosphorylated to glucose-6-phosphate to prevent it from leaving the cell .
Subcellular Localization
Inside the cell, this compound is primarily found in the cytoplasm where glycolysis takes place. It can also be found in the mitochondria where it can be further metabolized to produce ATP .
Eigenschaften
IUPAC Name |
6-(hydroxymethyl)oxane-2,3,4,5-tetrol | |
---|---|---|
Details | Computed by Lexichem TK 2.7.0 (PubChem release 2021.05.07) | |
Source | PubChem | |
URL | https://pubchem.ncbi.nlm.nih.gov | |
Description | Data deposited in or computed by PubChem | |
InChI |
InChI=1S/C6H12O6/c7-1-2-3(8)4(9)5(10)6(11)12-2/h2-11H,1H2 | |
Details | Computed by InChI 1.0.6 (PubChem release 2021.05.07) | |
Source | PubChem | |
URL | https://pubchem.ncbi.nlm.nih.gov | |
Description | Data deposited in or computed by PubChem | |
InChI Key |
WQZGKKKJIJFFOK-UHFFFAOYSA-N | |
Details | Computed by InChI 1.0.6 (PubChem release 2021.05.07) | |
Source | PubChem | |
URL | https://pubchem.ncbi.nlm.nih.gov | |
Description | Data deposited in or computed by PubChem | |
Canonical SMILES |
C(C1C(C(C(C(O1)O)O)O)O)O | |
Details | Computed by OEChem 2.3.0 (PubChem release 2021.05.07) | |
Source | PubChem | |
URL | https://pubchem.ncbi.nlm.nih.gov | |
Description | Data deposited in or computed by PubChem | |
Molecular Formula |
C6H12O6 | |
Details | Computed by PubChem 2.1 (PubChem release 2021.05.07) | |
Source | PubChem | |
URL | https://pubchem.ncbi.nlm.nih.gov | |
Description | Data deposited in or computed by PubChem | |
DSSTOX Substance ID |
DTXSID80858960 | |
Record name | Hexopyranose | |
Source | EPA DSSTox | |
URL | https://comptox.epa.gov/dashboard/DTXSID80858960 | |
Description | DSSTox provides a high quality public chemistry resource for supporting improved predictive toxicology. | |
Molecular Weight |
180.16 g/mol | |
Details | Computed by PubChem 2.1 (PubChem release 2021.05.07) | |
Source | PubChem | |
URL | https://pubchem.ncbi.nlm.nih.gov | |
Description | Data deposited in or computed by PubChem | |
CAS No. |
42752-07-8, 579-36-2, 2280-44-6, 39392-65-9, 10257-28-0 | |
Record name | Hexopyranose | |
Source | CAS Common Chemistry | |
URL | https://commonchemistry.cas.org/detail?cas_rn=42752-07-8 | |
Description | CAS Common Chemistry is an open community resource for accessing chemical information. Nearly 500,000 chemical substances from CAS REGISTRY cover areas of community interest, including common and frequently regulated chemicals, and those relevant to high school and undergraduate chemistry classes. This chemical information, curated by our expert scientists, is provided in alignment with our mission as a division of the American Chemical Society. | |
Explanation | The data from CAS Common Chemistry is provided under a CC-BY-NC 4.0 license, unless otherwise stated. | |
Record name | D-Allopyranose | |
Source | DTP/NCI | |
URL | https://dtp.cancer.gov/dtpstandard/servlet/dwindex?searchtype=NSC&outputformat=html&searchlist=144657 | |
Description | The NCI Development Therapeutics Program (DTP) provides services and resources to the academic and private-sector research communities worldwide to facilitate the discovery and development of new cancer therapeutic agents. | |
Explanation | Unless otherwise indicated, all text within NCI products is free of copyright and may be reused without our permission. Credit the National Cancer Institute as the source. | |
Record name | NSC287045 | |
Source | DTP/NCI | |
URL | https://dtp.cancer.gov/dtpstandard/servlet/dwindex?searchtype=NSC&outputformat=html&searchlist=287045 | |
Description | The NCI Development Therapeutics Program (DTP) provides services and resources to the academic and private-sector research communities worldwide to facilitate the discovery and development of new cancer therapeutic agents. | |
Explanation | Unless otherwise indicated, all text within NCI products is free of copyright and may be reused without our permission. Credit the National Cancer Institute as the source. | |
Record name | NSC274237 | |
Source | DTP/NCI | |
URL | https://dtp.cancer.gov/dtpstandard/servlet/dwindex?searchtype=NSC&outputformat=html&searchlist=274237 | |
Description | The NCI Development Therapeutics Program (DTP) provides services and resources to the academic and private-sector research communities worldwide to facilitate the discovery and development of new cancer therapeutic agents. | |
Explanation | Unless otherwise indicated, all text within NCI products is free of copyright and may be reused without our permission. Credit the National Cancer Institute as the source. | |
Record name | D-galactose | |
Source | DTP/NCI | |
URL | https://dtp.cancer.gov/dtpstandard/servlet/dwindex?searchtype=NSC&outputformat=html&searchlist=8102 | |
Description | The NCI Development Therapeutics Program (DTP) provides services and resources to the academic and private-sector research communities worldwide to facilitate the discovery and development of new cancer therapeutic agents. | |
Explanation | Unless otherwise indicated, all text within NCI products is free of copyright and may be reused without our permission. Credit the National Cancer Institute as the source. | |
Record name | Hexopyranose | |
Source | EPA DSSTox | |
URL | https://comptox.epa.gov/dashboard/DTXSID80858960 | |
Description | DSSTox provides a high quality public chemistry resource for supporting improved predictive toxicology. | |
Synthesis routes and methods I
Procedure details
Synthesis routes and methods II
Procedure details
Retrosynthesis Analysis
AI-Powered Synthesis Planning: Our tool employs the Template_relevance Pistachio, Template_relevance Bkms_metabolic, Template_relevance Pistachio_ringbreaker, Template_relevance Reaxys, Template_relevance Reaxys_biocatalysis model, leveraging a vast database of chemical reactions to predict feasible synthetic routes.
One-Step Synthesis Focus: Specifically designed for one-step synthesis, it provides concise and direct routes for your target compounds, streamlining the synthesis process.
Accurate Predictions: Utilizing the extensive PISTACHIO, BKMS_METABOLIC, PISTACHIO_RINGBREAKER, REAXYS, REAXYS_BIOCATALYSIS database, our tool offers high-accuracy predictions, reflecting the latest in chemical research and data.
Strategy Settings
Precursor scoring | Relevance Heuristic |
---|---|
Min. plausibility | 0.01 |
Model | Template_relevance |
Template Set | Pistachio/Bkms_metabolic/Pistachio_ringbreaker/Reaxys/Reaxys_biocatalysis |
Top-N result to add to graph | 6 |
Feasible Synthetic Routes
Q1: What is the molecular formula and weight of β-D-glucose?
A1: β-D-Glucose shares the same molecular formula as its isomer α-D-glucose: C6H12O6. Its molecular weight is 180.16 g/mol.
Q2: How does the structure of β-D-glucose differ from α-D-glucose?
A2: Both are anomers, differing in the orientation of the hydroxyl group (-OH) at the anomeric carbon (C1). In β-D-glucose, the -OH group on C1 is oriented in the equatorial position, while in α-D-glucose, it is in the axial position. This seemingly small difference leads to distinct chemical properties and biological activities.
Q3: Are there spectroscopic techniques to differentiate between the anomers of D-glucose?
A3: Yes, techniques like Nuclear Magnetic Resonance (NMR) spectroscopy can distinguish between α and β anomers. For instance, the anomeric proton (H1) in β-D-glucose typically exhibits a characteristic chemical shift in the 1H NMR spectrum. []
Q4: How does β-D-glucose interact with enzymes involved in glucose metabolism?
A4: β-D-glucose exhibits distinct interactions with various enzymes involved in glucose metabolism. For instance, studies on hexokinase, an enzyme catalyzing the first step of glycolysis, demonstrate anomeric preference. Bovine heart hexokinase displays a higher maximal velocity with β-D-glucose, while yeast hexokinase shows a higher affinity for α-D-glucose. Interestingly, these anomeric preferences are temperature-dependent. [] []
Q5: Do these anomeric preferences translate to differences in metabolic fate?
A5: Yes, studies in rat adipocytes revealed that while α-D-glucose utilization was higher overall, the fraction of glucose catabolized via the pentose phosphate pathway was higher with β-D-glucose. These findings highlight the anomeric specificity of glucose metabolism even when exposed to equilibrated D-glucose. []
Q6: Are there differences in how β-D-glucose is transported compared to α-D-glucose?
A6: Research on human red blood cells suggests that both anomers are transported by the hexose transport system without any significant difference. This was observed at both low (0.6 degrees C) and physiological (36.6 degrees C) temperatures, suggesting no anomeric preference for the transporter. []
Q7: Does β-D-glucose play a role in the synthesis of specialized metabolites?
A7: Yes, β-D-glucose serves as a precursor for the synthesis of various compounds. In Lycopersicon pennellii, a wild tomato species, β-D-glucose is involved in the biosynthesis of 2,3,4-tri-O-acylglucoses. These compounds, secreted by glandular trichomes, possess short to medium-chain fatty acids and contribute to plant defense mechanisms. The biosynthesis involves the activation of fatty acids to form 1-O-acyl-β-D-glucopyranose derivatives, which then undergo transacylation reactions to generate the final tri-O-acylglucoses. [] []
Q8: Can β-D-glucose act as a stabilizing agent in nanoparticle synthesis?
A8: Absolutely. Research demonstrates the use of β-D-glucose as both a reducing and capping agent in the "green" synthesis of gold nanoparticles. These nanoparticles, averaging 8.2 nm in diameter, were successfully extracted into an organic phase and used to create thin films and ordered arrays. [] []
Q9: Has β-D-glucose been utilized in the development of catalytic systems?
A9: Yes, β-D-glucose-stabilized gold nanoparticles have demonstrated catalytic activity. They effectively catalyze the reduction of 4-nitrophenol in the presence of NaBH4, a reaction that is otherwise unfeasible with NaBH4 alone. This highlights the potential of these bio-synthesized nanoparticles for catalytic applications. []
Q10: What is the significance of the β-D-glucose scaffold in drug discovery?
A10: The β-D-glucose scaffold holds promise as a β-turn mimetic in drug design. Research has shown that this scaffold can mimic the β-turn in somatostatin (SRIF) and its synthetic analog L-363,301, both of which bind to SRIF receptors. This discovery paved the way for the development of non-peptidic β-D-glucose-based analogs with agonist activity at SRIF receptors. []
Haftungsausschluss und Informationen zu In-Vitro-Forschungsprodukten
Bitte beachten Sie, dass alle Artikel und Produktinformationen, die auf BenchChem präsentiert werden, ausschließlich zu Informationszwecken bestimmt sind. Die auf BenchChem zum Kauf angebotenen Produkte sind speziell für In-vitro-Studien konzipiert, die außerhalb lebender Organismen durchgeführt werden. In-vitro-Studien, abgeleitet von dem lateinischen Begriff "in Glas", beinhalten Experimente, die in kontrollierten Laborumgebungen unter Verwendung von Zellen oder Geweben durchgeführt werden. Es ist wichtig zu beachten, dass diese Produkte nicht als Arzneimittel oder Medikamente eingestuft sind und keine Zulassung der FDA für die Vorbeugung, Behandlung oder Heilung von medizinischen Zuständen, Beschwerden oder Krankheiten erhalten haben. Wir müssen betonen, dass jede Form der körperlichen Einführung dieser Produkte in Menschen oder Tiere gesetzlich strikt untersagt ist. Es ist unerlässlich, sich an diese Richtlinien zu halten, um die Einhaltung rechtlicher und ethischer Standards in Forschung und Experiment zu gewährleisten.