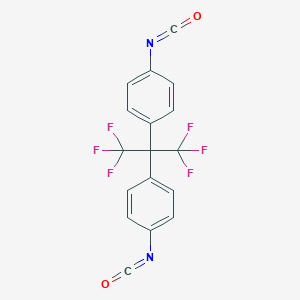
2,2-Bis(4-isocyanatophenyl)hexafluoropropane
Übersicht
Beschreibung
2,2-Bis(4-isocyanatophenyl)hexafluoropropane is a chemical compound with the molecular formula C17H8F6N2O2 . It is primarily used for research purposes .
Molecular Structure Analysis
The molecular structure of this compound contains a total of 36 bonds. These include 28 non-H bonds, 16 multiple bonds, 4 rotatable bonds, 4 double bonds, 12 aromatic bonds, 2 six-membered rings, and 2 isocyanates (aromatic) .Physical and Chemical Properties Analysis
This compound is a liquid at 20°C. It has a molecular weight of 386.25. The compound has a flash point of 189°C, a specific gravity of 1.46 (20/20), and a refractive index of 1.53 .Wissenschaftliche Forschungsanwendungen
Application in Elastomers
2,2-Bis(4-isocyanatophenyl)hexafluoropropane has been utilized as a component in the synthesis of novel compounds applicable as curing agents for fluorine-containing elastomers, particularly those with CN groups as cross-linkable groups (Sonoi, Tatsu, German, & Polishchuk, 1998).
Reactivity Studies
Research on the kinetics of reactions involving this compound, specifically its interaction with n-butanol, has provided insights into its reactivity compared to similar compounds (Gafurova, Chernyi, Lodygina, Livshits, Baturin, & Entelis, 1976).
Sensor Development
The compound's derivatives, like 2,2-bis(3-allyl-4-hydroxyphenyl)hexafluoropropane, have been developed for use as coating materials in the detection of toxic chemical warfare agents, demonstrating its utility in sensitive and critical applications (Bhadury, Dubey, Singh, & Saxena, 2005).
Polymer Synthesis
Its use extends to the field of polymer science, where it plays a critical role in the synthesis of various polymers such as fluorinated metal-organic frameworks with selective gas sorption properties (Fernandez et al., 2010), and in the development of fluorine-containing polyamides with notable thermal stability (Liaw & Wang, 1996).
Advanced Materials
In advanced materials, it is used for synthesizing new polymers with specific properties, such as iron-based metal-organic frameworks capable of selective separation of hexane isomers, a crucial process in refining gasoline (Lv et al., 2018).
Applications in High-Performance Polymers
High-performance fluorinated polyimides have been developed from derivatives of this compound for use in areas requiring materials with exceptional thermal and dielectric properties (Guan et al., 2014).
Safety and Hazards
Wirkmechanismus
Target of Action
This compound is primarily used in the synthesis of polyimides
Mode of Action
As a diisocyanate, 2,2-Bis(4-isocyanatophenyl)hexafluoropropane can react with compounds containing active hydrogen atoms, such as alcohols and amines. The isocyanate groups (-N=C=O) can form urethane or urea linkages, which are key components in polyurethane and polyurea polymers .
Result of Action
The primary result of the action of this compound is the formation of polyimide structures
Action Environment
The action of this compound can be influenced by environmental factors such as temperature and humidity. For instance, it is sensitive to moisture and heat . In a biological context, factors such as pH and the presence of reactive biological molecules could potentially influence its reactivity and stability.
Eigenschaften
IUPAC Name |
1-[1,1,1,3,3,3-hexafluoro-2-(4-isocyanatophenyl)propan-2-yl]-4-isocyanatobenzene | |
---|---|---|
Source | PubChem | |
URL | https://pubchem.ncbi.nlm.nih.gov | |
Description | Data deposited in or computed by PubChem | |
InChI |
InChI=1S/C17H8F6N2O2/c18-16(19,20)15(17(21,22)23,11-1-5-13(6-2-11)24-9-26)12-3-7-14(8-4-12)25-10-27/h1-8H | |
Source | PubChem | |
URL | https://pubchem.ncbi.nlm.nih.gov | |
Description | Data deposited in or computed by PubChem | |
InChI Key |
QIPLQPPNURSGKC-UHFFFAOYSA-N | |
Source | PubChem | |
URL | https://pubchem.ncbi.nlm.nih.gov | |
Description | Data deposited in or computed by PubChem | |
Canonical SMILES |
C1=CC(=CC=C1C(C2=CC=C(C=C2)N=C=O)(C(F)(F)F)C(F)(F)F)N=C=O | |
Source | PubChem | |
URL | https://pubchem.ncbi.nlm.nih.gov | |
Description | Data deposited in or computed by PubChem | |
Molecular Formula |
C17H8F6N2O2 | |
Source | PubChem | |
URL | https://pubchem.ncbi.nlm.nih.gov | |
Description | Data deposited in or computed by PubChem | |
DSSTOX Substance ID |
DTXSID10371096 | |
Record name | 2,2-Bis(4-isocyanatophenyl)hexafluoropropane | |
Source | EPA DSSTox | |
URL | https://comptox.epa.gov/dashboard/DTXSID10371096 | |
Description | DSSTox provides a high quality public chemistry resource for supporting improved predictive toxicology. | |
Molecular Weight |
386.25 g/mol | |
Source | PubChem | |
URL | https://pubchem.ncbi.nlm.nih.gov | |
Description | Data deposited in or computed by PubChem | |
CAS No. |
10224-18-7 | |
Record name | 2,2-Bis(4-isocyanatophenyl)hexafluoropropane | |
Source | EPA DSSTox | |
URL | https://comptox.epa.gov/dashboard/DTXSID10371096 | |
Description | DSSTox provides a high quality public chemistry resource for supporting improved predictive toxicology. | |
Record name | 2,2-Bis(4-isocyanatophenyl)hexafluoropropane | |
Source | European Chemicals Agency (ECHA) | |
URL | https://echa.europa.eu/information-on-chemicals | |
Description | The European Chemicals Agency (ECHA) is an agency of the European Union which is the driving force among regulatory authorities in implementing the EU's groundbreaking chemicals legislation for the benefit of human health and the environment as well as for innovation and competitiveness. | |
Explanation | Use of the information, documents and data from the ECHA website is subject to the terms and conditions of this Legal Notice, and subject to other binding limitations provided for under applicable law, the information, documents and data made available on the ECHA website may be reproduced, distributed and/or used, totally or in part, for non-commercial purposes provided that ECHA is acknowledged as the source: "Source: European Chemicals Agency, http://echa.europa.eu/". Such acknowledgement must be included in each copy of the material. ECHA permits and encourages organisations and individuals to create links to the ECHA website under the following cumulative conditions: Links can only be made to webpages that provide a link to the Legal Notice page. | |
Retrosynthesis Analysis
AI-Powered Synthesis Planning: Our tool employs the Template_relevance Pistachio, Template_relevance Bkms_metabolic, Template_relevance Pistachio_ringbreaker, Template_relevance Reaxys, Template_relevance Reaxys_biocatalysis model, leveraging a vast database of chemical reactions to predict feasible synthetic routes.
One-Step Synthesis Focus: Specifically designed for one-step synthesis, it provides concise and direct routes for your target compounds, streamlining the synthesis process.
Accurate Predictions: Utilizing the extensive PISTACHIO, BKMS_METABOLIC, PISTACHIO_RINGBREAKER, REAXYS, REAXYS_BIOCATALYSIS database, our tool offers high-accuracy predictions, reflecting the latest in chemical research and data.
Strategy Settings
Precursor scoring | Relevance Heuristic |
---|---|
Min. plausibility | 0.01 |
Model | Template_relevance |
Template Set | Pistachio/Bkms_metabolic/Pistachio_ringbreaker/Reaxys/Reaxys_biocatalysis |
Top-N result to add to graph | 6 |
Feasible Synthetic Routes
A: 2,2-Bis(4-isocyanatophenyl)hexafluoropropane is a diisocyanate commonly used in polyurethane synthesis. [, ] It reacts with polyols (molecules with multiple hydroxyl groups) through its two isocyanate (-N=C=O) groups. This reaction forms urethane linkages (-NH-COO-), ultimately creating crosslinked polyurethane networks. [] The properties of the resulting polyurethane, such as its flexibility, strength, and thermal stability, are influenced by factors like the specific polyol used and the reaction conditions.
A:
A: The research on the "kinetics of three dimensional polymerization and the properties of crosslinked polyether urethane elastomers synthesized with the participation of 2,2-bis-(4-isocyanatophenyl) hexafluoropropane" [] directly investigates this question. While the abstract doesn't provide specific results, it's likely the study examines how this specific diisocyanate influences the properties of the final polyurethane elastomers. Factors like mechanical strength, thermal degradation behavior, and resistance to solvents would be relevant to material compatibility and stability.
Haftungsausschluss und Informationen zu In-Vitro-Forschungsprodukten
Bitte beachten Sie, dass alle Artikel und Produktinformationen, die auf BenchChem präsentiert werden, ausschließlich zu Informationszwecken bestimmt sind. Die auf BenchChem zum Kauf angebotenen Produkte sind speziell für In-vitro-Studien konzipiert, die außerhalb lebender Organismen durchgeführt werden. In-vitro-Studien, abgeleitet von dem lateinischen Begriff "in Glas", beinhalten Experimente, die in kontrollierten Laborumgebungen unter Verwendung von Zellen oder Geweben durchgeführt werden. Es ist wichtig zu beachten, dass diese Produkte nicht als Arzneimittel oder Medikamente eingestuft sind und keine Zulassung der FDA für die Vorbeugung, Behandlung oder Heilung von medizinischen Zuständen, Beschwerden oder Krankheiten erhalten haben. Wir müssen betonen, dass jede Form der körperlichen Einführung dieser Produkte in Menschen oder Tiere gesetzlich strikt untersagt ist. Es ist unerlässlich, sich an diese Richtlinien zu halten, um die Einhaltung rechtlicher und ethischer Standards in Forschung und Experiment zu gewährleisten.