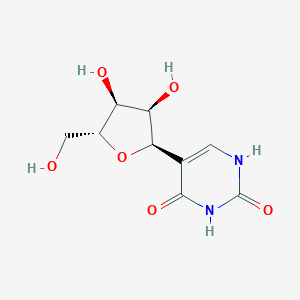
Pseudouridine B
Übersicht
Beschreibung
It is the most abundant RNA modification in cellular RNA and is found in various types of RNA, including transfer RNA, ribosomal RNA, small nuclear RNA, and small nucleolar RNA . Pseudouridine B is unique because it contains a carbon-carbon glycosidic bond instead of the usual nitrogen-carbon bond found in uridine . This modification enhances the stability and functionality of RNA molecules.
Vorbereitungsmethoden
Synthetic Routes and Reaction Conditions: The synthesis of pseudouridine B involves the use of adenosine-5’-monophosphate and uracil as starting materials. The process includes a reverse reaction catalyzed by pseudouridine monophosphate glycosidase . This semi-enzymatic synthetic route is preferred due to its efficiency and specificity.
Industrial Production Methods: Industrial production of this compound typically involves large-scale enzymatic synthesis. The enzymes used in this process are highly specific and efficient, allowing for the production of large quantities of this compound with high purity .
Analyse Chemischer Reaktionen
Types of Reactions: Pseudouridine B undergoes various chemical reactions, including:
Oxidation: this compound can be oxidized under specific conditions, leading to the formation of oxidized derivatives.
Reduction: Reduction reactions can convert this compound into its reduced forms.
Substitution: this compound can participate in substitution reactions, where specific groups are replaced by other functional groups.
Common Reagents and Conditions:
Oxidation: Common oxidizing agents include hydrogen peroxide and potassium permanganate.
Reduction: Reducing agents such as sodium borohydride and lithium aluminum hydride are commonly used.
Substitution: Substitution reactions often involve reagents like alkyl halides and nucleophiles under controlled conditions.
Major Products:
Wissenschaftliche Forschungsanwendungen
Pseudouridine B has numerous applications in scientific research, including:
Wirkmechanismus
Pseudouridine B enhances the stability of RNA molecules by influencing their secondary conformation. The isomerization process involves the breakage of the nitrogen-carbon bond and the formation of a carbon-carbon bond, which increases the rotational freedom and conformational flexibility of the RNA molecule . This modification also introduces an extra hydrogen bond donor, which can participate in novel molecular interactions, further stabilizing the RNA structure .
Vergleich Mit ähnlichen Verbindungen
Uridine: The parent compound of pseudouridine B, which contains a nitrogen-carbon glycosidic bond.
N1-methylpseudouridine: A modified form of this compound that has been used in mRNA vaccines for COVID-19.
Comparison: this compound is unique due to its carbon-carbon glycosidic bond, which provides greater stability and conformational flexibility compared to uridine . N1-methylpseudouridine, on the other hand, offers enhanced stability and reduced immunogenicity, making it suitable for therapeutic applications .
Biologische Aktivität
Pseudouridine (Ψ), often referred to as the "fifth nucleotide," is a naturally occurring modified nucleoside found in various RNA types, including mRNA, tRNA, and rRNA. Its biological activity has garnered significant attention due to its roles in RNA stability, translation efficiency, and regulation of gene expression. This article delves into the biological activity of Pseudouridine B, highlighting its mechanisms, effects on cellular processes, and implications for therapeutic applications.
Overview of this compound
Pseudouridine is formed by the isomerization of uridine, resulting in a stable C–C glycosidic bond between the nucleobase and ribose. This modification enhances the structural integrity of RNA and influences various biological functions. This compound specifically refers to its incorporation into RNA molecules, which has been shown to significantly affect translation and immune response.
-
Translation Efficiency :
- Research indicates that mRNAs containing pseudouridine exhibit enhanced translational capacity compared to their unmodified counterparts. Studies have demonstrated that pseudouridine-containing mRNAs activate the protein kinase R (PKR) to a lesser extent, allowing for more efficient translation without repression from innate immune responses .
- In vitro experiments have shown that pseudouridine-modified mRNAs lead to higher luciferase activity in mammalian cells, suggesting improved translational outcomes in vivo .
- RNA Stability :
-
Regulation of Gene Expression :
- Pseudouridylation has been linked to alternative splicing and the regulation of microRNA biogenesis. For instance, the pseudouridine synthase TruB1 enhances the maturation of let-7 microRNAs by interacting with primary transcripts . This suggests a broader role for pseudouridine in post-transcriptional regulation.
Case Study 1: Enhanced mRNA Vaccines
A study investigated the effects of incorporating pseudouridine into mRNA vaccines. The results indicated that these modified mRNAs not only exhibited reduced immunogenicity but also demonstrated superior protein expression levels when administered in vivo. Specifically, luciferase activity was found to be significantly higher (up to 78-fold) in mice injected with pseudouridine-modified mRNA compared to unmodified mRNA .
Case Study 2: Cancer Research
Research has highlighted the role of pseudouridine modifications in various cancers. For example, alterations in rRNA uridine modification due to DKC1 deficiency have been linked to disrupted ribosomal function and cancer progression . Furthermore, pseudouridylation has been implicated in the development of digestive system cancers such as colorectal and liver cancer .
Data Tables
Eigenschaften
IUPAC Name |
5-[(2R,3R,4S,5R)-3,4-dihydroxy-5-(hydroxymethyl)oxolan-2-yl]-1H-pyrimidine-2,4-dione | |
---|---|---|
Source | PubChem | |
URL | https://pubchem.ncbi.nlm.nih.gov | |
Description | Data deposited in or computed by PubChem | |
InChI |
InChI=1S/C9H12N2O6/c12-2-4-5(13)6(14)7(17-4)3-1-10-9(16)11-8(3)15/h1,4-7,12-14H,2H2,(H2,10,11,15,16)/t4-,5-,6-,7-/m1/s1 | |
Source | PubChem | |
URL | https://pubchem.ncbi.nlm.nih.gov | |
Description | Data deposited in or computed by PubChem | |
InChI Key |
PTJWIQPHWPFNBW-DBRKOABJSA-N | |
Source | PubChem | |
URL | https://pubchem.ncbi.nlm.nih.gov | |
Description | Data deposited in or computed by PubChem | |
Canonical SMILES |
C1=C(C(=O)NC(=O)N1)C2C(C(C(O2)CO)O)O | |
Source | PubChem | |
URL | https://pubchem.ncbi.nlm.nih.gov | |
Description | Data deposited in or computed by PubChem | |
Isomeric SMILES |
C1=C(C(=O)NC(=O)N1)[C@@H]2[C@@H]([C@@H]([C@H](O2)CO)O)O | |
Source | PubChem | |
URL | https://pubchem.ncbi.nlm.nih.gov | |
Description | Data deposited in or computed by PubChem | |
Molecular Formula |
C9H12N2O6 | |
Source | PubChem | |
URL | https://pubchem.ncbi.nlm.nih.gov | |
Description | Data deposited in or computed by PubChem | |
Molecular Weight |
244.20 g/mol | |
Source | PubChem | |
URL | https://pubchem.ncbi.nlm.nih.gov | |
Description | Data deposited in or computed by PubChem | |
Retrosynthesis Analysis
AI-Powered Synthesis Planning: Our tool employs the Template_relevance Pistachio, Template_relevance Bkms_metabolic, Template_relevance Pistachio_ringbreaker, Template_relevance Reaxys, Template_relevance Reaxys_biocatalysis model, leveraging a vast database of chemical reactions to predict feasible synthetic routes.
One-Step Synthesis Focus: Specifically designed for one-step synthesis, it provides concise and direct routes for your target compounds, streamlining the synthesis process.
Accurate Predictions: Utilizing the extensive PISTACHIO, BKMS_METABOLIC, PISTACHIO_RINGBREAKER, REAXYS, REAXYS_BIOCATALYSIS database, our tool offers high-accuracy predictions, reflecting the latest in chemical research and data.
Strategy Settings
Precursor scoring | Relevance Heuristic |
---|---|
Min. plausibility | 0.01 |
Model | Template_relevance |
Template Set | Pistachio/Bkms_metabolic/Pistachio_ringbreaker/Reaxys/Reaxys_biocatalysis |
Top-N result to add to graph | 6 |
Feasible Synthetic Routes
Haftungsausschluss und Informationen zu In-Vitro-Forschungsprodukten
Bitte beachten Sie, dass alle Artikel und Produktinformationen, die auf BenchChem präsentiert werden, ausschließlich zu Informationszwecken bestimmt sind. Die auf BenchChem zum Kauf angebotenen Produkte sind speziell für In-vitro-Studien konzipiert, die außerhalb lebender Organismen durchgeführt werden. In-vitro-Studien, abgeleitet von dem lateinischen Begriff "in Glas", beinhalten Experimente, die in kontrollierten Laborumgebungen unter Verwendung von Zellen oder Geweben durchgeführt werden. Es ist wichtig zu beachten, dass diese Produkte nicht als Arzneimittel oder Medikamente eingestuft sind und keine Zulassung der FDA für die Vorbeugung, Behandlung oder Heilung von medizinischen Zuständen, Beschwerden oder Krankheiten erhalten haben. Wir müssen betonen, dass jede Form der körperlichen Einführung dieser Produkte in Menschen oder Tiere gesetzlich strikt untersagt ist. Es ist unerlässlich, sich an diese Richtlinien zu halten, um die Einhaltung rechtlicher und ethischer Standards in Forschung und Experiment zu gewährleisten.