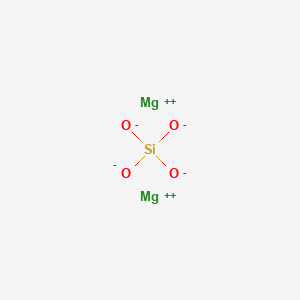
Magnesium orthosilicate
- Klicken Sie auf QUICK INQUIRY, um ein Angebot von unserem Expertenteam zu erhalten.
- Mit qualitativ hochwertigen Produkten zu einem WETTBEWERBSFÄHIGEN Preis können Sie sich mehr auf Ihre Forschung konzentrieren.
Beschreibung
Magnesium orthosilicate, also known as this compound, is a useful research compound. Its molecular formula is Mg2O4Si and its molecular weight is 140.69 g/mol. The purity is usually 95%.
The exact mass of the compound this compound is unknown and the complexity rating of the compound is unknown. Its Medical Subject Headings (MeSH) category is Chemicals and Drugs Category - Inorganic Chemicals - Silicon Compounds - Supplementary Records. The storage condition is unknown. Please store according to label instructions upon receipt of goods.Use and application categories indicated by third-party sources: Cosmetics -> Absorbent; Anticaking; Bulking; Opacifying; Viscosity controlling. However, this does not mean our product can be used or applied in the same or a similar way.
BenchChem offers high-quality this compound suitable for many research applications. Different packaging options are available to accommodate customers' requirements. Please inquire for more information about this compound including the price, delivery time, and more detailed information at info@benchchem.com.
Eigenschaften
CAS-Nummer |
10034-94-3 |
---|---|
Molekularformel |
Mg2O4Si |
Molekulargewicht |
140.69 g/mol |
IUPAC-Name |
dimagnesium;silicate |
InChI |
InChI=1S/2Mg.O4Si/c;;1-5(2,3)4/q2*+2;-4 |
InChI-Schlüssel |
HCWCAKKEBCNQJP-UHFFFAOYSA-N |
SMILES |
[O-][Si]([O-])([O-])[O-].[Mg+2].[Mg+2] |
Kanonische SMILES |
[O-][Si]([O-])([O-])[O-].[Mg+2].[Mg+2] |
Key on ui other cas no. |
10034-94-3 |
Synonyme |
forsterite Mg2SiO4 |
Herkunft des Produkts |
United States |
Synthesis routes and methods
Procedure details
Haftungsausschluss und Informationen zu In-Vitro-Forschungsprodukten
Bitte beachten Sie, dass alle Artikel und Produktinformationen, die auf BenchChem präsentiert werden, ausschließlich zu Informationszwecken bestimmt sind. Die auf BenchChem zum Kauf angebotenen Produkte sind speziell für In-vitro-Studien konzipiert, die außerhalb lebender Organismen durchgeführt werden. In-vitro-Studien, abgeleitet von dem lateinischen Begriff "in Glas", beinhalten Experimente, die in kontrollierten Laborumgebungen unter Verwendung von Zellen oder Geweben durchgeführt werden. Es ist wichtig zu beachten, dass diese Produkte nicht als Arzneimittel oder Medikamente eingestuft sind und keine Zulassung der FDA für die Vorbeugung, Behandlung oder Heilung von medizinischen Zuständen, Beschwerden oder Krankheiten erhalten haben. Wir müssen betonen, dass jede Form der körperlichen Einführung dieser Produkte in Menschen oder Tiere gesetzlich strikt untersagt ist. Es ist unerlässlich, sich an diese Richtlinien zu halten, um die Einhaltung rechtlicher und ethischer Standards in Forschung und Experiment zu gewährleisten.