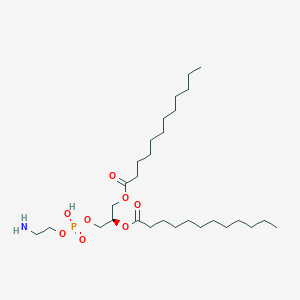
1,2-Dilauroyl-sn-glycero-3-phosphoethanolamine
Übersicht
Beschreibung
1,2-Dilauroyl-sn-glycero-3-phosphoethanolamine (DLPE) is a water-soluble derivative of phosphatidylethanolamine with lauric acid (12:0) acyl chains . It is used to prepare anionic liposomes and binary giant unilamellar vesicles .
Molecular Structure Analysis
The molecular formula of DLPE is C29H58NO8P . The IUPAC name is [(2R)-3-[2-aminoethoxy(hydroxy)phosphoryl]oxy-2-dodecanoyloxypropyl] dodecanoate . The molecular weight is 579.75 .Wissenschaftliche Forschungsanwendungen
Component of Cell Membranes
DLPE is a phospholipid that is one of the major components of cell membranes in bacteria and in the nervous system . It plays a crucial role in maintaining the integrity and functionality of these cells.
Liposome Production
DLPE is commonly used in the generation of liposomes . Liposomes are spherical vesicles that have at least one lipid bilayer. They are used as a vehicle for administration of nutrients and pharmaceutical drugs.
Micelle Formation
DLPE is also used in the generation of micelles . Micelles are lipid molecules that arrange themselves in a spherical form in aqueous solutions. They are used in a variety of industrial and consumer products, including cleaning products and cosmetics.
Drug Delivery Systems
DLPE-based particle drug delivery studies have shown promising results . Dispersions of DLPE have been shown to exhibit a metastable state which allows for the manipulation of delayed nucleation. This provides new avenues in the design of novel modalities for the drug delivery of therapeutic payloads.
Synthetic Lipid Research
DLPE is used in synthetic lipid research . It is used to study the properties and behaviors of synthetic lipids and their potential applications in various fields.
Metabolic Studies
DLPE is a metabolite produced during a metabolic reaction in Escherichia coli . Therefore, it can be used in studies related to bacterial metabolism.
Wirkmechanismus
Target of Action
1,2-Dilauroyl-sn-glycero-3-phosphoethanolamine (DLPE) is a derivative of phosphatidylethanolamine with lauric acid (12:0) acyl chains . It primarily targets cell membranes, where it can be used as liposomes .
Mode of Action
DLPE interacts with its targets by integrating into the lipid bilayer of cell membranes. As a liposome, it can encapsulate and deliver various bioactive agents, enhancing their cellular uptake .
Biochemical Pathways
As a phosphatidylethanolamine derivative, it may influence the synthesis and metabolism of phospholipids .
Pharmacokinetics
As a liposome, it can potentially enhance the bioavailability of encapsulated drugs by facilitating their delivery to target cells .
Result of Action
The molecular and cellular effects of DLPE’s action largely depend on the bioactive agents it delivers as a liposome. For instance, it has been used to efficiently deliver genes into human cancer cells .
Eigenschaften
IUPAC Name |
[(2R)-3-[2-aminoethoxy(hydroxy)phosphoryl]oxy-2-dodecanoyloxypropyl] dodecanoate | |
---|---|---|
Source | PubChem | |
URL | https://pubchem.ncbi.nlm.nih.gov | |
Description | Data deposited in or computed by PubChem | |
InChI |
InChI=1S/C29H58NO8P/c1-3-5-7-9-11-13-15-17-19-21-28(31)35-25-27(26-37-39(33,34)36-24-23-30)38-29(32)22-20-18-16-14-12-10-8-6-4-2/h27H,3-26,30H2,1-2H3,(H,33,34)/t27-/m1/s1 | |
Source | PubChem | |
URL | https://pubchem.ncbi.nlm.nih.gov | |
Description | Data deposited in or computed by PubChem | |
InChI Key |
ZLGYVWRJIZPQMM-HHHXNRCGSA-N | |
Source | PubChem | |
URL | https://pubchem.ncbi.nlm.nih.gov | |
Description | Data deposited in or computed by PubChem | |
Canonical SMILES |
CCCCCCCCCCCC(=O)OCC(COP(=O)(O)OCCN)OC(=O)CCCCCCCCCCC | |
Source | PubChem | |
URL | https://pubchem.ncbi.nlm.nih.gov | |
Description | Data deposited in or computed by PubChem | |
Isomeric SMILES |
CCCCCCCCCCCC(=O)OC[C@H](COP(=O)(O)OCCN)OC(=O)CCCCCCCCCCC | |
Source | PubChem | |
URL | https://pubchem.ncbi.nlm.nih.gov | |
Description | Data deposited in or computed by PubChem | |
Molecular Formula |
C29H58NO8P | |
Source | PubChem | |
URL | https://pubchem.ncbi.nlm.nih.gov | |
Description | Data deposited in or computed by PubChem | |
Molecular Weight |
579.7 g/mol | |
Source | PubChem | |
URL | https://pubchem.ncbi.nlm.nih.gov | |
Description | Data deposited in or computed by PubChem | |
CAS RN |
59752-57-7 | |
Record name | 1,2-Dilauroyl-sn-glycero-3-phosphoethanolamine | |
Source | CAS Common Chemistry | |
URL | https://commonchemistry.cas.org/detail?cas_rn=59752-57-7 | |
Description | CAS Common Chemistry is an open community resource for accessing chemical information. Nearly 500,000 chemical substances from CAS REGISTRY cover areas of community interest, including common and frequently regulated chemicals, and those relevant to high school and undergraduate chemistry classes. This chemical information, curated by our expert scientists, is provided in alignment with our mission as a division of the American Chemical Society. | |
Explanation | The data from CAS Common Chemistry is provided under a CC-BY-NC 4.0 license, unless otherwise stated. | |
Record name | 1,2-Dilauroyl-sn-glycero-3-phosphoethanolamine | |
Source | ChemIDplus | |
URL | https://pubchem.ncbi.nlm.nih.gov/substance/?source=chemidplus&sourceid=0059752577 | |
Description | ChemIDplus is a free, web search system that provides access to the structure and nomenclature authority files used for the identification of chemical substances cited in National Library of Medicine (NLM) databases, including the TOXNET system. | |
Record name | (R)-1-[[[(2-aminoethoxy)hydroxyphosphinyl]oxy]methyl]ethylene dilaurate | |
Source | European Chemicals Agency (ECHA) | |
URL | https://echa.europa.eu/substance-information/-/substanceinfo/100.056.266 | |
Description | The European Chemicals Agency (ECHA) is an agency of the European Union which is the driving force among regulatory authorities in implementing the EU's groundbreaking chemicals legislation for the benefit of human health and the environment as well as for innovation and competitiveness. | |
Explanation | Use of the information, documents and data from the ECHA website is subject to the terms and conditions of this Legal Notice, and subject to other binding limitations provided for under applicable law, the information, documents and data made available on the ECHA website may be reproduced, distributed and/or used, totally or in part, for non-commercial purposes provided that ECHA is acknowledged as the source: "Source: European Chemicals Agency, http://echa.europa.eu/". Such acknowledgement must be included in each copy of the material. ECHA permits and encourages organisations and individuals to create links to the ECHA website under the following cumulative conditions: Links can only be made to webpages that provide a link to the Legal Notice page. | |
Record name | 1,2-DILAUROYL-SN-GLYCERO-3-PHOSPHOETHANOLAMINE | |
Source | FDA Global Substance Registration System (GSRS) | |
URL | https://gsrs.ncats.nih.gov/ginas/app/beta/substances/D1O2S89PCE | |
Description | The FDA Global Substance Registration System (GSRS) enables the efficient and accurate exchange of information on what substances are in regulated products. Instead of relying on names, which vary across regulatory domains, countries, and regions, the GSRS knowledge base makes it possible for substances to be defined by standardized, scientific descriptions. | |
Explanation | Unless otherwise noted, the contents of the FDA website (www.fda.gov), both text and graphics, are not copyrighted. They are in the public domain and may be republished, reprinted and otherwise used freely by anyone without the need to obtain permission from FDA. Credit to the U.S. Food and Drug Administration as the source is appreciated but not required. | |
Retrosynthesis Analysis
AI-Powered Synthesis Planning: Our tool employs the Template_relevance Pistachio, Template_relevance Bkms_metabolic, Template_relevance Pistachio_ringbreaker, Template_relevance Reaxys, Template_relevance Reaxys_biocatalysis model, leveraging a vast database of chemical reactions to predict feasible synthetic routes.
One-Step Synthesis Focus: Specifically designed for one-step synthesis, it provides concise and direct routes for your target compounds, streamlining the synthesis process.
Accurate Predictions: Utilizing the extensive PISTACHIO, BKMS_METABOLIC, PISTACHIO_RINGBREAKER, REAXYS, REAXYS_BIOCATALYSIS database, our tool offers high-accuracy predictions, reflecting the latest in chemical research and data.
Strategy Settings
Precursor scoring | Relevance Heuristic |
---|---|
Min. plausibility | 0.01 |
Model | Template_relevance |
Template Set | Pistachio/Bkms_metabolic/Pistachio_ringbreaker/Reaxys/Reaxys_biocatalysis |
Top-N result to add to graph | 6 |
Feasible Synthetic Routes
Q & A
Q1: How does DLPE influence the characteristics of model lipid membranes?
A1: DLPE can significantly alter the properties of model membranes. For instance, when incorporated into monolayers with other phospholipids, DLPE modifies membrane fluidity and surface potential. [] This effect is also observed in bilayer systems, impacting the diffusion of water molecules within the membrane. [] Moreover, the presence of DLPE can influence the activity of enzymes like phospholipase A2, highlighting its role in modulating membrane-protein interactions. [, ]
Q2: Can you elaborate on the phase behavior of DLPE in model membrane systems?
A2: DLPE exhibits distinct phase behavior in monolayers depending on the lateral pressure. At room temperature, it exists in a liquid expanded (LE) phase below a specific surface pressure, beyond which it transitions into a liquid condensed (LC) phase. [] This transition is marked by a noticeable increase in surface viscosity and compressibility modulus. []
Q3: What are the advantages of using DLPE in liposome preparations?
A3: DLPE is often incorporated into liposome formulations due to its ability to influence liposome size and morphology. [, ] Studies utilizing Coarse Grained Molecular Dynamics (CGMD) simulations have demonstrated that the inclusion of DLPE allows for predicting the resulting liposome size, which aligns with empirical observations. [] This predictability is crucial for controlled drug delivery applications.
Q4: How is DLPE utilized in studying protein-membrane interactions?
A4: Researchers utilize modified DLPE molecules, such as those containing photoactivatable groups, to investigate protein interactions at the membrane interface. [] By incorporating a photoactivatable 1,2-Dilauroyl-sn-glycero-3-phosphoethanolamine derivative (ASA-DLPE) into model membranes, researchers can covalently crosslink proteins interacting with the membrane upon UV irradiation. [] This technique enables the identification of membrane-interacting proteins and provides valuable insights into their function.
Q5: What is the role of computational chemistry in understanding DLPE behavior?
A5: Molecular dynamics simulations, specifically CGMD, have proven invaluable in predicting the behavior of DLPE in liposome formulations. [] These simulations can accurately predict liposome size, which is consistent with experimental findings. [] Such computational approaches offer valuable insights into the self-assembly processes of DLPE and its interactions with other molecules.
Q6: Are there any studies on enzymatic modifications of DLPE?
A6: Yes, researchers have explored enzymatic modifications of DLPE for various applications. For instance, phospholipase D (PLD) has been successfully employed to catalyze the transphosphatidylation of DLPE with ethanolamine, leading to the production of 1,2-dilauroyl-sn-glycero-3-phosphoethanolamine. [] This enzymatic approach highlights the potential for modifying DLPE's headgroup for targeted applications.
Q7: Can you describe the structural characteristics of DLPE?
A7: DLPE comprises a glycerol backbone with two lauroyl (dodecanoyl) chains esterified at the sn-1 and sn-2 positions, and a phosphoethanolamine group attached to the sn-3 position. While specific spectroscopic data may vary depending on the study and techniques employed, techniques like neutron diffraction have been used to determine the conformation of DLPE in gel phases, revealing an all-trans conformation of the lauroyl chains and a specific alignment of the zwitterionic dipoles in the headgroups. []
Q8: Is there any information available on the stability of DLPE?
A8: While specific stability data for DLPE might require further investigation, research suggests that giant vesicles produced with DLPE and other phospholipids exhibit stability during long-term storage at 4°C. [] This finding indicates potential for storage and utilization of DLPE-containing formulations, but further research is needed to comprehensively assess its stability under various conditions.
Haftungsausschluss und Informationen zu In-Vitro-Forschungsprodukten
Bitte beachten Sie, dass alle Artikel und Produktinformationen, die auf BenchChem präsentiert werden, ausschließlich zu Informationszwecken bestimmt sind. Die auf BenchChem zum Kauf angebotenen Produkte sind speziell für In-vitro-Studien konzipiert, die außerhalb lebender Organismen durchgeführt werden. In-vitro-Studien, abgeleitet von dem lateinischen Begriff "in Glas", beinhalten Experimente, die in kontrollierten Laborumgebungen unter Verwendung von Zellen oder Geweben durchgeführt werden. Es ist wichtig zu beachten, dass diese Produkte nicht als Arzneimittel oder Medikamente eingestuft sind und keine Zulassung der FDA für die Vorbeugung, Behandlung oder Heilung von medizinischen Zuständen, Beschwerden oder Krankheiten erhalten haben. Wir müssen betonen, dass jede Form der körperlichen Einführung dieser Produkte in Menschen oder Tiere gesetzlich strikt untersagt ist. Es ist unerlässlich, sich an diese Richtlinien zu halten, um die Einhaltung rechtlicher und ethischer Standards in Forschung und Experiment zu gewährleisten.