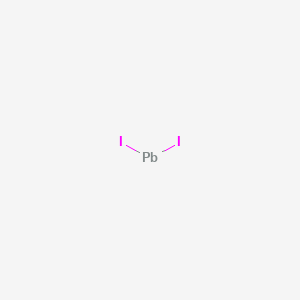
Blei(II)-iodid
- Klicken Sie auf QUICK INQUIRY, um ein Angebot von unserem Expertenteam zu erhalten.
- Mit qualitativ hochwertigen Produkten zu einem WETTBEWERBSFÄHIGEN Preis können Sie sich mehr auf Ihre Forschung konzentrieren.
Übersicht
Beschreibung
Lead(II) iodide is a chemical compound with the formula PbI2. It varies in color from yellow to red, depending on the temperature . At room temperature, it is a bright yellow odorless crystalline solid, that becomes orange and red when heated . It has nonlinear optical, ferroelectric, and ferroelastic properties .
Synthesis Analysis
Lead(II) iodide is commonly synthesized via a precipitation reaction between potassium iodide (KI) and lead (II) nitrate . A versatile lead iodide particle synthesis and film surface analysis for optoelectronics has been reported .Molecular Structure Analysis
The structure of PbI2, as determined by X-ray powder diffraction, is primarily hexagonal close-packed system with alternating between layers of lead atoms and iodide atoms, with largely ionic bonding . The crystal structure of the title compound has been determined by X-ray diffractometry .Chemical Reactions Analysis
Lead(II) iodide reacts with potassium iodide forming lead (II) iodide and potassium nitrate . If you add colorless potassium iodide solution (or any other source of iodide ions in solution) to a solution of lead (II) nitrate, a bright yellow precipitate of lead (II) iodide is produced .Physical And Chemical Properties Analysis
Lead(II) iodide is a bright yellow powder that is odorless . It has a density of 6.16 g/cm3 . The melting point of Lead iodide is 410 °C (770 °F; 683 K) and the boiling point is 872 °C (1,602 °F; 1,145 K) . It is insoluble in most liquids, barring boiling water and potassium iodine .Wissenschaftliche Forschungsanwendungen
Strahlungsdetektion
Blei(II)-iodid wird als Detektormaterial für hochenergetische Photonen verwendet, darunter Röntgen- und Gammastrahlen. Seine ferroelastischen Eigenschaften und seine Effizienz beim Stoppen von Röntgen- und Gammastrahlen sorgen für eine hervorragende Umweltstabilität .
Fotografie und Druck
In den Bereichen Fotografie und Druck wird this compound aufgrund seiner lichtempfindlichen Eigenschaften verwendet. Es hilft bei der Aufnahme von Bildern und der Erstellung von Drucken mit hoher Präzision .
Mosaikgold und Bronzierung
This compound wird zur Erzeugung von Mosaikgold- und Bronzierungseffekten eingesetzt, wahrscheinlich aufgrund seiner leuchtend gelben Farbe und seiner reflektierenden Eigenschaften .
Strahlenschutzmaterialien
Studien haben gezeigt, dass this compound in Verbundwerkstoffe eingearbeitet werden kann, um deren GammastrahlenschutzEigenschaften zu verbessern. Dies umfasst die Verbesserung der linearen und massenspezifischen Schwächungskoeffizienten, der effektiven Ordnungszahl (Z_eff) und der effektiven Elektronendichte .
Chemische Experimente
Die Verbindung ist für das „goldener Regen“-Experiment bekannt, bei dem sie aus einer heißen Lösung durch Abkühlen umkristallisiert wird, wobei goldgelbe Kristalle entstehen. Dies zeigt seine Löslichkeits- und Umkristallisationseigenschaften .
Nichtlineare optische Kristalle
This compound ist Bestandteil neuer Blei-Iodid-Formiate, die eine starke zweite Harmonische Generation (SHG) aufweisen. Diese sind aufgrund ihrer nichtlinearen optischen (NLO) Eigenschaften für industrielle und kommerzielle Anwendungen gefragt .
Wirkmechanismus
Target of Action
Lead(II) iodide, also known as diiodolead, is a chemical compound with the formula PbI2 . It primarily targets the central nervous system (CNS), blood, kidney, and thyroid .
Mode of Action
Lead(II) iodide has nonlinear optical, ferroelectric, and ferroelastic properties . It has a wide semiconducting band gap (Eg=2.3 eV) which enables low noise operation at room temperature and above . It has high X-ray and gamma ray stopping efficiency .
Biochemical Pathways
Lead(II) iodide is involved in the synthesis of lead perovskite , a material used in optoelectronic devices . The synthesis process involves the degradation of a 2-D sheet of crystals of lead(II) iodide into 1-D fibrous intermediates, followed by Oswald ripening of perovskite crystals .
Pharmacokinetics
Lead(II) iodide is a bright yellow odorless crystalline solid . It has a molar mass of 461.01 g/mol , a melting point of 410 °C , and a boiling point of 872 °C . It is soluble in water (0.44 g/L at 0 °C, 0.76 g/L at 20 °C, and 4.1 g/L at 100 °C), but insoluble in ethanol and cold HCl . It is soluble in alkalis, KI solution, and methyl isopropyl ketone .
Result of Action
The compound’s action results in the formation of a bright yellow odorless crystalline solid that becomes orange and red when heated . It was formerly employed as a yellow pigment in some paints, with the name iodide yellow . That use has been largely discontinued due to its toxicity and poor stability .
Action Environment
Lead(II) iodide has excellent environmental stability . It can operate at low noise at room temperature and above due to its wide semiconducting band gap . It is decomposed by light at temperatures above 125 °c . This effect has been used in a patented photographic process .
Safety and Hazards
Zukünftige Richtungen
Lead(II) iodide has a few specialized applications, such as the manufacture of solar cells, X-rays and gamma-ray detectors . The prepared 2D perovskites demonstrated robust stability under ambient conditions as well as resistance to water . This could motivate the use of these long alkyl chain cation-based 2D perovskite materials in perovskite solar cells for achieving prolonged device stability but also for the next generation LEDs and display technologies .
Eigenschaften
IUPAC Name |
lead(2+);diiodide |
Source
|
---|---|---|
Details | Computed by LexiChem 2.6.6 (PubChem release 2019.06.18) | |
Source | PubChem | |
URL | https://pubchem.ncbi.nlm.nih.gov | |
Description | Data deposited in or computed by PubChem | |
InChI |
InChI=1S/2HI.Pb/h2*1H;/q;;+2/p-2 |
Source
|
Details | Computed by InChI 1.0.5 (PubChem release 2019.06.18) | |
Source | PubChem | |
URL | https://pubchem.ncbi.nlm.nih.gov | |
Description | Data deposited in or computed by PubChem | |
InChI Key |
RQQRAHKHDFPBMC-UHFFFAOYSA-L |
Source
|
Details | Computed by InChI 1.0.5 (PubChem release 2019.06.18) | |
Source | PubChem | |
URL | https://pubchem.ncbi.nlm.nih.gov | |
Description | Data deposited in or computed by PubChem | |
Canonical SMILES |
[I-].[I-].[Pb+2] |
Source
|
Details | Computed by OEChem 2.1.5 (PubChem release 2019.06.18) | |
Source | PubChem | |
URL | https://pubchem.ncbi.nlm.nih.gov | |
Description | Data deposited in or computed by PubChem | |
Molecular Formula |
I2Pb |
Source
|
Details | Computed by PubChem 2.1 (PubChem release 2019.06.18) | |
Source | PubChem | |
URL | https://pubchem.ncbi.nlm.nih.gov | |
Description | Data deposited in or computed by PubChem | |
Molecular Weight |
461 g/mol |
Source
|
Details | Computed by PubChem 2.1 (PubChem release 2021.08.13) | |
Source | PubChem | |
URL | https://pubchem.ncbi.nlm.nih.gov | |
Description | Data deposited in or computed by PubChem | |
Haftungsausschluss und Informationen zu In-Vitro-Forschungsprodukten
Bitte beachten Sie, dass alle Artikel und Produktinformationen, die auf BenchChem präsentiert werden, ausschließlich zu Informationszwecken bestimmt sind. Die auf BenchChem zum Kauf angebotenen Produkte sind speziell für In-vitro-Studien konzipiert, die außerhalb lebender Organismen durchgeführt werden. In-vitro-Studien, abgeleitet von dem lateinischen Begriff "in Glas", beinhalten Experimente, die in kontrollierten Laborumgebungen unter Verwendung von Zellen oder Geweben durchgeführt werden. Es ist wichtig zu beachten, dass diese Produkte nicht als Arzneimittel oder Medikamente eingestuft sind und keine Zulassung der FDA für die Vorbeugung, Behandlung oder Heilung von medizinischen Zuständen, Beschwerden oder Krankheiten erhalten haben. Wir müssen betonen, dass jede Form der körperlichen Einführung dieser Produkte in Menschen oder Tiere gesetzlich strikt untersagt ist. Es ist unerlässlich, sich an diese Richtlinien zu halten, um die Einhaltung rechtlicher und ethischer Standards in Forschung und Experiment zu gewährleisten.