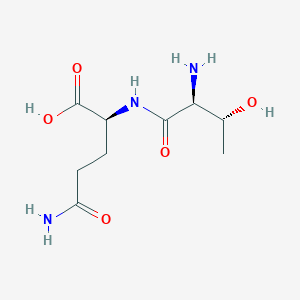
Thr-Gln
Übersicht
Beschreibung
Thr-Gln is a dipeptide composed of the amino acids threonine (Thr) and glutamine (Gln). It has been found in the repetitive domains of a cell surface adhesin from the Gram-positive organism Clostridium perfringens . These domains contain intramolecular ester bonds joining Thr and Gln side chains .
Synthesis Analysis
The synthesis of this compound involves various enzymes and pathways. For instance, a study on tea plants (Camellia sinensis) roots revealed that in the intermediate products of Lys, Thr, and Met synthesis from aspartate, the expression of ASA dehydrogenase (CsASADH) and aspartate kinase (CsAK) showed a trend of increasing first and then decreasing . Another study showed that tRNAs can be sheared and fragmented into small non-coding RNAs, also known as tRNA-derived small RNAs (tDRs), which have been shown to be essential for regulating cell proliferation, apoptosis, metastasis, and immunity .
Molecular Structure Analysis
The molecular structure of this compound involves the combination of the structures of threonine and glutamine. Threonine is an α-amino acid with the chemical formula HO2CCH(NH2)CH(OH)CH3. Glutamine is also an α-amino acid, and its side chain is similar to that of glutamic acid, except the carboxylic acid group is replaced by an amide .
Chemical Reactions Analysis
This compound undergoes various chemical reactions, particularly in biological systems. For instance, in a bacterial cell surface adhesin, this compound ester bond cross-links are generated autocatalytically by a serine protease-like mechanism .
Physical And Chemical Properties Analysis
The physical and chemical properties of this compound are influenced by the properties of its constituent amino acids, threonine and glutamine. For instance, glutamine is classified as a charge-neutral, polar amino acid .
Wissenschaftliche Forschungsanwendungen
Nanotechnology and Biosensors
The application of graphene-like two-dimensional layered nanomaterials (GLNs), including boron nitride nanosheets and graphitic-carbon nitride nanosheets, has been significant in various fields. These materials exhibit strong mechanical strength, high surface area, and remarkable biocompatibility, making them ideal for use in biosensors and nanomedicine. GLNs have shown potential in electrochemical and optical biosensors, bioimaging, drug delivery, and cancer therapy (Yang et al., 2015).
Biochemical Processes and Enzyme Function
Gamma-glutamyltranspeptidase (GGT) is an enzyme crucial in glutathione metabolism. It undergoes posttranslational cleavage between Gln-390 and Thr-391, with Thr-391 acting as the active residue in the catalytic reaction. This process illustrates the importance of the Thr-Gln bond in enzymatic functions and biochemical processes (Okada et al., 2007).
Dietary Supplements and Health
Studies on dietary glutamine (Gln) have shown its effects on the homeostasis of CD4+ T cells in mice with induced colitis. Gln administration was found to suppress Th1/Th17 and Th-associated cytokine expressions and upregulated the expression of T regulatory cells, which may modulate the balance of Th/Treg and reduce inflammatory reactions (Hsiung et al., 2014).
Molecular Biology and Protein Ligation
In molecular biology, a novel protein ligation technology uses spontaneously-formed this compound ester bonds found in cell-surface adhesins. This technology, acting as a "molecular superglue," is innovative for assembling and disassembling complex protein nanomaterials, demonstrating the versatility of the this compound bond in biochemical applications (Young et al., 2017).
Agricultural Research
In agriculture, the study of glutamine (Gln) in maize showed that mid-season leaf Gln can predict end-season grain yield and nitrogen content in maize, indicating the potential of Gln as a marker for plant health and productivity in response to nitrogen fertilization (Goron et al., 2017).
Zukünftige Richtungen
Eigenschaften
IUPAC Name |
5-amino-2-[(2-amino-3-hydroxybutanoyl)amino]-5-oxopentanoic acid | |
---|---|---|
Details | Computed by LexiChem 2.6.6 (PubChem release 2019.06.18) | |
Source | PubChem | |
URL | https://pubchem.ncbi.nlm.nih.gov | |
Description | Data deposited in or computed by PubChem | |
InChI |
InChI=1S/C9H17N3O5/c1-4(13)7(11)8(15)12-5(9(16)17)2-3-6(10)14/h4-5,7,13H,2-3,11H2,1H3,(H2,10,14)(H,12,15)(H,16,17) | |
Details | Computed by InChI 1.0.5 (PubChem release 2019.06.18) | |
Source | PubChem | |
URL | https://pubchem.ncbi.nlm.nih.gov | |
Description | Data deposited in or computed by PubChem | |
InChI Key |
BWUHENPAEMNGQJ-UHFFFAOYSA-N | |
Details | Computed by InChI 1.0.5 (PubChem release 2019.06.18) | |
Source | PubChem | |
URL | https://pubchem.ncbi.nlm.nih.gov | |
Description | Data deposited in or computed by PubChem | |
Canonical SMILES |
CC(C(C(=O)NC(CCC(=O)N)C(=O)O)N)O | |
Details | Computed by OEChem 2.1.5 (PubChem release 2019.06.18) | |
Source | PubChem | |
URL | https://pubchem.ncbi.nlm.nih.gov | |
Description | Data deposited in or computed by PubChem | |
Molecular Formula |
C9H17N3O5 | |
Details | Computed by PubChem 2.1 (PubChem release 2019.06.18) | |
Source | PubChem | |
URL | https://pubchem.ncbi.nlm.nih.gov | |
Description | Data deposited in or computed by PubChem | |
DSSTOX Substance ID |
DTXSID60394356 | |
Record name | Thr-Gln | |
Source | EPA DSSTox | |
URL | https://comptox.epa.gov/dashboard/DTXSID60394356 | |
Description | DSSTox provides a high quality public chemistry resource for supporting improved predictive toxicology. | |
Molecular Weight |
247.25 g/mol | |
Details | Computed by PubChem 2.1 (PubChem release 2021.05.07) | |
Source | PubChem | |
URL | https://pubchem.ncbi.nlm.nih.gov | |
Description | Data deposited in or computed by PubChem | |
CAS RN |
96337-79-0 | |
Record name | Thr-Gln | |
Source | EPA DSSTox | |
URL | https://comptox.epa.gov/dashboard/DTXSID60394356 | |
Description | DSSTox provides a high quality public chemistry resource for supporting improved predictive toxicology. | |
Retrosynthesis Analysis
AI-Powered Synthesis Planning: Our tool employs the Template_relevance Pistachio, Template_relevance Bkms_metabolic, Template_relevance Pistachio_ringbreaker, Template_relevance Reaxys, Template_relevance Reaxys_biocatalysis model, leveraging a vast database of chemical reactions to predict feasible synthetic routes.
One-Step Synthesis Focus: Specifically designed for one-step synthesis, it provides concise and direct routes for your target compounds, streamlining the synthesis process.
Accurate Predictions: Utilizing the extensive PISTACHIO, BKMS_METABOLIC, PISTACHIO_RINGBREAKER, REAXYS, REAXYS_BIOCATALYSIS database, our tool offers high-accuracy predictions, reflecting the latest in chemical research and data.
Strategy Settings
Precursor scoring | Relevance Heuristic |
---|---|
Min. plausibility | 0.01 |
Model | Template_relevance |
Template Set | Pistachio/Bkms_metabolic/Pistachio_ringbreaker/Reaxys/Reaxys_biocatalysis |
Top-N result to add to graph | 6 |
Feasible Synthetic Routes
Q & A
Q1: What is the molecular formula and weight of Thr-Gln?
A1: this compound (Threonylglutamine) comprises two amino acids, threonine and glutamine, linked by a peptide bond. Its molecular formula is C8H15N3O5, and its molecular weight is 233.22 g/mol.
Q2: Does the dipeptide this compound itself have any known independent biological activity?
A2: The provided research focuses on this compound within the context of larger peptides and proteins. While this compound is a naturally occurring dipeptide, its independent biological activity is not directly addressed in these studies.
Q3: What is the significance of the this compound sequence in proteins?
A3: The this compound sequence can play various roles depending on the protein context. For instance, in the Forkhead-associated (FHA) domain, it serves as a recognition site for phosphorylation. The FHA domain of the DNA damage-response kinase Dun1 shows high specificity for a doubly phosphorylated this compound cluster. []
Q4: Are there specific examples of this compound contributing to protein function?
A4: Yes, research suggests the this compound sequence in high molecular weight kininogen plays a role in modulating thrombin-induced platelet aggregation. Specifically, the heptapeptide Leu-Ala-This compound-Leu-Gln-Ala, derived from kininogen, showed inhibitory activity against this process. []
Q5: Is this compound involved in any post-translational modifications?
A5: Yes, this compound can be a target for phosphorylation. The threonine residue within this sequence is often phosphorylated by kinases, leading to changes in protein conformation and function. [] This phosphorylation is crucial for specific protein interactions, as exemplified by the FHA domain recognizing the phosphorylated this compound motif.
Q6: What is known about the role of this compound in plant growth factors?
A6: While the research doesn't directly focus on this compound within plant growth factors, it highlights the importance of the sulfated pentapeptide phytosulfokine-α (PSK-α), Tyr(SO3H)-Ile-Tyr(SO3H)-This compound. [, , ] Although the specific role of the this compound portion isn't isolated, the entire peptide is crucial for plant cell proliferation.
Q7: What analytical techniques are commonly used to study peptides containing this compound?
A7: Researchers employ a variety of methods, including:
- Edman degradation: Used to determine the amino acid sequence of peptides and proteins. [, , , ]
- Mass spectrometry: Provides information about the mass-to-charge ratio of ions, useful for identifying and characterizing peptides. [, , , ]
- High-performance liquid chromatography (HPLC): A technique for separating, identifying, and quantifying components of a mixture. It is often coupled with mass spectrometry for peptide analysis. [, ]
- Site-directed mutagenesis: Allows researchers to introduce specific mutations into DNA sequences, enabling the study of the functional consequences of amino acid substitutions within peptides and proteins. []
Haftungsausschluss und Informationen zu In-Vitro-Forschungsprodukten
Bitte beachten Sie, dass alle Artikel und Produktinformationen, die auf BenchChem präsentiert werden, ausschließlich zu Informationszwecken bestimmt sind. Die auf BenchChem zum Kauf angebotenen Produkte sind speziell für In-vitro-Studien konzipiert, die außerhalb lebender Organismen durchgeführt werden. In-vitro-Studien, abgeleitet von dem lateinischen Begriff "in Glas", beinhalten Experimente, die in kontrollierten Laborumgebungen unter Verwendung von Zellen oder Geweben durchgeführt werden. Es ist wichtig zu beachten, dass diese Produkte nicht als Arzneimittel oder Medikamente eingestuft sind und keine Zulassung der FDA für die Vorbeugung, Behandlung oder Heilung von medizinischen Zuständen, Beschwerden oder Krankheiten erhalten haben. Wir müssen betonen, dass jede Form der körperlichen Einführung dieser Produkte in Menschen oder Tiere gesetzlich strikt untersagt ist. Es ist unerlässlich, sich an diese Richtlinien zu halten, um die Einhaltung rechtlicher und ethischer Standards in Forschung und Experiment zu gewährleisten.