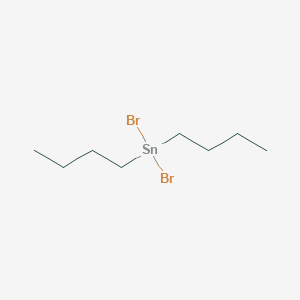
Dibutyltin dibromide
Übersicht
Beschreibung
Dibutyltin dibromide (DBTB) is an organotin compound characterized by two butyl groups and two bromine atoms bonded to a central tin atom. Organotin compounds are widely utilized as stabilizers in PVC production, catalysts in chemical synthesis, and biocides. Its structural analogs, such as dibutyltin dichloride (DBTC), diacetate (DBTA), dilaurate (DBTL), and maleate (DBTM), have been extensively studied for their biological and toxicological effects, particularly their endocrine-disrupting properties .
Vorbereitungsmethoden
Common Synthetic Routes
Halogen Exchange from Dibutyltin Dichloride
A widely used method for preparing dibutyltin dibromide involves the halogen exchange reaction of dibutyltin dichloride with a bromide source, such as hydrobromic acid or metal bromides (e.g., sodium bromide). This reaction typically proceeds in an organic solvent medium under reflux conditions.
-
$$
\text{(C}4\text{H}9)2\text{SnCl}2 + 2 \text{NaBr} \rightarrow \text{(C}4\text{H}9)2\text{SnBr}2 + 2 \text{NaCl}
$$ -
- Solvent: Toluene, ethanol, or other organic solvents
- Temperature: Reflux (approximately 60–110 °C depending on solvent)
- Time: Several hours (typically 3–6 h)
- Molar ratio: 1:2 (dibutyltin dichloride to sodium bromide)
Purification: The product is isolated by filtration to remove sodium chloride, followed by solvent evaporation and recrystallization.
Direct Bromination of Dibutyltin Oxide or Tetrabutyltin
Another approach involves brominating dibutyltin oxide or tetrabutyltin with bromine or brominating agents under controlled conditions.
-
$$
\text{(C}4\text{H}9)2\text{SnO} + \text{Br}2 \rightarrow \text{(C}4\text{H}9)2\text{SnBr}2 + \text{H}_2\text{O}
$$ -
- Solvent: Non-polar solvents such as hexane or toluene
- Temperature: 0–25 °C to avoid side reactions
- Bromine is added dropwise to control exothermicity.
Notes: This method requires careful handling due to the reactivity of bromine and potential side reactions.
Detailed Procedure from Recent Research Findings
While direct literature on this compound preparation is limited, analogous preparation methods for dibutyltin dichloride and dibutyltin oxide provide insight into optimized synthetic protocols adaptable for dibromide synthesis.
Preparation via Dibutyltin Dichloride Intermediates
Step 1: Synthesis of dibutyltin dichloride from tetrabutyltin and tin tetrachloride using catalysts such as modified nanometer cellulose and aluminum chloride compounds. This step yields dibutyltin dichloride with purity above 98.5% under controlled temperatures (60–138 °C) and reaction times (3–4 h).
Step 2: Halogen exchange with sodium bromide in an organic solvent (e.g., ethanol or toluene) at 50–70 °C with stirring for 2–5 hours.
Step 3: Separation of the organic phase containing this compound, followed by solvent recovery via distillation and vacuum drying to obtain the final product.
Use of Surfactants and Solvent Systems
Research indicates that surfactants such as normal heptane, toluene, or ethanol can facilitate the reaction by promoting phase transfer and improving yield and purity. The surfactant concentration is typically maintained at a mass ratio of 1:500 to 1:650 relative to dibutyltin chloride or its analogs.
Reaction Parameters and Optimization
Parameter | Typical Range / Value | Notes |
---|---|---|
Starting material purity | 98–99.5% (dibutyltin chloride) | High purity required for better yield and product quality |
Alkali solution concentration | 5–15% (NaOH or KOH) | Used in related oxide preparation, may be adapted for halogen exchange |
Molar ratio (dibutyltin:halide) | 1:2–3 | Ensures complete halogen substitution |
Reaction temperature | 45–70 °C | Controlled to avoid decomposition |
Reaction time | 1–5 hours | Sufficient for complete conversion |
Surfactant mass ratio | 1:500–1:650 (surfactant:dibutyltin) | Enhances phase transfer and product isolation |
Vacuum drying pressure | <0.01 MPa | For final product drying |
Drying temperature | 70–85 °C | Maintains product integrity |
Summary of Preparation Methodology
Step | Description | Conditions & Notes |
---|---|---|
1 | Dissolve dibutyltin chloride in organic solvent + surfactant | Stir at 50–70 °C; surfactant ratio 1:500–650 |
2 | Add sodium bromide solution dropwise | Rate: 8–20 kg/min; maintain temperature 45–65 °C |
3 | Stir isothermally for 1–4 hours | Ensures complete halogen exchange |
4 | Separate organic phase via stratification | 1–2 hours; removes aqueous impurities |
5 | Distill off solvent and surfactant | Temperature 78–98 °C; solvent recovery |
6 | Centrifugal washing and vacuum drying | Dry at 70–85 °C under vacuum <0.01 MPa |
7 | Pulverize to obtain pure this compound | Final product is white crystalline powder |
Research Findings and Notes
The use of surfactants and organic solvents is crucial to achieving high purity and yield by facilitating the phase separation of product from aqueous impurities, especially chloride ions.
The molar ratio of dibutyltin chloride to alkali (or bromide source) must be carefully controlled to avoid incomplete substitution or side reactions.
Vacuum drying under controlled temperature and pressure preserves the product's structural integrity and removes residual solvents.
The reaction is sensitive to temperature and addition rates; slow addition of sodium bromide and controlled stirring prevent local concentration spikes and side reactions.
High-purity starting materials and controlled reaction environments result in this compound with low halide impurities (<50 ppm chloride when adapted from oxide preparation methods).
Analyse Chemischer Reaktionen
Types of Reactions: Dibutyltin dibromide undergoes various chemical reactions, including:
Substitution Reactions: It can react with nucleophiles to replace the bromide ions.
Catalytic Reactions: It is used as a catalyst in the trimethylsilylcyanation of aldehydes and imines.
Common Reagents and Conditions:
Trimethylsilyl Cyanide: Used in the presence of this compound to catalyze the addition to aldehydes and imines.
Solvents: Common solvents include toluene and other non-polar solvents.
Major Products:
Trimethylsilylated Cyanohydrins: Formed from the reaction with aldehydes.
α-Aminonitriles: Formed from the reaction with aldimines.
Wissenschaftliche Forschungsanwendungen
Dibutyltin dibromide is an organotin compound with the chemical formula . It appears as a colorless to pale yellow liquid at room temperature. This compound is known for its use in organic synthesis and as a catalyst in various chemical reactions.
Scientific Research Applications
This compound has diverse applications in scientific research, including use as a catalyst in organic synthesis, in material science, and in biological research.
Organic Synthesis
This compound serves as a catalyst in various organic reactions, such as the trimethylsilylcyanation of aldehydes and imines. It efficiently catalyzes the addition of trimethylsilyl cyanide to aldehydes and aldimines. The uniqueness of this compound lies in its specific reactivity with bromide ions, influencing its catalytic properties and reactivity in organic synthesis.
Material Science
In material science, this compound is used in the synthesis of organotin polymers and other materials. Epoxy composites containing resin modified with this compound are characterized by increased fungitoxicity and fire resistance .
Biological Research
Dibutyltin compounds have been studied for their potential biological activities, including antitubercular properties. Dibutyltin compounds, including this compound, have been shown to have effects on various types of cells, influencing cell function, cell signaling pathways, gene expression, and cellular metabolism.
Industrial Applications
Beyond scientific research, this compound finds use in the production of polyvinyl chloride (PVC) stabilizers and other industrial chemicals.
Health Effects
Acute-duration studies with dibutyltins have described lethal doses in rats between 20 and 50 mg/kg administered by gavage .
Systemic Effects
No studies were located regarding musculoskeletal or ocular effects in humans or animals after oral exposure to inorganic tin or organotin compounds .
Respiratory Effects
No histopathological alterations were observed in the lungs, bronchi, and trachea from rats and mice fed diets that provided up to 6.7 and 19.8 mg/kg/day of dibutyltin diacetate, respectively, for 78 weeks .
Cardiovascular Effects
No gross or microscopic alterations were observed in the heart of rats dosed with up to 5.7 mg dibutyltin dichloride/kg/day for 90 days .
Gastrointestinal Effects
Wirkmechanismus
The mechanism of action of dibutyltin dibromide involves its ability to coordinate with various substrates and catalyze reactions. In the case of the trimethylsilylcyanation reaction, this compound activates the aldehyde or imine substrate, facilitating the addition of trimethylsilyl cyanide . The molecular targets and pathways involved in its biological activities, such as antitubercular action, are still under investigation .
Vergleich Mit ähnlichen Verbindungen
Structural and Functional Differences
Dibutyltin compounds share a common tin core with butyl ligands but differ in their anionic substituents (Table 1). These structural variations influence their solubility, bioavailability, and interactions with biological targets.
Table 1: Structural Comparison of Dibutyltin Compounds
Toxicity Profiles
Dibutyltin compounds exhibit varying toxicities based on their substituents:
- Maximum Non-Toxic Concentrations: DBTL (10 µM) is less toxic than DBTC (1 µM) and DBTM (0.1 µM) in cell-based assays.
PPARγ and RXRα Agonism
- PPARγ Activation : DBTC exhibits the strongest PPARγ activation (7.5-fold), followed by DBTA (2.25-fold) and DBTM (2.61-fold).
- RXRα Activation : DBTC and DBTL act as partial RXRα agonists, with 1.99- and 2.49-fold activation, respectively.
- Mechanism : All dibutyltins bind PPARγ via ionic interactions between tin and Cys285; mutating this residue abolishes activity.
Adipogenic Effects
DBTL is the most potent adipogen, increasing lipid accumulation in 3T3-L1 cells more effectively than DBTC or DBTM. Tributyltin chloride (TBTC), a tri-substituted organotin, outperforms all dibutyltins in adipogenesis but has higher toxicity.
Regulatory and Research Status
- DBTC: Best-studied dibutyltin compound, with confirmed roles in adipogenesis, immunotoxicity, and PPARγ/RXRα pathways.
- DBTL : Subject of reclassification proposals due to disputed toxicity data.
- DBTB: Not directly addressed in the provided literature, but its bromine substituents may alter reactivity compared to chloride analogs.
Biologische Aktivität
Dibutyltin dibromide (DBT) is an organotin compound that has garnered attention for its diverse biological activities, particularly in the fields of toxicology and pharmacology. This article delves into the biological effects, mechanisms of action, and relevant case studies associated with DBT, supported by data tables and research findings.
Chemical Structure and Properties
This compound is characterized by its chemical formula . It is a member of the butyltin family, which has been widely used in various industrial applications, including as a biocide and stabilizer in plastics. Its structure allows for interactions with biological macromolecules, leading to significant biological effects.
Mechanisms of Biological Activity
The biological activity of this compound is primarily attributed to its ability to interact with cellular components, including proteins and nucleic acids. Notably, DBT has been shown to bind to sulfhydryl groups in proteins, leading to oxidative stress and subsequent cellular damage. This mechanism underlies its cytotoxic effects and potential for inducing apoptosis in various cell types.
1. Cytotoxicity and Apoptosis
DBT has demonstrated significant cytotoxic effects across different cell lines. A study highlighted that DBT induces apoptosis in PC12 cells through a mitochondria-dependent pathway, characterized by increased reactive oxygen species (ROS) production and mitochondrial membrane potential disruption . The following table summarizes key findings on cytotoxicity:
Cell Line | IC50 (µM) | Mechanism of Action |
---|---|---|
PC12 | 15 | Mitochondrial dysfunction, ROS generation |
HCT-116 | 25 | Induction of apoptosis |
MCF-7 | 30 | Cell cycle arrest at G2/M phase |
2. Antiproliferative Activity
Research indicates that DBT exhibits antiproliferative properties against various cancer cell lines. The compound's ability to inhibit cell growth is linked to its interaction with cellular signaling pathways involved in proliferation. For instance, studies have reported that DBT can significantly reduce the viability of cancer cells such as MCF-7 (breast cancer) and A-549 (lung cancer) by inducing apoptosis and blocking the cell cycle .
3. Neurotoxicity
This compound has been classified as a neurotoxicant. In animal models, exposure to DBT has resulted in neurobehavioral deficits and alterations in neurotransmitter levels. The compound's neurotoxic effects are believed to stem from its capacity to induce oxidative stress, leading to neuronal damage .
Case Study 1: Reproductive Toxicity
A significant study evaluated the reproductive toxicity of organotin compounds, including this compound, in rats. Results indicated that exposure led to reduced pregnancy rates and uterine atrophy after prolonged exposure periods . This underscores the potential risks associated with DBT exposure in reproductive health.
Case Study 2: Environmental Impact
The environmental persistence of dibutyltin compounds has raised concerns regarding their ecological impact. Studies have shown that DBT can accumulate in aquatic organisms, leading to bioaccumulation and potential toxicity within food chains . Monitoring programs have documented elevated levels of organotin compounds in sediments from industrialized regions.
Q & A
Basic Research Questions
Q. What are the standard protocols for synthesizing dibutyltin dibromide, and how can experimental reproducibility be ensured?
Synthesizing this compound typically involves reacting dibutyltin oxide with hydrobromic acid under controlled stoichiometric and temperature conditions. To ensure reproducibility:
- Document reaction parameters (e.g., molar ratios, reflux duration, solvent purity).
- Purify products via recrystallization or vacuum distillation, and verify purity using techniques like melting point analysis or gas chromatography .
- Provide detailed procedural steps in the Experimental section of manuscripts, including equipment specifications (e.g., reflux apparatus) and safety protocols for handling bromine derivatives .
Q. Which spectroscopic techniques are most effective for characterizing this compound, and what key spectral markers should researchers prioritize?
Key techniques include:
- NMR Spectroscopy : Focus on NMR for tin-centered environments (e.g., chemical shifts between δ 0–200 ppm) and / NMR for alkyl group assignments .
- FT-IR Spectroscopy : Identify Sn-Br stretching vibrations (~200–250 cm) and Sn-C bonds (~500–600 cm) .
- Elemental Analysis : Confirm Br and Sn content within ±0.3% of theoretical values . Cross-reference data with established databases (e.g., NIST Chemistry WebBook) to validate structural assignments .
Q. What are the primary degradation pathways of this compound in aqueous environments, and which analytical methods are optimal for tracking these transformations?
Degradation pathways include hydrolysis (yielding dibutyltin hydroxide) and photolytic cleavage. To monitor these:
- Use HPLC-MS to detect hydrolysis byproducts and quantify degradation rates under varying pH/temperature conditions.
- Employ ICP-OES or XANES to track tin speciation in environmental matrices .
- Design controlled kinetic studies with spiked samples to isolate degradation mechanisms from abiotic factors .
Advanced Research Questions
Q. How can researchers resolve contradictions in reported toxicity data of this compound across different biological models?
Contradictions often arise from variations in exposure protocols, model organisms, or endpoint measurements. Methodological solutions include:
- Conducting meta-analyses to harmonize datasets, adjusting for variables like dose-response thresholds and bioaccumulation factors.
- Applying toxicogenomic profiling to identify conserved molecular pathways (e.g., oxidative stress markers) across models .
- Using Bayesian statistical frameworks to quantify uncertainty and model inter-study variability .
Q. What computational modeling approaches are suitable for predicting the environmental persistence of this compound, and how can their parameters be validated experimentally?
- Quantum Mechanical Methods : Density Functional Theory (DFT) to calculate bond dissociation energies (BDEs) for Sn-Br bonds, predicting hydrolysis rates .
- QSAR Models : Train models using experimental log and soil sorption data to estimate bioaccumulation potential .
- Validation : Compare computational predictions with field data (e.g., half-life in sediment cores) and lab-based microcosm studies .
Q. How can kinetic studies be designed to elucidate the catalytic behavior of this compound in esterification reactions under varying solvent conditions?
- Experimental Design :
- Use a stopped-flow apparatus to monitor reaction rates in real-time across solvents (e.g., polar aprotic vs. nonpolar).
- Vylate temperature and catalyst loading to construct Arrhenius plots and identify rate-limiting steps .
- Data Analysis :
- Apply the Eyring-Polanyi equation to correlate solvent dielectric constants with activation energies.
- Validate mechanistic hypotheses using isotopic labeling (e.g., in ester products) .
Q. Methodological Considerations
- Literature Review : Prioritize primary sources (e.g., Journal of Organometallic Chemistry) over reviews to avoid biased interpretations .
- Data Reproducibility : Archive raw spectral data and computational input files in repositories like Zenodo for peer validation .
- Ethical Compliance : Adhere to OECD guidelines for ecotoxicity testing to ensure data comparability across studies .
Eigenschaften
IUPAC Name |
dibromo(dibutyl)stannane | |
---|---|---|
Source | PubChem | |
URL | https://pubchem.ncbi.nlm.nih.gov | |
Description | Data deposited in or computed by PubChem | |
InChI |
InChI=1S/2C4H9.2BrH.Sn/c2*1-3-4-2;;;/h2*1,3-4H2,2H3;2*1H;/q;;;;+2/p-2 | |
Source | PubChem | |
URL | https://pubchem.ncbi.nlm.nih.gov | |
Description | Data deposited in or computed by PubChem | |
InChI Key |
QSHZUFRQHSINTB-UHFFFAOYSA-L | |
Source | PubChem | |
URL | https://pubchem.ncbi.nlm.nih.gov | |
Description | Data deposited in or computed by PubChem | |
Canonical SMILES |
CCCC[Sn](CCCC)(Br)Br | |
Source | PubChem | |
URL | https://pubchem.ncbi.nlm.nih.gov | |
Description | Data deposited in or computed by PubChem | |
Molecular Formula |
C8H18Br2Sn | |
Source | PubChem | |
URL | https://pubchem.ncbi.nlm.nih.gov | |
Description | Data deposited in or computed by PubChem | |
DSSTOX Substance ID |
DTXSID90244153 | |
Record name | Stannane, dibromodibutyl- | |
Source | EPA DSSTox | |
URL | https://comptox.epa.gov/dashboard/DTXSID90244153 | |
Description | DSSTox provides a high quality public chemistry resource for supporting improved predictive toxicology. | |
Molecular Weight |
392.75 g/mol | |
Source | PubChem | |
URL | https://pubchem.ncbi.nlm.nih.gov | |
Description | Data deposited in or computed by PubChem | |
CAS No. |
996-08-7 | |
Record name | Dibutyltin dibromide | |
Source | CAS Common Chemistry | |
URL | https://commonchemistry.cas.org/detail?cas_rn=996-08-7 | |
Description | CAS Common Chemistry is an open community resource for accessing chemical information. Nearly 500,000 chemical substances from CAS REGISTRY cover areas of community interest, including common and frequently regulated chemicals, and those relevant to high school and undergraduate chemistry classes. This chemical information, curated by our expert scientists, is provided in alignment with our mission as a division of the American Chemical Society. | |
Explanation | The data from CAS Common Chemistry is provided under a CC-BY-NC 4.0 license, unless otherwise stated. | |
Record name | Stannane, dibromodibutyl- | |
Source | ChemIDplus | |
URL | https://pubchem.ncbi.nlm.nih.gov/substance/?source=chemidplus&sourceid=0000996087 | |
Description | ChemIDplus is a free, web search system that provides access to the structure and nomenclature authority files used for the identification of chemical substances cited in National Library of Medicine (NLM) databases, including the TOXNET system. | |
Record name | Stannane, dibromodibutyl- | |
Source | EPA DSSTox | |
URL | https://comptox.epa.gov/dashboard/DTXSID90244153 | |
Description | DSSTox provides a high quality public chemistry resource for supporting improved predictive toxicology. | |
Record name | dibromodibutylstannane | |
Source | European Chemicals Agency (ECHA) | |
URL | https://echa.europa.eu/information-on-chemicals | |
Description | The European Chemicals Agency (ECHA) is an agency of the European Union which is the driving force among regulatory authorities in implementing the EU's groundbreaking chemicals legislation for the benefit of human health and the environment as well as for innovation and competitiveness. | |
Explanation | Use of the information, documents and data from the ECHA website is subject to the terms and conditions of this Legal Notice, and subject to other binding limitations provided for under applicable law, the information, documents and data made available on the ECHA website may be reproduced, distributed and/or used, totally or in part, for non-commercial purposes provided that ECHA is acknowledged as the source: "Source: European Chemicals Agency, http://echa.europa.eu/". Such acknowledgement must be included in each copy of the material. ECHA permits and encourages organisations and individuals to create links to the ECHA website under the following cumulative conditions: Links can only be made to webpages that provide a link to the Legal Notice page. | |
Retrosynthesis Analysis
AI-Powered Synthesis Planning: Our tool employs the Template_relevance Pistachio, Template_relevance Bkms_metabolic, Template_relevance Pistachio_ringbreaker, Template_relevance Reaxys, Template_relevance Reaxys_biocatalysis model, leveraging a vast database of chemical reactions to predict feasible synthetic routes.
One-Step Synthesis Focus: Specifically designed for one-step synthesis, it provides concise and direct routes for your target compounds, streamlining the synthesis process.
Accurate Predictions: Utilizing the extensive PISTACHIO, BKMS_METABOLIC, PISTACHIO_RINGBREAKER, REAXYS, REAXYS_BIOCATALYSIS database, our tool offers high-accuracy predictions, reflecting the latest in chemical research and data.
Strategy Settings
Precursor scoring | Relevance Heuristic |
---|---|
Min. plausibility | 0.01 |
Model | Template_relevance |
Template Set | Pistachio/Bkms_metabolic/Pistachio_ringbreaker/Reaxys/Reaxys_biocatalysis |
Top-N result to add to graph | 6 |
Feasible Synthetic Routes
Haftungsausschluss und Informationen zu In-Vitro-Forschungsprodukten
Bitte beachten Sie, dass alle Artikel und Produktinformationen, die auf BenchChem präsentiert werden, ausschließlich zu Informationszwecken bestimmt sind. Die auf BenchChem zum Kauf angebotenen Produkte sind speziell für In-vitro-Studien konzipiert, die außerhalb lebender Organismen durchgeführt werden. In-vitro-Studien, abgeleitet von dem lateinischen Begriff "in Glas", beinhalten Experimente, die in kontrollierten Laborumgebungen unter Verwendung von Zellen oder Geweben durchgeführt werden. Es ist wichtig zu beachten, dass diese Produkte nicht als Arzneimittel oder Medikamente eingestuft sind und keine Zulassung der FDA für die Vorbeugung, Behandlung oder Heilung von medizinischen Zuständen, Beschwerden oder Krankheiten erhalten haben. Wir müssen betonen, dass jede Form der körperlichen Einführung dieser Produkte in Menschen oder Tiere gesetzlich strikt untersagt ist. Es ist unerlässlich, sich an diese Richtlinien zu halten, um die Einhaltung rechtlicher und ethischer Standards in Forschung und Experiment zu gewährleisten.