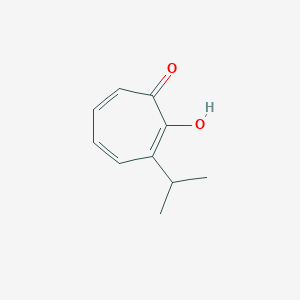
beta-Thujaplicine
- Klicken Sie auf QUICK INQUIRY, um ein Angebot von unserem Expertenteam zu erhalten.
- Mit qualitativ hochwertigen Produkten zu einem WETTBEWERBSFÄHIGEN Preis können Sie sich mehr auf Ihre Forschung konzentrieren.
Übersicht
Beschreibung
Vorbereitungsmethoden
Synthesewege und Reaktionsbedingungen: : Alpha-Thujaplicin kann durch verschiedene Verfahren synthetisiert werden. Ein gängiger Syntheseweg beinhaltet die Cyclisierung eines geeigneten Vorläufers, wie z. B. 2-Hydroxy-3-Isopropyl-2,4,6-Cycloheptatrien-1-on . Die Reaktion erfordert typischerweise spezielle Bedingungen, darunter die Verwendung von Katalysatoren und kontrollierte Temperaturen, um die Bildung der gewünschten Tropolonstruktur zu gewährleisten .
Industrielle Produktionsmethoden: : Die industrielle Produktion von Alpha-Thujaplicin beinhaltet häufig die Extraktion der Verbindung aus dem Kernholz von Bäumen der Familie Cupressaceae. Der Extraktionsprozess umfasst die Lösungsmittelextraktion, gefolgt von Reinigungsschritten wie Kristallisation oder Chromatographie, um die reine Verbindung zu isolieren .
Analyse Chemischer Reaktionen
Arten von Reaktionen: : Alpha-Thujaplicin durchläuft verschiedene chemische Reaktionen, darunter:
Oxidation: Alpha-Thujaplicin kann oxidiert werden, um entsprechende Chinone oder andere oxidierte Derivate zu bilden.
Substitution: Alpha-Thujaplicin kann Substitutionsreaktionen eingehen, bei denen funktionelle Gruppen durch andere Gruppen ersetzt werden.
Häufige Reagenzien und Bedingungen
Oxidation: Häufige Oxidationsmittel sind Kaliumpermanganat (KMnO4) und Chromtrioxid (CrO3).
Reduktion: Reduktionsmittel wie Natriumborhydrid (NaBH4) und Lithiumaluminiumhydrid (LiAlH4) werden häufig verwendet.
Substitution: Verschiedene Nucleophile und Elektrophile können in Substitutionsreaktionen verwendet werden, abhängig vom gewünschten Produkt.
Hauptprodukte
Oxidation: Bildung von Chinonen und anderen oxidierten Derivaten.
Reduktion: Bildung von Dihydroderivaten.
Substitution: Bildung von substituierten Tropolonderivaten.
Wissenschaftliche Forschungsanwendungen
Alpha-Thujaplicin hat eine breite Palette von Anwendungen in der wissenschaftlichen Forschung, darunter:
Industrie: Wird bei der Entwicklung von antimikrobiellen Mitteln für den Holzschutz und andere Anwendungen eingesetzt.
Wirkmechanismus
Alpha-Thujaplicin entfaltet seine Wirkung durch verschiedene Mechanismen:
Antibakterielle und antifungale Aktivität: Alpha-Thujaplicin stört die Integrität der Zellmembran von Bakterien und Pilzen, was zum Zelltod führt.
Antitumor-Aktivität: Alpha-Thujaplicin induziert Apoptose (programmierter Zelltod) in Krebszellen, indem es bestimmte Signalwege aktiviert und die Zellproliferation hemmt.
Metallchelatisierung: Alpha-Thujaplicin hat eine starke Metallchelatisierungseigenschaft, die die Aktivität von metall-abhängigen Enzymen hemmen und zelluläre Prozesse stören kann.
Wirkmechanismus
Alpha-thujaplicin exerts its effects through several mechanisms:
Antibacterial and Antifungal Activity: Alpha-thujaplicin disrupts the cell membrane integrity of bacteria and fungi, leading to cell death.
Anticancer Activity: Alpha-thujaplicin induces apoptosis (programmed cell death) in cancer cells by activating specific signaling pathways and inhibiting cell proliferation.
Metal Chelation: Alpha-thujaplicin has a strong metal-chelating capacity, which can inhibit the activity of metal-dependent enzymes and disrupt cellular processes.
Vergleich Mit ähnlichen Verbindungen
Alpha-Thujaplicin gehört zu einer Gruppe isomeren Tropolonderivaten, darunter:
Beta-Thujaplicin (Hinokitiol): Bekannt für seine starken antibakteriellen und antifungalen Eigenschaften.
Gamma-Thujaplicin: Zeigt ähnliche biologische Aktivitäten, aber mit unterschiedlicher Potenz.
Einzigartigkeit: : Alpha-Thujaplicin ist einzigartig aufgrund seiner spezifischen Isopropylgruppenpositionierung, die seine chemische Reaktivität und biologische Aktivität beeinflusst . Im Vergleich zu Beta-Thujaplicin und Gamma-Thujaplicin weist Alpha-Thujaplicin unterschiedliche Eigenschaften auf, die es für bestimmte Anwendungen in Forschung und Industrie geeignet machen .
Eigenschaften
CAS-Nummer |
1946-74-3 |
---|---|
Molekularformel |
C10H12O2 |
Molekulargewicht |
164.20 g/mol |
IUPAC-Name |
2-hydroxy-3-propan-2-ylcyclohepta-2,4,6-trien-1-one |
InChI |
InChI=1S/C10H12O2/c1-7(2)8-5-3-4-6-9(11)10(8)12/h3-7H,1-2H3,(H,11,12) |
InChI-Schlüssel |
TUFYVOCKVJOUIR-UHFFFAOYSA-N |
SMILES |
CC(C)C1=C(C(=O)C=CC=C1)O |
Kanonische SMILES |
CC(C)C1=C(C(=O)C=CC=C1)O |
Key on ui other cas no. |
1946-74-3 |
Herkunft des Produkts |
United States |
Retrosynthesis Analysis
AI-Powered Synthesis Planning: Our tool employs the Template_relevance Pistachio, Template_relevance Bkms_metabolic, Template_relevance Pistachio_ringbreaker, Template_relevance Reaxys, Template_relevance Reaxys_biocatalysis model, leveraging a vast database of chemical reactions to predict feasible synthetic routes.
One-Step Synthesis Focus: Specifically designed for one-step synthesis, it provides concise and direct routes for your target compounds, streamlining the synthesis process.
Accurate Predictions: Utilizing the extensive PISTACHIO, BKMS_METABOLIC, PISTACHIO_RINGBREAKER, REAXYS, REAXYS_BIOCATALYSIS database, our tool offers high-accuracy predictions, reflecting the latest in chemical research and data.
Strategy Settings
Precursor scoring | Relevance Heuristic |
---|---|
Min. plausibility | 0.01 |
Model | Template_relevance |
Template Set | Pistachio/Bkms_metabolic/Pistachio_ringbreaker/Reaxys/Reaxys_biocatalysis |
Top-N result to add to graph | 6 |
Feasible Synthetic Routes
Haftungsausschluss und Informationen zu In-Vitro-Forschungsprodukten
Bitte beachten Sie, dass alle Artikel und Produktinformationen, die auf BenchChem präsentiert werden, ausschließlich zu Informationszwecken bestimmt sind. Die auf BenchChem zum Kauf angebotenen Produkte sind speziell für In-vitro-Studien konzipiert, die außerhalb lebender Organismen durchgeführt werden. In-vitro-Studien, abgeleitet von dem lateinischen Begriff "in Glas", beinhalten Experimente, die in kontrollierten Laborumgebungen unter Verwendung von Zellen oder Geweben durchgeführt werden. Es ist wichtig zu beachten, dass diese Produkte nicht als Arzneimittel oder Medikamente eingestuft sind und keine Zulassung der FDA für die Vorbeugung, Behandlung oder Heilung von medizinischen Zuständen, Beschwerden oder Krankheiten erhalten haben. Wir müssen betonen, dass jede Form der körperlichen Einführung dieser Produkte in Menschen oder Tiere gesetzlich strikt untersagt ist. Es ist unerlässlich, sich an diese Richtlinien zu halten, um die Einhaltung rechtlicher und ethischer Standards in Forschung und Experiment zu gewährleisten.