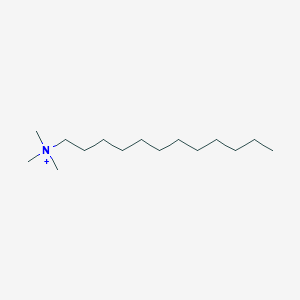
Dodecyltrimethylammonium
Übersicht
Beschreibung
Dodecyltrimethylammonium ist eine quaternäre Ammoniumverbindung mit der chemischen Formel C₁₅H₃₄N. Es ist ein kationisches Tensid, das in verschiedenen Anwendungen weit verbreitet ist, da es hydrophobe Wechselwirkungen und elektrostatische Kräfte stören kann. Diese Verbindung ist bekannt für ihre Wirksamkeit bei der Solubilisierung von Proteinen und Peptiden, der Extraktion von DNA und der Lyse von Zellen .
Vorbereitungsmethoden
Dodecyltrimethylammonium kann durch die Reaktion von Dodecyl bromid mit Trimethylamin synthetisiert werden. Die Reaktion erfolgt typischerweise in einem organischen Lösungsmittel wie Ethanol oder Methanol unter Rückflussbedingungen. Das Produkt, this compound bromid, wird dann durch Umkristallisation gereinigt . Industrielle Produktionsmethoden umfassen oft ähnliche Synthesewege, jedoch in größerem Maßstab, mit zusätzlichen Reinigungsschritten, um eine hohe Reinheit zu gewährleisten .
Analyse Chemischer Reaktionen
Dodecyltrimethylammonium durchläuft verschiedene chemische Reaktionen, einschließlich Ionenaustauschreaktionen, bei denen es andere Kationen in einem Substrat ersetzen kann. Es beteiligt sich auch an Micellisationsvorgängen, wobei es Micellen in wässrigen Lösungen bildet. Häufige Reagenzien, die in diesen Reaktionen verwendet werden, umfassen Natriumdodecylsulfat und andere Tenside . Die wichtigsten Produkte, die aus diesen Reaktionen gebildet werden, hängen von den jeweiligen Bedingungen und den verwendeten Reagenzien ab .
Wissenschaftliche Forschungsanwendungen
Dodecyltrimethylammonium hat ein breites Spektrum an Anwendungen in der wissenschaftlichen Forschung:
Chemie: Es wird als Tensid bei der Herstellung von Gold-Nanopartikeln und anderen Nanomaterialien verwendet.
Biologie: Es wird bei Zelllyseprozessen eingesetzt, um intrazelluläre Komponenten für die Analyse freizusetzen.
Industrie: Es wird als Emulgator in Gummi und Asphalt sowie als Antistatikmittel in synthetischen Fasern verwendet.
Wirkmechanismus
Der Wirkmechanismus von this compound beruht auf seiner Fähigkeit, hydrophobe Wechselwirkungen und elektrostatische Kräfte zu stören. Diese Störung ermöglicht es, membrangebundene Proteine und Peptide zu solubilisieren, wodurch sie für die Analyse und Charakterisierung zugänglich werden . Es interagiert auch mit DNA, verändert ihre Konformation und erleichtert ihre Freisetzung aus zellulären Komponenten . Die kationische Natur der Verbindung ermöglicht es ihr, mit negativ geladenen Zellmembranen zu interagieren, was zu Zelllyse und der Freisetzung intrazellulärer Inhalte führt .
Wirkmechanismus
The mechanism of action of dodecyltrimethylammonium involves its ability to disrupt hydrophobic interactions and electrostatic forces. This disruption allows it to solubilize membrane-bound proteins and peptides, making them accessible for analysis and characterization . It also interacts with DNA, altering its conformation and facilitating its release from cellular components . The compound’s cationic nature enables it to interact with negatively charged cell membranes, leading to cell lysis and the release of intracellular contents .
Vergleich Mit ähnlichen Verbindungen
Dodecyltrimethylammonium ähnelt anderen quaternären Ammoniumverbindungen wie Hexadecyltrimethylammonium und Didecyldimethylammonium. Es zeichnet sich durch ein ausgewogenes Verhältnis von hydrophoben und hydrophilen Eigenschaften aus, wodurch es besonders effektiv bei der Solubilisierung einer breiten Palette von Biomolekülen ist . Ähnliche Verbindungen umfassen:
Hexadecyltrimethylammonium: Bekannt für seinen Einsatz bei der Synthese von mesoporösen Siliciumdioxidmaterialien.
Didecyldimethylammonium: Wird in Desinfektionsmitteln und Antiseptika verwendet.
Die Vielseitigkeit und Wirksamkeit von this compound in verschiedenen Anwendungen machen es zu einer wertvollen Verbindung in der wissenschaftlichen Forschung und in industriellen Prozessen.
Eigenschaften
CAS-Nummer |
10182-91-9 |
---|---|
Molekularformel |
C15H34N+ |
Molekulargewicht |
228.44 g/mol |
IUPAC-Name |
dodecyl(trimethyl)azanium |
InChI |
InChI=1S/C15H34N/c1-5-6-7-8-9-10-11-12-13-14-15-16(2,3)4/h5-15H2,1-4H3/q+1 |
InChI-Schlüssel |
VICYBMUVWHJEFT-UHFFFAOYSA-N |
SMILES |
CCCCCCCCCCCC[N+](C)(C)C |
Kanonische SMILES |
CCCCCCCCCCCC[N+](C)(C)C |
10182-91-9 | |
Verwandte CAS-Nummern |
1119-94-4 (bromide) 112-00-5 (chloride) 13623-06-8 (methyl sulfate salt) |
Synonyme |
1-dodecanaminium, N,N,N-trimethyl-, bromide ammonium, dodecyltrimethyl-, chloride ammonium, dodecyltrimethyl-,bromide C(12)TAC C12QAC C12TAC dodecyl trimethyl ammonium chloride dodecyl trimethylammonium chloride dodecyltrimethylammonium dodecyltrimethylammonium bromide dodecyltrimethylammonium chloride dodecyltrimethylammonium chromate (2:1) dodecyltrimethylammonium ethyl sulfate dodecyltrimethylammonium hydroxide dodecyltrimethylammonium iodide dodecyltrimethylammonium maleate (2:1) dodecyltrimethylammonium methyl sulfate salt dodecyltrimethylammonium perchlorate dodecyltrimethylammonium sulfate (2:1) dodecyltrimethylammonium sulfide (2:1) dodecyltrimethylammonium thiomolybdate salt hydrate DoTAC compound DTMACl cpd laurtrimonium laurtrimonium bromide laurtrimonium chloride lauryl trimethyl ammonium chloride lauryltrimethylammonium bromide lauryltrimethylammonium chloride monolauryltrimethylammonium chloride N,N,N-trimethyl-1-dodecanaminium chloride n-dodecyl triethylammonium bromide n-dodecyltrimethylammonium n-dodecyltrimethylammonium chloride |
Herkunft des Produkts |
United States |
Retrosynthesis Analysis
AI-Powered Synthesis Planning: Our tool employs the Template_relevance Pistachio, Template_relevance Bkms_metabolic, Template_relevance Pistachio_ringbreaker, Template_relevance Reaxys, Template_relevance Reaxys_biocatalysis model, leveraging a vast database of chemical reactions to predict feasible synthetic routes.
One-Step Synthesis Focus: Specifically designed for one-step synthesis, it provides concise and direct routes for your target compounds, streamlining the synthesis process.
Accurate Predictions: Utilizing the extensive PISTACHIO, BKMS_METABOLIC, PISTACHIO_RINGBREAKER, REAXYS, REAXYS_BIOCATALYSIS database, our tool offers high-accuracy predictions, reflecting the latest in chemical research and data.
Strategy Settings
Precursor scoring | Relevance Heuristic |
---|---|
Min. plausibility | 0.01 |
Model | Template_relevance |
Template Set | Pistachio/Bkms_metabolic/Pistachio_ringbreaker/Reaxys/Reaxys_biocatalysis |
Top-N result to add to graph | 6 |
Feasible Synthetic Routes
Haftungsausschluss und Informationen zu In-Vitro-Forschungsprodukten
Bitte beachten Sie, dass alle Artikel und Produktinformationen, die auf BenchChem präsentiert werden, ausschließlich zu Informationszwecken bestimmt sind. Die auf BenchChem zum Kauf angebotenen Produkte sind speziell für In-vitro-Studien konzipiert, die außerhalb lebender Organismen durchgeführt werden. In-vitro-Studien, abgeleitet von dem lateinischen Begriff "in Glas", beinhalten Experimente, die in kontrollierten Laborumgebungen unter Verwendung von Zellen oder Geweben durchgeführt werden. Es ist wichtig zu beachten, dass diese Produkte nicht als Arzneimittel oder Medikamente eingestuft sind und keine Zulassung der FDA für die Vorbeugung, Behandlung oder Heilung von medizinischen Zuständen, Beschwerden oder Krankheiten erhalten haben. Wir müssen betonen, dass jede Form der körperlichen Einführung dieser Produkte in Menschen oder Tiere gesetzlich strikt untersagt ist. Es ist unerlässlich, sich an diese Richtlinien zu halten, um die Einhaltung rechtlicher und ethischer Standards in Forschung und Experiment zu gewährleisten.