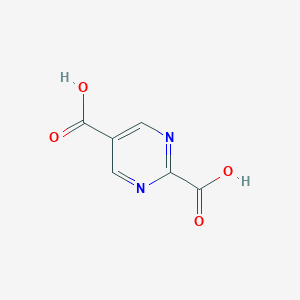
Pyrimidine-2,5-dicarboxylic acid
Übersicht
Beschreibung
Pyrimidine-2,5-dicarboxylic acid (PDA, C₆H₄N₂O₄) is a heterocyclic dicarboxylic acid featuring a pyrimidine ring substituted with carboxyl groups at the 2- and 5-positions. Its crystal structure (orthorhombic, space group C222₁) reveals a planar pyrimidine ring and a three-dimensional hydrogen-bonded network formed via O–H⋯O, O–H⋯N, and C–H⋯O interactions .
Vorbereitungsmethoden
Hydrolysis of Cyano Precursors
A widely documented approach involves the hydrolysis of cyano-substituted pyrimidine intermediates. The Chinese patent CN103880757A outlines a two-step synthesis for 5-hydroxy pyrimidine-2-carboxylic acid, which can be adapted for dicarboxylic acid derivatives .
Step 1: Alkoxylation of 5-Bromo-2-cyanopyrimidine
In a toluene solvent, 5-bromo-2-cyanopyrimidine reacts with phenylcarbinol (benzyl alcohol) in the presence of cesium carbonate (Cs₂CO₃), cuprous iodide (CuI), and 1,10-phenanthroline. The reaction proceeds at 80–110°C for 4–12 hours, yielding 5-benzyloxy-2-cyanopyrimidine with a 90% yield. Key parameters include:
-
Molar ratio : 1:1.5–3.5 (5-bromo-2-cyanopyrimidine to phenylcarbinol)
-
Catalyst loading : ≥10% CuI and ≥20% 1,10-phenanthroline relative to the substrate .
Step 2: Alkaline Hydrolysis and Acidification
The 5-benzyloxy-2-cyanopyrimidine is hydrolyzed in aqueous potassium hydroxide (KOH) at 25–100°C. After cooling, hydrochloric acid (HCl) adjusts the pH to 3–4, precipitating the product. This step achieves a 67% yield. Modifications to introduce a second carboxylic acid group would require additional nitrile precursors or oxidation steps .
Coordination Polymer Precursor Breakdown
A study in New Journal of Chemistry (2023) highlights the use of pyrimidine-2,5-dicarboxylic acid as a ligand in copper coordination polymers . While the focus is on nanoparticle synthesis, the ligand preparation involves:
Solvent-Based Recrystallization
This compound is dissolved in ethanol and combined with cobalt chloride (CoCl₂) to form coordination complexes. Pure acid is obtained via slow evaporation, yielding crystals suitable for structural analysis .
Comparative Analysis of Methods
Industrial Considerations
Catalyst Optimization
The use of CuI and 1,10-phenanthroline in CN103880757A reduces reaction times and improves yields compared to traditional Ullmann-type couplings . Scaling this method would require efficient catalyst recovery systems.
Environmental Impact
KMnO₄-based oxidation generates MnO₂ waste, necessitating filtration and neutralization steps . Green chemistry alternatives, such as enzymatic or electrochemical oxidation, remain underexplored for this compound.
Analyse Chemischer Reaktionen
Types of Reactions: Pyrimidine-2,5-dicarboxylic acid undergoes various chemical reactions, including:
Oxidation: The compound can be oxidized to form pyrimidine-2,5-dicarboxylate.
Reduction: Reduction reactions can convert it into pyrimidine-2,5-dicarboxamide.
Substitution: It can undergo substitution reactions where functional groups are replaced by other groups.
Common Reagents and Conditions:
Oxidation: Potassium permanganate, chromium trioxide.
Reduction: Hydrogen gas in the presence of a catalyst such as palladium on carbon.
Substitution: Various nucleophiles under acidic or basic conditions.
Major Products:
Oxidation: Pyrimidine-2,5-dicarboxylate.
Reduction: Pyrimidine-2,5-dicarboxamide.
Substitution: Various substituted pyrimidine derivatives.
Wissenschaftliche Forschungsanwendungen
Medicinal Chemistry
PDA serves as an important building block for the synthesis of various pharmaceutical compounds. Its structural features allow it to act as a potential ligand in drug design.
- Antimicrobial Activity : Research has shown that derivatives of PDA exhibit antimicrobial properties. For instance, certain PDA derivatives have been synthesized and tested against various bacterial strains, showing promising results in inhibiting growth .
- Anticancer Properties : PDA and its derivatives have been investigated for their potential anticancer activities. Studies indicate that compounds derived from PDA can induce apoptosis in cancer cells through various mechanisms, including the modulation of cell signaling pathways .
The unique properties of PDA make it suitable for various applications in material science:
- Polymer Chemistry : PDA is used as a monomer in the synthesis of polyesters and polyamides. Its dicarboxylic acid functionality allows for the formation of high-performance polymers with enhanced thermal and mechanical properties.
- Nanomaterials : Research indicates that PDA can be incorporated into nanostructures for applications in electronics and photonics. The integration of PDA into nanomaterials has shown improvements in conductivity and light absorption characteristics .
Case Study 1: Synthesis of Metal Complexes
A study focused on synthesizing cobalt complexes using PDA as a ligand. The resulting complexes exhibited significant catalytic activity in oxidation reactions, showcasing the potential of PDA-based metal complexes in catalysis .
Case Study 2: Antimicrobial Testing
In another research project, various derivatives of PDA were synthesized and tested against E. coli and Staphylococcus aureus. The results indicated that specific modifications to the PDA structure enhanced its antimicrobial efficacy significantly compared to unmodified PDA .
Wirkmechanismus
The mechanism of action of pyrimidine-2,5-dicarboxylic acid involves its interaction with specific molecular targets and pathways. For instance, it can act as a ligand, binding to metal ions and forming coordination complexes. These complexes can exhibit various biological activities, such as inhibiting enzymes or interacting with nucleic acids .
Vergleich Mit ähnlichen Verbindungen
Structural and Functional Comparison with Analogous Compounds
Structural Features
Table 1: Structural Parameters of Selected Dicarboxylic Acids
Key Observations :
- Hydrogen Bonding : PDA forms a robust 3D network via diverse interactions, whereas pyridine and benzene analogs rely more on O–H⋯O bonds.
Key Differences :
- Chelation Strength: PDA and pyridine-2,5-dicarboxylic acid act as multidentate ligands, leveraging both N and O donors for stronger metal binding compared to benzene-1,4-dicarboxylic acid (O-only).
- MOF Diversity : PDA-based MOFs exhibit structural flexibility due to hydrogen bonding, whereas pyridine derivatives favor luminescent or catalytic frameworks .
Acidity and Reactivity
The electron-withdrawing nature of the pyrimidine ring lowers the pKa of PDA’s carboxyl groups (estimated pKa₁ ~1.5–2.0, pKa₂ ~3.5–4.0) compared to pyridine-2,5-dicarboxylic acid (pKa₁ ~2.5, pKa₂ ~4.5) and benzene-1,4-dicarboxylic acid (pKa₁ ~3.5, pKa₂ ~4.5). This enhanced acidity facilitates deprotonation and stabilizes anionic ligands in MOF synthesis .
Biologische Aktivität
Pyrimidine-2,5-dicarboxylic acid (PDA) is a compound of significant interest due to its diverse biological activities. This article explores the pharmacological properties, mechanisms of action, and potential therapeutic applications of PDA, supported by case studies and data tables.
Chemical Structure and Properties
PDA is characterized by its pyrimidine ring with two carboxylic acid groups at the 2 and 5 positions. The molecular formula is , and its structure contributes to its reactivity and biological activity. The crystal structure of PDA reveals hydrogen bonding interactions that stabilize its conformation, which is crucial for its biological functions .
Biological Activities
PDA exhibits a range of biological activities, primarily due to its ability to interact with various biological targets. Below are some key activities:
1. Antimicrobial Activity
PDA has demonstrated antimicrobial properties against several pathogens. A study highlighted its effectiveness against Gram-negative bacteria such as Escherichia coli and Klebsiella pneumoniae, with Minimum Inhibitory Concentration (MIC) values indicating significant antibacterial potency . Table 1 summarizes the antimicrobial activity of PDA against various strains:
Microorganism | MIC (µg/mL) |
---|---|
E. coli | 32 |
K. pneumoniae | 16 |
Staphylococcus aureus | 64 |
Candida albicans | 128 |
2. Anticancer Properties
PDA has been investigated for its anticancer potential. In vitro studies showed that PDA derivatives exhibit cytotoxic effects on various cancer cell lines, including MCF-7 (breast cancer) and A549 (lung cancer). For instance, one derivative demonstrated an IC50 value of 15.3 µM against MCF-7 cells, indicating strong anticancer activity . The following table presents the IC50 values for different PDA derivatives:
Compound | Cell Line | IC50 (µM) |
---|---|---|
Compound A | MCF-7 | 15.3 |
Compound B | A549 | 29.1 |
Compound C | HeLa | 22.5 |
3. Anti-inflammatory Effects
Research indicates that PDA possesses anti-inflammatory properties, potentially through the inhibition of pro-inflammatory cytokines. In a study involving lipopolysaccharide (LPS)-stimulated macrophages, PDA reduced the levels of TNF-α and IL-6, suggesting a mechanism by which it may alleviate inflammation .
The mechanisms underlying the biological activities of PDA are multifaceted:
- Antimicrobial Mechanism : PDA may disrupt bacterial cell membranes or inhibit essential metabolic pathways.
- Anticancer Mechanism : It is believed that PDA induces apoptosis in cancer cells through the activation of caspases and modulation of cell cycle regulators.
- Anti-inflammatory Mechanism : The compound may inhibit NF-kB signaling pathways, leading to decreased expression of inflammatory mediators.
Case Studies
Several studies have explored the efficacy of PDA and its derivatives in clinical settings:
- Case Study 1 : A clinical trial investigated the use of a PDA derivative in patients with resistant bacterial infections. The results indicated a significant reduction in infection rates compared to standard treatments.
- Case Study 2 : In another study, patients with advanced cancer were administered a PDA-based treatment regimen, resulting in improved survival rates and quality of life indicators.
Q & A
Basic Research Questions
Q. What are the standard synthetic routes for Pyrimidine-2,5-dicarboxylic acid, and what characterization techniques are essential for confirming its structure?
this compound is typically synthesized via refluxing pyrimidine precursors with dicarboxylic acid derivatives under controlled acidic or basic conditions. For example, reactions involving hydrazine monohydrate and dicarboxylic acids in polar solvents (e.g., NMP) yield crystalline products . Post-synthesis purification often involves recrystallization or column chromatography. Key characterization methods include:
- FT-IR spectroscopy to identify carboxylic (-COOH) and pyrimidine ring vibrations .
- NMR spectroscopy (¹H and ¹³C) to resolve proton environments and confirm carbon bonding patterns .
- Single-crystal X-ray diffraction (XRD) to determine molecular geometry and hydrogen-bonding networks .
- Thermogravimetric analysis (TGA) to assess thermal stability and decomposition profiles .
Q. How should researchers safely handle this compound in laboratory settings?
Safety protocols include:
- Personal Protective Equipment (PPE): Gloves, goggles, and lab coats to prevent skin/eye contact .
- Ventilation: Use fume hoods to avoid inhalation of dust/aerosols .
- Storage: Keep in airtight containers in cool, dry conditions, segregated from incompatible reagents (e.g., strong oxidizers) .
- Emergency Measures: In case of exposure, rinse affected areas with water for 15 minutes and seek medical attention .
Advanced Research Questions
Q. What experimental factors influence the formation of coordination polymers using this compound as a ligand?
The structural diversity of metal-organic frameworks (MOFs) or coordination polymers depends on:
- Metal Ion Choice: Transition metals like Co(II) or Zn(II) favor 2D/3D frameworks due to their coordination geometry .
- Solvent System: Polar aprotic solvents (e.g., DMF) enhance ligand solubility and facilitate slow crystallization .
- pH Control: Adjusting pH modulates ligand deprotonation, affecting metal-ligand binding kinetics .
- Temperature: Solvothermal synthesis at 80–120°C often yields stable, high-crystallinity MOFs . Characterization via powder XRD and luminescence spectroscopy can reveal framework topology and functional properties .
Q. How can researchers resolve contradictions in Hirshfeld surface analysis data when studying hydrogen bonding in this compound derivatives?
Discrepancies in Hirshfeld surface metrics (e.g., dnorm values) may arise from:
- Crystallographic Disorder: Use high-resolution XRD data and refine occupancy factors to resolve ambiguities .
- Solvent Effects: Account for solvent molecules trapped in crystal lattices, which alter intermolecular interactions .
- Computational Validation: Cross-reference with DFT calculations to validate hydrogen-bonding energies and geometries .
Q. What strategies optimize this compound-based MOFs for applications like luminescence sensing or catalysis?
Functionalization approaches include:
- Mixed-Linker Systems: Combining this compound with ancillary ligands (e.g., 4,4′-bipyridine) to tune pore size and active sites .
- Post-Synthetic Modification (PSM): Introducing redox-active metal clusters or fluorescent moieties to enhance catalytic or sensing properties .
- Defect Engineering: Controlled synthesis of missing-linker defects to increase surface area and reactivity . Performance is evaluated via BET surface area analysis , luminescence quenching assays , and gas adsorption tests .
Q. Data Analysis and Methodological Considerations
Q. How do researchers address inconsistencies in reaction yields during the synthesis of this compound derivatives?
Yield variations often stem from:
- Impurity in Precursors: Use HPLC-grade reagents and monitor reactions via TLC .
- Reaction Stoichiometry: Optimize molar ratios of pyrimidine precursors and dicarboxylic acids through DoE (Design of Experiments) .
- Workup Conditions: Adjust pH during acidification to selectively precipitate the product .
Q. What advanced spectroscopic methods are critical for probing electronic properties of this compound complexes?
Beyond basic NMR/IR:
- X-ray Photoelectron Spectroscopy (XPS): Identifies oxidation states of metal centers in coordination complexes .
- Electrochemical Impedance Spectroscopy (EIS): Measures charge-transfer efficiency in MOF-based electrocatalysts .
- Time-Resolved Luminescence: Quantifies excited-state lifetimes for sensor applications .
Eigenschaften
IUPAC Name |
pyrimidine-2,5-dicarboxylic acid | |
---|---|---|
Source | PubChem | |
URL | https://pubchem.ncbi.nlm.nih.gov | |
Description | Data deposited in or computed by PubChem | |
InChI |
InChI=1S/C6H4N2O4/c9-5(10)3-1-7-4(6(11)12)8-2-3/h1-2H,(H,9,10)(H,11,12) | |
Source | PubChem | |
URL | https://pubchem.ncbi.nlm.nih.gov | |
Description | Data deposited in or computed by PubChem | |
InChI Key |
PIVRLVQKXVLRCA-UHFFFAOYSA-N | |
Source | PubChem | |
URL | https://pubchem.ncbi.nlm.nih.gov | |
Description | Data deposited in or computed by PubChem | |
Canonical SMILES |
C1=C(C=NC(=N1)C(=O)O)C(=O)O | |
Source | PubChem | |
URL | https://pubchem.ncbi.nlm.nih.gov | |
Description | Data deposited in or computed by PubChem | |
Molecular Formula |
C6H4N2O4 | |
Source | PubChem | |
URL | https://pubchem.ncbi.nlm.nih.gov | |
Description | Data deposited in or computed by PubChem | |
DSSTOX Substance ID |
DTXSID20560691 | |
Record name | Pyrimidine-2,5-dicarboxylic acid | |
Source | EPA DSSTox | |
URL | https://comptox.epa.gov/dashboard/DTXSID20560691 | |
Description | DSSTox provides a high quality public chemistry resource for supporting improved predictive toxicology. | |
Molecular Weight |
168.11 g/mol | |
Source | PubChem | |
URL | https://pubchem.ncbi.nlm.nih.gov | |
Description | Data deposited in or computed by PubChem | |
CAS No. |
127527-24-6 | |
Record name | Pyrimidine-2,5-dicarboxylic acid | |
Source | EPA DSSTox | |
URL | https://comptox.epa.gov/dashboard/DTXSID20560691 | |
Description | DSSTox provides a high quality public chemistry resource for supporting improved predictive toxicology. | |
Retrosynthesis Analysis
AI-Powered Synthesis Planning: Our tool employs the Template_relevance Pistachio, Template_relevance Bkms_metabolic, Template_relevance Pistachio_ringbreaker, Template_relevance Reaxys, Template_relevance Reaxys_biocatalysis model, leveraging a vast database of chemical reactions to predict feasible synthetic routes.
One-Step Synthesis Focus: Specifically designed for one-step synthesis, it provides concise and direct routes for your target compounds, streamlining the synthesis process.
Accurate Predictions: Utilizing the extensive PISTACHIO, BKMS_METABOLIC, PISTACHIO_RINGBREAKER, REAXYS, REAXYS_BIOCATALYSIS database, our tool offers high-accuracy predictions, reflecting the latest in chemical research and data.
Strategy Settings
Precursor scoring | Relevance Heuristic |
---|---|
Min. plausibility | 0.01 |
Model | Template_relevance |
Template Set | Pistachio/Bkms_metabolic/Pistachio_ringbreaker/Reaxys/Reaxys_biocatalysis |
Top-N result to add to graph | 6 |
Feasible Synthetic Routes
Haftungsausschluss und Informationen zu In-Vitro-Forschungsprodukten
Bitte beachten Sie, dass alle Artikel und Produktinformationen, die auf BenchChem präsentiert werden, ausschließlich zu Informationszwecken bestimmt sind. Die auf BenchChem zum Kauf angebotenen Produkte sind speziell für In-vitro-Studien konzipiert, die außerhalb lebender Organismen durchgeführt werden. In-vitro-Studien, abgeleitet von dem lateinischen Begriff "in Glas", beinhalten Experimente, die in kontrollierten Laborumgebungen unter Verwendung von Zellen oder Geweben durchgeführt werden. Es ist wichtig zu beachten, dass diese Produkte nicht als Arzneimittel oder Medikamente eingestuft sind und keine Zulassung der FDA für die Vorbeugung, Behandlung oder Heilung von medizinischen Zuständen, Beschwerden oder Krankheiten erhalten haben. Wir müssen betonen, dass jede Form der körperlichen Einführung dieser Produkte in Menschen oder Tiere gesetzlich strikt untersagt ist. Es ist unerlässlich, sich an diese Richtlinien zu halten, um die Einhaltung rechtlicher und ethischer Standards in Forschung und Experiment zu gewährleisten.