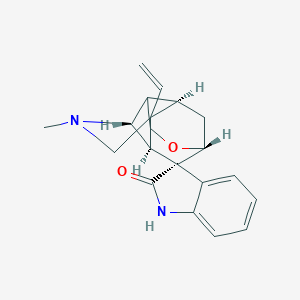
Gelsemine
Beschreibung
Significance as a Monoterpenoid Indole Alkaloid in Natural Product Chemistry
Gelsemine holds a notable position in natural product chemistry due to its classification as a monoterpenoid indole alkaloid. wikipedia.org This class of compounds is characterized by structures containing both an indole moiety (derived from tryptophan) and a monoterpene unit (derived from isoprene units). nih.gov The unique and complex polycyclic architecture of this compound, featuring a spiro-indolinone nucleus and multiple stereocenters, contributes to its chemical diversity and structural complexity, making it an attractive target for synthetic chemists. nih.govbenthamdirect.com
Monoterpenoid indole alkaloids from the Gelsemium genus, including this compound, are considered major active ingredients and have been extensively studied for their diverse chemical structures and potential biological activities. nih.govresearchgate.net Over 200 alkaloids have been reported from Gelsemium and its endophytic fungi, highlighting the genus as a rich source of these natural products. researchgate.net this compound itself is identified as a principal alkaloid, particularly from Gelsemium sempervirens. researchgate.net
Historical Context of Research on Gelsemium Alkaloids
Research into Gelsemium alkaloids, and indirectly this compound, has a history spanning over a hundred years. wikipedia.org The presence of alkaloids in Gelsemium sempervirens was initially established by Wormley, who extracted an amorphous base. capes.gov.br Sonnenschein later obtained an amorphous base, which he named this compound and assigned a chemical formula based on analyses of the free base and its salts. capes.gov.br Gerrard is credited with being the first to obtain crystalline, albeit impure, this compound. capes.gov.br
The isolation of this compound from Gelsemium sempervirens occurred in 1870. wikipedia.orgthieme-connect.de Its chemical formula was determined to be C₂₀H₂₂N₂O₂. wikipedia.org The complex structure of this compound was finally elucidated much later, in 1959, through the application of X-ray crystallographic analysis and nuclear magnetic resonance (NMR) spectroscopy by Conroy and Chakrabarti. wikipedia.orgcapes.gov.br This structural determination was a significant step in understanding the chemistry of this intricate natural product.
The unique structure and potential bio-pharmacological properties of this compound have spurred significant interest in its total synthesis, with the first racemic total synthesis reported in 1994. benthamdirect.comthieme-connect.de Since then, several other total syntheses have been achieved by different research groups, employing various synthetic strategies to tackle its challenging molecular architecture. benthamdirect.comthieme-connect.de
Research findings have indicated that this compound is a modulator of inhibitory neurotransmitter receptors, specifically glycine receptors (GlyRs) and GABAA receptors (GABAARs). mdpi.comnih.gov Studies have explored its interactions with these ligand-gated ion channels in the central nervous system. mdpi.comnih.gov For instance, research suggests that this compound's modulatory effects on GlyRs may involve binding to the orthosteric site. mdpi.com
While this article focuses on the chemical and historical aspects of this compound research within academic contexts, the compound's complex structure and its presence in Gelsemium plants have made it a compelling subject for investigation in natural product chemistry and synthetic methodology.
Eigenschaften
Key on ui mechanism of action |
GELSEMINE ANTAGONIZED THE INHIBITORY EFFECTS OF GLYCINE, BETA-ALANINE, L-ALPHA-ALANINE, TAURINE & NORADRENALINE ON SPINAL NEURONS OF CATS. |
---|---|
CAS-Nummer |
509-15-9 |
Molekularformel |
C20H22N2O2 |
Molekulargewicht |
322.4 g/mol |
IUPAC-Name |
(2'S,3S)-2'-ethenyl-4'-methylspiro[1H-indole-3,7'-9-oxa-4-azatetracyclo[6.3.1.02,6.05,11]dodecane]-2-one |
InChI |
InChI=1S/C20H22N2O2/c1-3-19-10-22(2)16-11-9-24-15(8-13(11)19)20(17(16)19)12-6-4-5-7-14(12)21-18(20)23/h3-7,11,13,15-17H,1,8-10H2,2H3,(H,21,23)/t11?,13?,15?,16?,17?,19-,20-/m0/s1 |
InChI-Schlüssel |
NFYYATWFXNPTRM-KJWRJJNGSA-N |
SMILES |
CN1CC2(C3CC4C5(C2C1C3CO4)C6=CC=CC=C6NC5=O)C=C |
Isomerische SMILES |
CN1C[C@]2(C3CC4[C@]5(C2C1C3CO4)C6=CC=CC=C6NC5=O)C=C |
Kanonische SMILES |
CN1CC2(C3CC4C5(C2C1C3CO4)C6=CC=CC=C6NC5=O)C=C |
Aussehen |
Powder |
Color/Form |
CRYSTALS FROM ACETONE |
melting_point |
178 °C |
Andere CAS-Nummern |
509-15-9 |
Piktogramme |
Acute Toxic |
Löslichkeit |
SLIGHTLY SOL IN WATER; SOL IN ALCOHOL, BENZENE, CHLOROFORM, ETHER, ACETONE, DILUTE ACIDS |
Synonyme |
(+)-Gelsemine; NSC 21729; [3R-(3α,4aβ,5α,8α,8aβ,9S*,10S*)]-5-Ethenyl-3,4,4a,5,6,7,8,8a-octahydro-7-methylspiro[3,5,8-ethanylylidene-1H-pyrano[3,4-c]pyridine-10,3’-[3H]indol]-2’(1’H)-one; (3R,3’S,4aR,5S,8S,8aS,9S)-5-Ethenyl-3,4,4a,5,6,7,8,8a-octahydro |
Herkunft des Produkts |
United States |
Structural Elucidation and Stereochemical Assignment Methodologies
Early Methodologies in Structural Determination of Gelsemine
This compound was first isolated in 1870 from Gelsemium sempervirens. wikipedia.orgresearchgate.net Initial investigations focused on determining its empirical formula and identifying key functional groups through chemical degradation and derivatization reactions. Early studies established its chemical formula as C20H22N2O2. wikipedia.orgresearchgate.net However, the complex polycyclic nature of the molecule and the arrangement of its atoms remained unknown for many years. Classical methods, while providing clues about the elemental composition and some functionalitites, were insufficient to fully unravel the intricate cage structure and the spatial arrangement of its atoms. researchgate.net
Advanced Spectroscopic and Crystallographic Approaches for Complex Alkaloid Structures
The definitive structural elucidation and stereochemical assignment of this compound were achieved in 1959 through the application of advanced techniques available at the time: X-ray crystallography and Nuclear Magnetic Resonance (NMR) spectroscopy. wikipedia.orgwikiwand.com
X-ray Crystallography: This technique proved crucial in determining the three-dimensional arrangement of atoms in crystalline this compound or its derivatives. By analyzing the diffraction pattern produced when X-rays interact with the crystal lattice, researchers could deduce the electron density map and, subsequently, the precise positions of atoms and their connectivity. wikipedia.orgazolifesciences.com Lovell and coworkers utilized X-ray crystallography in 1959 to elucidate the structure of this compound hydrohalides, providing the first clear picture of the molecule's complex architecture. researchgate.netdntb.gov.ua X-ray crystallography remains a powerful tool for the unambiguous determination of molecular structures, especially for complex natural products that can be obtained in crystalline form. rsc.orgamazon.com
Nuclear Magnetic Resonance (NMR) Spectroscopy: Concurrently with crystallographic studies, NMR spectroscopy played a vital role in confirming and refining the structural assignment. wikipedia.orgwikiwand.com Early 1H NMR studies provided information about the types of protons present and their connectivity based on spin-spin coupling patterns. As NMR technology advanced, techniques such as 13C NMR and two-dimensional NMR experiments (e.g., COSY, HSQC, HMBC) became invaluable for mapping the carbon skeleton and establishing correlations between protons and carbons across multiple bonds. slideshare.netresearchgate.netnih.gov These spectroscopic data provided crucial constraints that complemented the crystallographic findings and helped to fully assign the structure and relative stereochemistry of this compound. dntb.gov.uaacs.org
The combination of X-ray crystallography and NMR spectroscopy was particularly powerful for a molecule like this compound with its multiple rings and stereocenters, allowing for the confident assignment of its complex structure and stereochemistry. wikipedia.orgwikiwand.comresearchgate.net Modern NMR techniques, including high-field magnets and advanced pulse sequences, continue to be essential for the structure elucidation of novel and complex alkaloids. slideshare.netnih.gov Mass spectrometry (MS), particularly hyphenated techniques like LC-MS/MS, also plays a significant role in determining the molecular weight and fragmentation patterns, providing complementary information for structural confirmation and the identification of related alkaloids. researchgate.netslideshare.netoup.comnih.gov
The successful structural determination of this compound in 1959 by Conroy and Chakrabarti using NMR and by Lovell and coworkers using X-ray crystallography marked a significant achievement in natural product chemistry and laid the foundation for further research into this fascinating alkaloid. wikipedia.orgresearchgate.netwikiwand.comdntb.gov.ua
Method | Contribution to this compound Structure Elucidation | Key Findings |
Early Chemical Methods | Elemental analysis, functional group tests, degradation reactions. | Empirical formula (C20H22N2O2). wikipedia.orgresearchgate.net |
X-ray Crystallography | Provided 3D atomic arrangement and connectivity in solid state. | Definitive cage structure and relative stereochemistry. researchgate.netdntb.gov.ua |
NMR Spectroscopy (Early) | Information on proton types and coupling patterns. | Insights into local structural fragments. |
NMR Spectroscopy (Advanced) | Detailed carbon skeleton, proton-carbon correlations, spatial proximity (NOE). | Confirmation of connectivity, assignment of relative stereochemistry. dntb.gov.uaacs.org |
Mass Spectrometry | Molecular weight determination, fragmentation analysis. | Confirmation of molecular formula, identification of structural subunits. oup.comnih.gov |
Table 1: Summary of Methodologies Used in this compound Structure Elucidation
Biosynthetic Investigations of Gelsemine
Proposed Biosynthetic Pathways from Precursor Molecules (e.g., Strictosidine)
The central precursor for essentially all monoterpenoid indole alkaloids, including gelsemine, is 3α(S)-strictosidine. wikipedia.orgwikiwand.com Strictosidine is formed in a single enzyme-catalyzed step from the condensation of tryptamine and secologanin. wikipedia.orgwikiwand.combiorxiv.org Tryptamine is derived from the shikimate pathway, while secologanin is a terpene produced via the mevalonic acid pathway. wikipedia.orgwikiwand.combiorxiv.org
From strictosidine, the biosynthetic pathway to this compound is thought to involve a series of intermediates. While the complete pathway is still under investigation, proposed intermediates include koumicine (akkuammidine), koumidine, vobasindiol, anhydrovobasindiol, and gelsenidine (humantienine-type). wikipedia.org
Enzymatic Steps and Key Intermediates in this compound Biosynthesis
The biosynthesis of MIAs begins with the strictosidine biosynthetic gene cluster (BGC), which involves enzymes such as tryptophan decarboxylase (TDC), strictosidine synthase (STR), and a Multidrug and Toxic Compound Extrusion (MATE) transporter (MATE1). biorxiv.orgresearchgate.net TDC and STR are responsible for the condensation of tryptamine and secologanin to form strictosidine. biorxiv.org MATE1 is thought to facilitate this reaction by transporting secologanin into the vacuole where STR is located. biorxiv.org
Following the formation of strictosidine, the pathway diverges to produce various MIA skeletons. researchgate.net For this compound, the proposed intermediates suggest a complex series of enzymatic transformations, including rearrangements and cyclizations, to form its characteristic spiro-indolinone core and caged structure. wikipedia.orgresearchgate.net While specific enzymes catalyzing each step from strictosidine to this compound are not fully characterized, studies in Gelsemium sempervirens have identified conserved key enzymes like GsTDC, GsSTR, and GsSGD (strictosidine glucosidase) that are exclusively produced in the roots. researchgate.net Strictosidine glucosidase acts on strictosidine to produce strictosidine aglycone, which is a key branch point in MIA biosynthesis. biorxiv.orgresearchgate.net
Proposed Key Intermediates in this compound Biosynthesis from Strictosidine:
Intermediate Name | Type |
Strictosidine | Indole alkaloid |
Koumicine (Akkuammidine) | Indole alkaloid |
Koumidine | Indole alkaloid |
Vobasindiol | Indole alkaloid |
Anhydrovobasindiol | Indole alkaloid |
Gelsenidine (Humantienine-type) | Indole alkaloid |
This compound | Oxindole alkaloid |
Note: This table is based on proposed intermediates as of 1998. wikipedia.org
Relationship to Other Gelsemium Alkaloids Biosynthesis
The Gelsemium genus produces a diverse array of monoterpenoid indole alkaloids, which can be classified into several types, including gelsedine, this compound, humantenine, koumine, sarpagine, and yohimbane types. tandfonline.comresearchgate.netresearchgate.net All these alkaloids share strictosidine as a common precursor. wikipedia.orgwikiwand.combiorxiv.org
The divergence in the biosynthetic pathways after strictosidine aglycone leads to the different structural classes of Gelsemium alkaloids. researchgate.net For instance, koumine and gelsemicine are also derived from the same general pathway as this compound. wikipedia.org Koumine is thought to be derived from anhydrovobasindiol through oxidation and rearrangement, while gelsemicine may be formed from this compound itself via aromatic oxidation and O-methylation. wikipedia.org
Studies have shown that while some gene clusters involved in MIA biosynthesis are conserved between Gelsemium sempervirens and other MIA-producing plants like Catharanthus roseus, the enzymes may act on different substrates, leading to the structural diversity observed in Gelsemium alkaloids. researchgate.net The spatiotemporal distribution of different alkaloids within Gelsemium elegans also suggests complex and potentially interconnected biosynthetic processes occurring in different tissues and growth stages. frontiersin.org
Synthetic Strategies Towards Gelsemine
Retrosynthetic Analyses and Key Disconnections
Retrosynthetic analysis of Gelsemine typically involves identifying key bonds and functional groups that can be disconnected to yield simpler, more readily available starting materials. Common disconnections focus on the spiro-oxindole junction, the bicyclo[3.2.1]octane core, and the formation of the heterocyclic rings. researchgate.netresearchgate.net Strategies often involve constructing the core structure first, followed by the elaboration of the peripheral rings and installation of the spiro-oxindole. bham.ac.ukacs.org
Strategies for the Spiro-Oxindole Moiety Construction
The construction of the spiro-oxindole moiety, a key feature of this compound, is a significant synthetic challenge, often leading to mixtures of stereoisomers. bham.ac.ukjst.go.jp Various strategies have been employed to address this, including metal-catalyzed free-radical C-H activation and intramolecular Heck reactions. bham.ac.uknih.gov
Several methods have been developed to install the spiro-oxindole, with varying degrees of stereocontrol. These include radical cyclization, intramolecular Heck reaction, and acid-mediated cyclization, some of which yield mixtures of isomers. bham.ac.uk Fukuyama's group reported a stereoselective installation of the spiro-oxindole using a Knoevenagel-like condensation followed by a divinylcyclopropane – cycloheptadiene rearrangement. bham.ac.ukjst.go.jp Danishefsky's synthesis involved a complex route to access the spiro-oxindole as a single isomer. bham.ac.uk More recent approaches have explored novel synthetic methods for spiro-oxindole construction, including the photolysis of alkoxy-substituted-1-alkenylbenzotriazoles. researchgate.netresearchgate.netrsc.org
Table 1 summarizes some of the reported methods for spiro-oxindole installation and their diastereomeric ratios (d.r.).
Method | Year | Diastereomeric Ratio (Desired/Undesired) |
Johnson radical cyclisation | 1994 | 1 : 2 |
Hart radical cyclisation | 1994 | 1.6 : 1 |
Speckamp intramolecular Heck reaction | 1994 | 2 : 1 |
Fukuyama divinylcyclopropane-cycloheptadiene rearrangement | 1996 | >99 : 1 |
Overman intramolecular Heck reaction | 1999 | 1 : 11 |
Fukuyama divinylcyclopropane-cycloheptadiene rearrangement | 2000 | >99 : 1 |
Danishefsky Eschenmoser-Claisen rearrangement | 2002 | >99 : 1 |
Aubé intramolecular Heck reaction | 2007 | 1.7 : 1 |
Qin condensation and acid-mediated cyclisation | 2012 | 10 : 1 |
Approaches to the Bicyclo[3.2.1]octane Core
The bicyclo[3.2.1]octane core is another central structural feature of this compound, and its construction has been approached through various strategies. researchgate.netnih.govresearchgate.netscispace.com Approaches include [4+3] cycloaddition reactions and intramolecular Michael addition steps. bham.ac.uk
One strategy involves the rapid construction of the bicyclo[3.2.1]octane core using a [4+3] cycloaddition. bham.ac.uk Another approach utilizes a bridge swapping strategy starting from an oxabicyclo[3.2.1]octanone, involving elimination of the bridging ether oxygen and intramolecular Michael addition of a tethered cyanoacetamide unit. acs.org Stork and co-workers developed a novel construction of the core structure using a Claisen rearrangement. bham.ac.uk Johnson's group synthesized a tricyclic intermediate that underwent Mannich cyclization to afford the bicyclo[3.2.1]octane ring. bham.ac.uk Metal-catalyzed cycloisomerization-Cope rearrangement sequences have also been employed to construct the central core. researchgate.netnih.govresearchgate.net
Formation of the Pyrrolidine and Tetrahydropyran Rings
The pyrrolidine and tetrahydropyran rings are integral parts of the this compound cage structure. nih.govbham.ac.ukscispace.com The formation of these rings often occurs in later stages of the synthesis or can be integrated into the construction of the core. bham.ac.uk
The pyrrolidine ring has been constructed using methods such as intramolecular reactions between N-acyliminium ions and allylsilane groups. bham.ac.uk Fukuyama's group reported an intramolecular Michael addition to construct the pyrrolidine ring in their enantioselective total synthesis. bham.ac.uk Danishefsky's method for creating the pyrrolidine ring involved a Lewis acid catalyzed intramolecular displacement. researchgate.netresearchgate.net
The tetrahydropyran ring formation has been achieved through various methods, including intramolecular oxymercuration. bham.ac.ukuva.nl Fleming and co-workers reported a tetrahydropyran synthesis using a base-mediated cyclization step. bham.ac.uk In some syntheses, the tetrahydropyran ring is formed concurrently with the construction of the core structure, for instance, through a Claisen rearrangement or Mannich cyclization. bham.ac.uk Selective reduction of a tricyclic ketone can lead to an alcohol that cyclizes to form a lactone, serving as a precursor to the tetrahydropyran ring. bham.ac.uk
Total Synthesis Approaches
This compound's complex structure has inspired numerous total syntheses, both racemic and asymmetric, since the first reports in the early 1990s. wikipedia.orgbenthamdirect.comresearchgate.netutexas.edubaranlab.orgresearchgate.net These syntheses showcase a variety of innovative chemical transformations and strategies to assemble the hexacyclic framework. benthamdirect.comresearchgate.net
Racemic Total Syntheses Methodologies
Several racemic total syntheses of this compound have been reported, each employing distinct key steps and strategies. wikipedia.orgutexas.edubaranlab.orgbham.ac.ukresearchgate.netuni.lumdpi.comu-tokyo.ac.jp Early racemic syntheses were reported by the groups of Johnson, Speckamp, and Hart in 1994. wikipedia.orgutexas.edubaranlab.orgnih.gov Subsequent racemic syntheses were achieved by Fukuyama, Overman, and Danishefsky. wikipedia.orgutexas.edubaranlab.orgnih.gov
Group | Year | Number of Steps | Overall Yield (%) |
Speckamp | 1994 | 19 | 0.83 |
Johnson | 1994 | 29 | 0.58 |
Fukuyama | 1996 | 32 | 0.67 |
Hart | 1997 | 23 | 0.25 |
Overman | 1999 | 26 | 1.2 |
Danishefsky | 2002 | 36 | 0.019 |
Photo-induced [2+2]-Cycloaddition Strategies
Photo-induced [2+2]-cycloaddition reactions have been utilized as a key strategy in some racemic total syntheses of this compound to construct the core ring system. researchgate.netresearchgate.netresearchgate.net The Johnson group, in their 1994 racemic total synthesis, employed an effective photo-[2+2] cycloaddition of a triene intermediate to build the essential tricyclic core structure. researchgate.netresearchgate.net This approach provided a tricyclic diester intermediate possessing key structural features of this compound. researchgate.net Photolysis of alkoxy-substituted-1-alkenylbenzotriazoles has also been explored as a route to spiro-oxindoles, which has been applied in a total synthesis of this compound. researchgate.netrsc.orggoogle.com
N-Acyliminium Ion Cyclization Methodologies
N-Acyliminium ion cyclizations have been utilized in approaches towards the this compound core structure. This strategy involves the intramolecular reaction of a nucleophile with an electrophilic N-acyliminium ion intermediate. One study described the preparation of a tetracyclic skeletal part of this compound through a highly stereospecific intramolecular reaction of a silyl enol ether with an N-acyliminium ion. dntb.gov.ua Another instance involved an iodide-promoted intramolecular reaction of an allene with an N-acyliminium ion intermediate to form a bicyclic vinyl iodide, a key step in the synthesis of gelsedine, a related oxindole alkaloid. researchgate.netnih.gov The cyclization of an N-acyliminium ion derived from an enamide has also been explored, although considerations regarding the stability of the pyran ring under acidic conditions needed to generate the acyliminium cation were noted.
Free Radical Cyclization Approaches
Free radical cyclization reactions have played significant roles in the synthesis of this compound, particularly in constructing key substructures like the tricyclic core and the oxindole moiety. acs.orgacs.org These approaches often involve the generation of carbon-centered radicals that undergo intramolecular addition to double or triple bonds, forming new rings. One study highlighted the importance of free radical cyclizations in building the tricyclic core and the oxindole substructures, and also employed an isomerization-cyclization strategy to complete the this compound cage. acs.orgacs.org Observations in these approaches included intramolecular 1,4- and 1,6-hydrogen atom transfers and a free radical cyclization-fragmentation sequence. acs.org Another reported approach utilized photoinduced intramolecular cycloaddition, which can involve radical intermediates, in the synthesis of a key tricyclic diester intermediate containing 12 of the 13 carbon atoms of a target ketone intermediate.
Intramolecular Mannich Reaction Pathways
Intramolecular Mannich reactions have been employed in this compound synthesis to form crucial carbon-nitrogen bonds and construct cyclic systems. One retrosynthetic analysis suggested that a key bond in this compound could be formed by an intramolecular Mannich reaction of an iminium species, which could be derived from the protonation of an enamine. Another synthetic route involved a sequential anionic aza-Cope rearrangement and Mannich cyclization as key steps in the total synthesis of (±)-gelsemine. nih.govresearchgate.net The preparation of a precursor for a Mannich cyclization from a Diels-Alder reaction between N-methylmaleimide and (E)-hex-3,5-dien-1-ol has also been reported. bham.ac.uk
Divinylcyclopropane-Cycloheptadiene Rearrangement
The divinylcyclopropane-cycloheptadiene rearrangement, a type of Cope rearrangement, has been a powerful tool in constructing the complex ring system of this compound. baranlab.orguni-konstanz.derice.edu This thermal rearrangement allows for the conversion of a divinylcyclopropane into a seven-membered cycloheptadiene ring. In the context of this compound synthesis, this rearrangement has been utilized to construct the desired quaternary center and the spiro-oxindole system with high stereoselectivity. bham.ac.ukbeilstein-journals.org One synthesis reported a very smooth divinylcyclopropane-cycloheptadiene rearrangement of a trans-divinylcyclopropane at elevated temperature, noting that the presence of electron-withdrawing groups on the divinylcyclopropane could rationalize the unusually low temperature required for a preceding trans-cis isomerization. beilstein-journals.org
Eschenmoser-Claisen Rearrangement in Total Synthesis
The Eschenmoser-Claisen rearrangement, a princeton.eduprinceton.edu-sigmatropic rearrangement, has been applied in the total synthesis of this compound. This rearrangement typically involves the reaction of an allylic alcohol with an amide acetal or ortho ester derivative to form a γ,δ-unsaturated amide or ester. In one total synthesis of this compound, the Eschenmoser-Claisen rearrangement was identified as a key step. baranlab.orgacs.org Modeling studies have explored the stereoselectivity of Johnson-Claisen rearrangements, a variant of the Claisen rearrangement, in the synthesis of this compound, investigating the factors influencing the facial stereoselectivity. acs.org
Asymmetric Total Syntheses Methodologies
Achieving the correct stereochemistry is paramount in the synthesis of natural products like this compound, which possesses multiple chiral centers. Several asymmetric total syntheses of this compound have been reported, employing various strategies to control stereochemistry. researchgate.netbenthamdirect.comresearchgate.netthieme-connect.com Among the reported total syntheses, at least three have been asymmetric. researchgate.netbenthamdirect.com
Organocatalytic Diels-Alder Reaction Strategies
Organocatalytic Diels-Alder reactions have emerged as a powerful strategy for the asymmetric construction of cyclic systems in the synthesis of complex molecules like this compound. This approach utilizes small organic molecules as catalysts to promote the Diels-Alder reaction with high levels of stereo- and enantioselectivity. One asymmetric total synthesis of (+)-gelsemine featured a highly diastereoselective and enantioselective organocatalytic Diels-Alder reaction as a key step. benthamdirect.comdntb.gov.uaresearchgate.netnih.govamanote.com This strategy is considered a significant advancement, potentially offering advantages over traditional metal catalysis. researchgate.net
Enol-Oxonium Cyclization Approaches
Enol-oxonium cyclization strategies have been successfully employed in the total synthesis of this compound, particularly in approaches aiming to mimic the proposed biosynthetic pathway. benthamdirect.comresearchgate.netnih.govresearchgate.net This type of cyclization is instrumental in constructing key cyclic systems and establishing crucial stereocenters within the complex this compound framework.
One notable application of this strategy involves a multistep, one-pot enol-oxonium cyclization cascade. researchgate.netnih.govresearchgate.net This cascade has been shown to simultaneously construct the E and F rings of this compound and establish the stereochemistry at the C3 and C7 quaternary carbon centers. researchgate.netnih.govresearchgate.net The Qin group's 2012 biomimetic total synthesis of (+)-Gelsemine utilized an enol-oxonium cyclization cascade as a key step to assemble the cage structure, demonstrating its efficacy in constructing the core scaffold. nih.govresearchgate.netthieme-connect.com This approach commenced from a starting material derived from D-diethyl tartrate. researchgate.net
Intramolecular SN2 Substitution
In one asymmetric total synthesis of (+)-Gelsemine, an intramolecular SN2 substitution was employed as a late-stage transformation to construct the final this compound skeleton. researchgate.netresearchgate.netnih.gov This highlights the utility of this reaction in closing key rings and achieving the desired connectivity in complex natural product synthesis.
Catalytic Cycloisomerization Strategies
Catalytic cycloisomerization strategies offer efficient pathways to construct cyclic systems from acyclic or less complex precursors, and they have been explored in the context of this compound synthesis and the synthesis of related Gelsemium alkaloids. researchgate.netnih.govnih.govrsc.org These strategies often utilize transition metal catalysts to promote the rearrangement and cyclization of substrates.
Palladium-catalyzed cycloisomerization has been investigated for the construction of the this compound core structure. researchgate.netrsc.org One study reported a sequence-controlled palladium-catalyzed cycloisomerization cascade that successfully formed fused and spirocyclic rings in a single step, providing access to the tetracyclic core of this compound. researchgate.netrsc.org This approach demonstrated the potential of cascade cycloisomerization reactions for rapid complexity build-up. Gold catalysis has also been employed in cycloisomerization strategies towards Gelsemium alkaloids, including efforts related to accessing this compound and Gelsevirine through a "Common Core" strategy. nih.govuga.edu
Biomimetic Synthesis Approaches
Biomimetic synthesis approaches to this compound aim to emulate the proposed biosynthetic pathway of the alkaloid in a laboratory setting. researchgate.netnih.govresearchgate.netthieme-connect.comresearchgate.netuga.edubham.ac.ukexlibrisgroup.com These strategies are often guided by hypotheses about how the plant constructs the complex this compound scaffold from simpler precursors.
A prominent feature of biomimetic syntheses of this compound is the use of cascade reactions, such as the enol-oxonium cyclization cascade, to form multiple rings and stereocenters in a single operation, mirroring the efficiency of enzymatic processes in nature. benthamdirect.comresearchgate.netnih.govresearchgate.netthieme-connect.com The 2012 total synthesis of (+)-Gelsemine by the Qin group is a notable example of a biomimetic approach that successfully utilized an enol-oxonium cyclization cascade as a key step to form the characteristic cage structure. nih.govresearchgate.netthieme-connect.com These biomimetic strategies not only provide synthetic routes to the natural product but also lend support to proposed biosynthetic routes. researchgate.netnih.govresearchgate.net
Comparative Analysis of Synthetic Efficiencies and Stereocontrol in this compound Total Synthesis
Stereocontrol is paramount in this compound synthesis due to the presence of multiple contiguous stereocenters. benthamdirect.comresearchgate.netresearchgate.net Different strategies employ various methods to control stereochemistry, including the use of chiral starting materials, asymmetric catalysts, and diastereoselective reactions such as the organocatalytic Diels-Alder reaction used in the more efficient 2015 synthesis. researchgate.netresearchgate.netnih.gov The enol-oxonium cyclization cascade has also been shown to form specific stereoisomers. thieme-connect.com
The comparative analysis of reported syntheses highlights the ongoing efforts to develop more efficient and stereoselective routes to this compound, driven by its complex structure and potential biological relevance.
Synthesis Year | Group | Chirality | Number of Steps | Overall Yield (%) | Key Strategies Mentioned |
1994 | Speckamp | Racemic | 19 | 0.83 | |
1994 | Johnson | Racemic | 29 | 0.58 | Oxindole spiroannelation wikipedia.org |
1996 | Fukuyama | Racemic | 32 | 0.67 | |
1997 | Hart | Racemic | 23 | 0.25 | Free Radical Cyclizations wikipedia.org |
1999 | Overman | Racemic | 26 | 1.2 | Aza-Cope Rearrangement−Mannich Cyclizations wikipedia.orgacs.org |
2000 | Fukuyama | Asymmetric | 31 | 0.86 | |
2002 | Danishefsky | Racemic | 36 | 0.019 | |
2012 | Qin | Asymmetric | 25 | 1 | Biomimetic, Enol-Oxonium Cyclization Cascade nih.govresearchgate.netthieme-connect.comresearchgate.net |
2015 | Chen, Zhai, et al. | Asymmetric | 12 | 5 | Organocatalytic Diels-Alder, Intramolecular SN2 researchgate.netresearchgate.netnih.gov |
Compound Names and PubChem CIDs:
Compound Name | PubChem CID |
This compound | 5390854 nih.gov |
D-diethyl tartrate | 123805 |
Gelsevirine | 14217344 mdpi.com |
Gelsenicine | 21123652 mdpi.com |
Koumine | 102004413 mdpi.com |
Strictosidine | 10510 uni.lu |
Tryptamine | 7809 |
Secologanin | 644036 |
Note: PubChem CIDs for compounds other than this compound were found via separate searches to fulfill the request for all mentioned compounds.##
This compound, a complex indole alkaloid isolated from the Gelsemium genus, presents a significant challenge in total synthesis due to its intricate hexacyclic cage structure and the presence of seven contiguous stereocenters, including two quaternary carbons. benthamdirect.comresearchgate.netresearchgate.net Over the past few decades, numerous research groups have developed diverse strategies to construct this challenging molecular architecture, employing various key reactions and cascade sequences. benthamdirect.com These synthetic endeavors have not only provided access to this compound but have also advanced the field of organic synthesis by developing novel methodologies and achieving high levels of stereocontrol.
Enol-Oxonium Cyclization Approaches
Enol-oxonium cyclization strategies have been successfully employed in the total synthesis of this compound, particularly in approaches aiming to mimic the proposed biosynthetic pathway. benthamdirect.comresearchgate.netnih.govresearchgate.net This type of cyclization is instrumental in constructing key cyclic systems and establishing crucial stereocenters within the complex this compound framework.
One notable application of this strategy involves a multistep, one-pot enol-oxonium cyclization cascade. researchgate.netnih.govresearchgate.net This cascade has been shown to simultaneously construct the E and F rings of this compound and establish the stereochemistry at the C3 and C7 quaternary carbon centers. researchgate.netnih.govresearchgate.net The Qin group's 2012 biomimetic total synthesis of (+)-Gelsemine utilized an enol-oxonium cyclization cascade as a key step to assemble the cage structure, demonstrating its efficacy in constructing the core scaffold. nih.govresearchgate.netthieme-connect.com This approach commenced from a starting material derived from D-diethyl tartrate. researchgate.net
Intramolecular SN2 Substitution
In one asymmetric total synthesis of (+)-Gelsemine, an intramolecular SN2 substitution was employed as a late-stage transformation to construct the final this compound skeleton. researchgate.netresearchgate.netnih.gov This highlights the utility of this reaction in closing key rings and achieving the desired connectivity in complex natural product synthesis.
Catalytic Cycloisomerization Strategies
Catalytic cycloisomerization strategies offer efficient pathways to construct cyclic systems from acyclic or less complex precursors, and they have been explored in the context of this compound synthesis and the synthesis of related Gelsemium alkaloids. researchgate.netnih.govnih.govrsc.org These strategies often utilize transition metal catalysts to promote the rearrangement and cyclization of substrates.
Palladium-catalyzed cycloisomerization has been investigated for the construction of the this compound core structure. researchgate.netrsc.org One study reported a sequence-controlled palladium-catalyzed cycloisomerization cascade that successfully formed fused and spirocyclic rings in a single step, providing access to the tetracyclic core of this compound. researchgate.netrsc.org This approach demonstrated the potential of cascade cycloisomerization reactions for rapid complexity build-up. Gold catalysis has also been employed in cycloisomerization strategies towards Gelsemium alkaloids, including efforts related to accessing this compound and Gelsevirine through a "Common Core" strategy. nih.govuga.edu
Biomimetic Synthesis Approaches
Biomimetic synthesis approaches to this compound aim to emulate the proposed biosynthetic pathway of the alkaloid in a laboratory setting. researchgate.netnih.govresearchgate.netthieme-connect.comresearchgate.netuga.edubham.ac.ukexlibrisgroup.com These strategies are often guided by hypotheses about how the plant constructs the complex this compound scaffold from simpler precursors.
A prominent feature of biomimetic syntheses of this compound is the use of cascade reactions, such as the enol-oxonium cyclization cascade, to form multiple rings and stereocenters in a single operation, mirroring the efficiency of enzymatic processes in nature. benthamdirect.comresearchgate.netnih.govresearchgate.netthieme-connect.com The 2012 total synthesis of (+)-Gelsemine by the Qin group is a notable example of a biomimetic approach that successfully utilized an enol-oxonium cyclization cascade as a key step to form the characteristic cage structure. nih.govresearchgate.netthieme-connect.com These biomimetic strategies not only provide synthetic routes to the natural product but also lend support to proposed biosynthetic routes. researchgate.netnih.govresearchgate.net
Comparative Analysis of Synthetic Efficiencies and Stereocontrol in this compound Total Synthesis
Stereocontrol is paramount in this compound synthesis due to the presence of multiple contiguous stereocenters. benthamdirect.comresearchgate.netresearchgate.net Different strategies employ various methods to control stereochemistry, including the use of chiral starting materials, asymmetric catalysts, and diastereoselective reactions such as the organocatalytic Diels-Alder reaction used in the more efficient 2015 synthesis. researchgate.netresearchgate.netnih.gov The enol-oxonium cyclization cascade has also been shown to form specific stereoisomers. thieme-connect.com
The comparative analysis of reported syntheses highlights the ongoing efforts to develop more efficient and stereoselective routes to this compound, driven by its complex structure and potential biological relevance.
Synthesis Year | Group | Chirality | Number of Steps | Overall Yield (%) | Key Strategies Mentioned |
1994 | Speckamp | Racemic | 19 | 0.83 | |
1994 | Johnson | Racemic | 29 | 0.58 | Oxindole spiroannelation wikipedia.org |
1996 | Fukuyama | Racemic | 32 | 0.67 | |
1997 | Hart | Racemic | 23 | 0.25 | Free Radical Cyclizations wikipedia.org |
1999 | Overman | Racemic | 26 | 1.2 | Aza-Cope Rearrangement−Mannich Cyclizations wikipedia.orgacs.org |
2000 | Fukuyama | Asymmetric | 31 | 0.86 | |
2002 | Danishefsky | Racemic | 36 | 0.019 | |
2012 | Qin | Asymmetric | 25 | 1 | Biomimetic, Enol-Oxonium Cyclization Cascade nih.govresearchgate.netthieme-connect.comresearchgate.net |
2015 | Chen, Zhai, et al. | Asymmetric | 12 | 5 | Organocatalytic Diels-Alder, Intramolecular SN2 researchgate.netresearchgate.netnih.gov |
Molecular Mechanisms of Action
Glycine Receptor (GlyR) Agonism and Modulation
Gelsemine is a functional modulator of glycine receptors (GlyRs), which are major ligand-gated ion channels controlling central nervous system (CNS) synaptic inhibition, particularly in the spinal cord and brainstem mdpi.comnih.govwikipedia.orgphysiology.org. This compound has been shown to directly modulate recombinant and native glycine receptors, exhibiting conformation-specific and subunit-selective effects nih.gov. While initial reports suggested agonistic activity wikipedia.org, further studies indicate a more complex modulatory profile, including potentiation and inhibition depending on the GlyR subunit composition and this compound concentration nih.gov.
Subunit-Specific Actions on Glycine Receptor Isoforms (e.g., α1, α2, α3)
This compound exerts subunit-specific actions on GlyRs composed of alpha (α) subunits mdpi.com. Studies on homomeric GlyRs (composed solely of alpha subunits) have revealed distinct effects. This compound displayed a bell-shaped modulation on currents through homomeric α1GlyRs, meaning it potentiated currents at lower concentrations and inhibited them at higher concentrations mdpi.comnih.gov. In contrast, it showed a consistent concentration-dependent inhibition on homomeric α2 and α3GlyRs mdpi.comnih.gov.
The presence of the beta (β) subunit can influence this compound's modulation. For example, 10 µM this compound potentiated glycine-activated currents through α1 homomeric receptors, but currents through α1β heteromeric receptors were not significantly modulated at this concentration. nih.gov. However, at 50 µM, this compound still potentiated α1 homomers but inhibited α1β heteromers nih.gov. The this compound-induced inhibition of homomeric and heteromeric α2 and α3 glycine receptors, however, displayed similar pharmacological profiles, suggesting that the beta subunit does not significantly influence this compound's inhibitory effect on these subtypes nih.gov.
Ligand Binding and Functional Modulation Characteristics
Experimental evidence from radioligand assays and electrophysiological analyses suggests that this compound's actions on GlyRs occur in a competitive manner mdpi.comresearchgate.net. This compound displaces glycine concentration-response curves mdpi.comresearchgate.netmdpi.com. Specifically, it displaces the curves for homopentameric α1GlyRs to the left, consistent with potentiation at lower concentrations, and displaces the curves for α2 and α3GlyRs to the right, consistent with inhibition mdpi.comresearchgate.net.
This compound modulation of GlyRs is voltage-independent nih.gov. The modulation is associated with differential changes in the apparent affinity for glycine and in the open probability of the ion channel nih.gov. Single-channel recordings have shown that this compound significantly enhanced the open probability of α1GlyRs nih.gov.
The binding site for low-toxicity alkaloids like this compound on GlyRs is primarily located at the orthosteric site between two α-subunits mdpi.com. Studies using chimeric and mutated GlyRs indicate that the extracellular domain (ECD) and residues within the orthosteric site are critical for the alkaloid's effects mdpi.comresearchgate.net. For instance, mutation of F63 on the α-subunit to alanine results in the loss of this compound's ability to regulate GlyR, suggesting F63 plays a crucial role in this compound binding mdpi.com.
Role of GlyR in Inhibitory Neurotransmission
Glycine receptors mediate fast inhibitory neurotransmission primarily in the spinal cord, brainstem, and retina nih.govwikipedia.orgguidetopharmacology.org. They are crucial for controlling motor and sensory functions, including vision and audition nih.gov. GlyRs are ligand-gated chloride ion channels; upon binding of an agonist like glycine, the channel opens, allowing chloride ions to flow into the neuron nih.govwikipedia.org. This influx of negatively charged chloride ions hyperpolarizes the postsynaptic membrane, making it less likely for the neuron to fire an action potential, thus mediating inhibition nih.govwikipedia.org. GlyRs are also found presynaptically, where they can modulate neurotransmitter release guidetopharmacology.org.
This compound's modulation of GlyRs can significantly impact this inhibitory neurotransmission. Potentiation of α1GlyRs could theoretically enhance inhibition, while inhibition of α2 and α3GlyRs could reduce it. The subunit-specific and concentration-dependent effects of this compound on GlyRs contribute to its complex pharmacological profile.
Gamma-Aminobutyric Acid Type A Receptor (GABAAR) Modulation
This compound also acts as a functional modulator of Gamma-Aminobutyric Acid Type A Receptors (GABAARs), which are the main biological targets of benzodiazepines and play a significant role in anxiety and pain pathways researchgate.netfrontiersin.orgnih.gov. GABAARs are also ligand-gated ion channels that mediate inhibitory neurotransmission, primarily through chloride ion influx wikipedia.org.
Functional Inhibition of Recombinant and Native GABAARs
Electrophysiological recordings have demonstrated that this compound inhibits the agonist-evoked currents of both recombinant and native GABAARs researchgate.netfrontiersin.orgnih.gov. Studies using recombinant GABAARs expressed in HEK293 cells and native receptors in cortical neurons have consistently shown this inhibitory effect researchgate.netfrontiersin.orgnih.gov. The functional inhibition by this compound is not associated with the benzodiazepine binding site researchgate.netfrontiersin.orgnih.gov.
This compound inhibits recombinant and native GABAARs with reported IC50 values of approximately 55-75 µM mdpi.comresearchgate.net. The inhibitory actions exerted by this compound on GABAAR configurations widely expressed in the CNS and associated with benzodiazepine actions were similar, implying the absence of significant subunit-selective effects on these particular configurations nih.gov. This compound displaces the GABA concentration-response curve to the right, consistent with competitive inhibition mdpi.comresearchgate.net.
Presynaptic Modulation of GABAergic Synaptic Function
The negative modulation of GABAARs and GABAergic synaptic function by this compound suggests that its mechanism of action is distinct from that of prototypical benzodiazepines frontiersin.org. While this functional profile may not support direct anxiolytic or analgesic actions through GABAARs, the reduction of GABAergic currents, along with GlyR inhibition, may contribute to the toxicity profile of this compound frontiersin.orgnih.gov.
Here is a summary of the effects of this compound on Glycine Receptor Subunits:
GlyR Subunit | This compound Concentration | Observed Effect |
α1 Homomeric | Lower | Potentiation |
α1 Homomeric | Higher | Inhibition |
α1β Heteromeric | 10 µM | Not significantly modulated |
α1β Heteromeric | 50 µM | Inhibition |
α2 Homomeric | All | Concentration-dependent Inhibition |
α2 Heteromeric | All | Concentration-dependent Inhibition |
α3 Homomeric | All | Concentration-dependent Inhibition |
α3 Heteromeric | All | Concentration-dependent Inhibition |
Here is a summary of the effects of this compound on GABAARs:
Receptor Type | This compound Effect | IC50 (approximate) | Binding Site Association | Presynaptic Effect |
Recombinant GABAARs | Functional Inhibition | 55-75 µM | Not associated with BDZ site researchgate.netfrontiersin.orgnih.gov | Diminished frequency of synaptic events |
Native GABAARs | Functional Inhibition | 55-75 µM | Not associated with BDZ site researchgate.netfrontiersin.orgnih.gov | Diminished frequency of synaptic events |
Neurosteroid Biosynthesis Pathway Modulation
This compound has been shown to modulate the neurosteroid biosynthesis pathway, specifically influencing the production of allopregnanolone. frontiersin.orgnih.govresearchgate.netresearchgate.net This modulation is suggested to contribute to some of its observed effects, such as anxiolytic and analgesic properties. frontiersin.orgresearchgate.netresearchgate.nettandfonline.commdpi.com
Upregulation of 3α-Hydroxysteroid Oxidoreductase (3α-HSOR) Expression
Research indicates that this compound can stimulate the biosynthesis of allopregnanolone through the upregulation of the mRNA expression of 3α-hydroxysteroid oxidoreductase (3α-HSOR). researchgate.netresearchgate.nettandfonline.comtaylorandfrancis.comnih.gov 3α-HSOR is a key enzyme involved in the production of neuroactive 3α-reduced steroids like allopregnanolone. nih.govnih.gov Studies in rat spinal cord have demonstrated that this compound increased 3α-HSOR synthesis. nih.gov This effect is suggested to be dependent on glycine receptor activation. nih.govresearchgate.net
Stimulation of Allopregnanolone Biosynthesis
This compound has been shown to stimulate the biosynthesis of the neurosteroid allopregnanolone (also known as 3α,5α-tetrahydroprogesterone or 3α,5α-THP). frontiersin.orgnih.govresearchgate.netresearchgate.nettandfonline.commdpi.com Allopregnanolone is known for its significant neurobiological actions, including neuroprotective, anxiolytic, and analgesic effects. nih.govresearchgate.net Studies using rat spinal cord slices demonstrated that this compound increased the conversion of [(3)H]-progesterone into [(3)H]3α,5α-THP. nih.gov This stimulation of allopregnanolone production by this compound is suggested to occur via the activation of 3α-HSOR. researchgate.net The effects of this compound on allopregnanolone biosynthesis have been observed in the spinal cord and hippocampus. researchgate.net
Transglutaminase 2 (TG2) Enzyme Inhibition
Recent studies have identified transglutaminase 2 (TG2) as a molecular target of this compound. nih.govresearchgate.netmdpi.comnih.govmdpi.com this compound has been found to inhibit the activity of this enzyme. nih.govresearchgate.netmdpi.com TG2 is an enzyme involved in protein cross-linking and has emerging roles in processes such as amyloid-beta (Aβ) aggregation and neurotoxicity. nih.govresearchgate.netmdpi.comresearchgate.net
Interaction with TG2 Catalytic Site
Molecular modeling and biochemical analyses suggest that this compound interacts directly with the catalytic site of the TG2 enzyme, leading to its inhibition. nih.govresearchgate.netmdpi.comnih.govresearchgate.netresearchgate.net This interaction is primarily mediated by hydrophobic contacts and further stabilized by hydrogen bonding. nih.govmdpi.com This binding to the catalytic core is thought to prevent the enzyme's access to substrates, such as the Aβ peptide, thereby hindering its transamidating activity. mdpi.com
Modulation of Protein Aggregation Processes (e.g., Aβ peptide)
This compound modulates TG2-mediated protein aggregation processes, particularly the aggregation of the amyloid-beta (Aβ) peptide, which is implicated in Alzheimer's disease. nih.govresearchgate.netmdpi.comnih.govmdpi.com By inhibiting TG2 activity, this compound attenuates the aggregation of Aβ. nih.govresearchgate.netmdpi.com Experimental data indicates that this compound treatment can significantly reduce the formation of detectable Aβ aggregates. nih.govresearchgate.net This modulation of Aβ aggregation by this compound is associated with a reduction in Aβ-induced neurotoxicity and preservation of neuronal function in cellular models. nih.govresearchgate.netmdpi.comnih.gov The proposed mechanism involves the disruption of TG2-mediated beta-sheet formation, which hinders Aβ nucleation and aggregation, potentially leading to the formation of less toxic Aβ species. mdpi.com
Data illustrating the concentration-dependent inhibition of TG2 activity by this compound has been reported. researchgate.net
This compound Concentration | TG2 Activity (% of control) |
0 µM | 100 |
10 µM | ~80 |
25 µM | ~60 |
50 µM | ~40 |
100 µM | ~20 |
Note: Data is approximate based on graphical representation in source researchgate.net.
Anti-inflammatory Pathway Modulation
This compound has demonstrated anti-inflammatory properties. nih.govresearchgate.netmdpi.comnih.govmdpi.comresearchgate.net Studies suggest that its anti-inflammatory effects may involve the modulation of specific signaling pathways and the reduction of pro-inflammatory mediators. researchgate.netnih.gov
Research indicates that this compound can suppress the activation of the NLRP3 inflammasome. researchgate.netnih.govresearchgate.net The NLRP3 inflammasome is a key component of the innate immune system that plays a role in inflammatory processes and the release of pro-inflammatory cytokines. nih.gov By inhibiting the NLRP3 inflammasome pathway, this compound appears to reduce the production of inflammatory cytokines such as interleukin-1β (IL-1β) and interleukin-6 (IL-6). researchgate.netnih.gov This inhibitory effect on inflammatory cytokine production has also been observed with other anxiolytic compounds. nih.gov Furthermore, this compound has been shown to reduce neuroinflammation by inhibiting the overactivation of microglia and astrocytes, which are involved in the inflammatory response in the central nervous system. nih.govmdpi.com
Inhibition of Inflammasome Activation (e.g., NLRP3)
Studies have indicated that this compound can modulate inflammasome activation. Specifically, research in a mouse model of chronic unpredictable mild stress (CUMS) demonstrated that this compound inhibited the activation of NLRP3-inflammasome pathways in the hypothalamus. nih.govnih.govresearchgate.net The NLRP3 inflammasome is a multiprotein complex that plays a critical role in the innate immune system. frontiersin.orgnih.govuni-bonn.deproquest.comnih.gov Its activation is a key event in the inflammatory response, leading to the cleavage and release of pro-inflammatory cytokines such as interleukin-1β (IL-1β) and interleukin-18. frontiersin.orgnih.govuni-bonn.deproquest.comnih.gov Inhibition of the NLRP3 inflammasome is therefore associated with a reduction in the maturation and release of these inflammatory mediators. guidetopharmacology.org While the precise mechanism by which this compound inhibits NLRP3 inflammasome activation requires further detailed investigation, the observed effect suggests a potential role for this compound in mitigating inflammation mediated by this pathway. nih.govnih.govresearchgate.net
Downregulation of Pro-inflammatory Cytokines (e.g., Interleukin-1β, Interleukin-6)
Beyond its effects on inflammasome activation, this compound has been shown to directly influence the levels of key pro-inflammatory cytokines. In CUMS-induced mice, this compound treatment resulted in decreased levels of interleukin-1β (IL-1β) and interleukin-6 (IL-6) in both the hypothalamus and hippocampus. nih.govnih.govresearchgate.net IL-1β and IL-6 are well-established pro-inflammatory cytokines involved in a wide range of inflammatory processes and immune responses. nih.govfrontiersin.orgzellavie.ch The observed reduction in these cytokine levels following this compound administration in experimental models suggests an anti-inflammatory effect. nih.govnih.govresearchgate.netfrontiersin.org Research also suggests a potential interplay between the reduction of these cytokines and the modulation of other pathways, such as CREB and BDNF expression. nih.gov
Structure-activity Relationship Sar Studies
Correlating Structural Features with Receptor Binding Affinity and Modulation
Gelsemine has been identified as a modulator of inhibitory ligand-gated ion channels in the central nervous system, specifically glycine receptors (GlyRs) and GABA type A receptors (GABAARs). mdpi.comresearchgate.netmdpi.com These receptors play a critical role in regulating neuronal activity. Studies have shown that this compound can directly modulate recombinant and native GlyRs, exhibiting conformation-specific and subunit-selective effects. nih.gov This modulation appears to involve changes in the apparent affinity for glycine and the open probability of the ion channel. nih.gov
This compound's interaction with GlyRs is complex. At low micromolar concentrations, it can potentiate currents through homomeric α1 GlyRs, while at higher concentrations, it shows inhibition, resulting in a bell-shaped modulation profile. nih.gov this compound also inhibits α2 and α3 GlyRs in a concentration-dependent manner. mdpi.com For GlyRs from spinal cord tissue, this compound displaces 3H-strychnine-binding curves, indicating a competitive mechanism of action on native GlyRs with an IC50 value of approximately 40 μM. mdpi.com Molecular docking studies suggest that this compound binds to the orthosteric site of the GlyR. mdpi.com
This compound also modulates GABAARs, generally exhibiting inhibitory actions on recombinant and native receptors with IC50 values ranging from approximately 55 to 75 μM. mdpi.com This modulation is not associated with the benzodiazepine binding site. nih.govresearchgate.net Compared to classical modulators like diazepam (for GABAARs) and strychnine (for GlyRs), this compound's modulatory activity is considered weaker. mdpi.com Molecular docking studies suggest that the transmembrane region of the β+/α− interface serves as a common binding site for this compound and other Gelsemium alkaloids on GABAARs. mdpi.com The binding free energy results from computational studies indicate that this compound has a more favorable binding interaction with GlyR compared to various binding sites on GABAARs, suggesting GlyR as a more likely primary target for this compound's action. mdpi.com
Data on this compound's Receptor Modulation:
Receptor Subtype | Modulation Effect | IC50/Concentration Range | Reference |
α1 GlyR | Potentiation (low µM), Inhibition (high µM) | 0.1-50 µM (potentiation), >100 µM (inhibition) | nih.gov |
α2 GlyR | Inhibition | Concentration-dependent | mdpi.com |
α3 GlyR | Inhibition | Concentration-dependent | mdpi.com |
Native Spinal GlyR | Inhibition | ~40 µM (IC50) | mdpi.com |
Recombinant GABAARs | Inhibition | ~55-75 µM (IC50) | mdpi.com |
Native GABAARs | Inhibition | ~55-75 µM (IC50) | mdpi.com |
Impact of Structural Modifications on GlyR and GABAAR Modulation
While comprehensive SAR studies specifically detailing the impact of precise structural modifications to the this compound core on GlyR and GABAAR modulation are still an active area of research, some insights can be drawn from studies on related Gelsemium alkaloids and computational analyses.
Studies comparing this compound, Koumine, and Gelsevirine have shown that these alkaloids exert inhibitory actions on GlyR and GABAAR function, while Humantenmine was found to be inactive. mdpi.com This suggests that the structural differences between these alkaloids contribute to their differential activity at these receptors. Molecular docking and mutagenesis studies have indicated that the extracellular domain and specific residues within the orthosteric site, such as F63 of the α-subunit, are critical for the interaction of this compound, Koumine, and Gelsevirine with GlyRs. researchgate.netmdpi.com The fact that Humantenmine is inactive suggests that its structure lacks the necessary features to bind effectively to these sites. mdpi.com
SAR in Anti-Inflammatory and Neuroprotective Activities
This compound has demonstrated both anti-inflammatory and neuroprotective properties. wikipedia.orgmdpi.comresearchgate.net SAR studies in this area are also evolving, with some findings pointing to specific structural elements involved.
Regarding anti-inflammatory activity, studies on other Gelsemium alkaloids have provided some clues. For instance, Gelsechizines A and B showed potent anti-inflammatory effects, and SAR study suggested that the presence of a β-N-acrylate moiety might be an important factor for their activity. tandfonline.com While this finding is not directly about this compound, it indicates that specific functional groups within the Gelsemium alkaloid structure can be crucial for anti-inflammatory effects. This compound itself has been shown to reduce neuroinflammation by inhibiting the overactivation of microglia and astrocytes and decreasing the levels of pro-inflammatory cytokines like IL-1β, IL-6, and TNF-α in models of Alzheimer's disease. researchgate.netresearchgate.net
In terms of neuroprotective activity, this compound has shown promise against beta-amyloid (Aβ) oligomer-induced toxicity, a key factor in Alzheimer's disease. mdpi.comresearchgate.netresearchgate.net this compound's neuroprotective effects may involve inhibiting the activity of transglutaminase 2 (TG2), an enzyme implicated in Aβ aggregation and neurotoxicity. mdpi.comresearchgate.net Molecular modeling and biochemical analyses suggest that this compound interacts with the catalytic site of TG2. mdpi.comresearchgate.net This interaction, likely dependent on specific structural features of this compound, leads to the inhibition of TG2 and modulation of Aβ aggregation. mdpi.comresearchgate.net Additionally, this compound's neuroprotective effects in models of hypoxic-ischemic brain injury have been linked to the suppression of inflammation and oxidative stress, potentially through the activation of the Nrf2/HO-1 pathway. nih.gov The structural requirements for activating this pathway are not yet fully elucidated but are part of ongoing SAR investigations.
Development of this compound Analogs and Derivatives for Modified Biological Profiles
The potential therapeutic benefits of this compound are accompanied by its known toxicity, which limits its clinical application. nih.gov This has driven efforts to develop this compound analogs and derivatives with modified biological profiles, aiming to retain beneficial activities while reducing toxicity. wikipedia.org
The synthesis of this compound and its analogs is challenging due to its complex structure. However, advancements in synthetic strategies, including total synthesis and semi-synthesis from key intermediates, are enabling the creation of novel derivatives. tandfonline.com These synthetic efforts aim to provide access to a wider range of this compound-like structures for SAR studies and the identification of compounds with improved therapeutic windows. tandfonline.comuga.edu
The development of analogs involves targeted modifications to the this compound scaffold to investigate the impact on specific activities, such as receptor binding affinity, anti-inflammatory effects, and neuroprotective properties. By systematically altering different parts of the molecule, researchers can identify the structural determinants responsible for desired effects and those contributing to toxicity. This iterative process of synthesis and biological evaluation is fundamental to designing derivatives with enhanced potency, selectivity, and safety profiles. While specific details on the SAR of individual this compound analogs and derivatives are often reported in primary research articles, the overarching goal is to create compounds that can selectively target relevant pathways with reduced off-target effects, thereby mitigating toxicity while preserving therapeutic efficacy.
Analytical Methodologies and Characterization in Research
Chromatographic Separation and Identification Techniques
Chromatographic methods are essential for separating gelsemine from other compounds present in a sample before its detection and identification.
High-Performance Liquid Chromatography (HPLC) with Various Detectors (e.g., UV, PDA, QTOF/MS)
High-Performance Liquid Chromatography (HPLC) is a widely used technique for the separation of this compound and other alkaloids. HPLC can be coupled with various detectors to provide different types of information.
HPLC coupled with a UV detector (HPLC-UV) is employed for the quantitative analysis of this compound, koumine, and gelsenicine in Gelsemium elegans. nih.gov This method has shown significant variation in the contents of these constituents depending on the plant part and origin. nih.gov
Ultra-high performance liquid chromatography coupled with photo-diode array and quadrupole time-of-flight mass spectrometry (UHPLC-PDA-QTOF/MS) is a powerful approach for the comprehensive characterization and identification of alkaloids in Gelsemium elegans. mdpi.com, nih.gov This method allows for the identification or tentative identification of numerous alkaloids based on their exact mass information, fragmentation characteristics, and retention times. nih.gov For instance, UHPLC-PDA-QTOF/MS has been used to identify 38 alkaloids in G. elegans. nih.gov This coupling is also used for the quantification of key alkaloids like this compound, koumine, and gelsenicine, serving as important indexes for assessing the quality of G. elegans as a medicinal plant. mdpi.com
In LC-QTOF/MS analysis, parameters such as mobile phase additives, gradient analysis times, and MS acquisition modes are optimized to maximize the detection of components with high peak intensities. nih.gov For example, an Agilent series 1290 Infinity HPLC coupled with an Agilent 6530 Q-TOF mass spectrometer has been used with a Waters C₁₈ column (3.5 μm, 4.6 mm × 150 mm), a flow rate of 0.3 mL/min, and a column temperature of 30 °C. nih.gov
Gas Chromatography-Mass Spectrometry (GC/MS)
Gas Chromatography-Mass Spectrometry (GC/MS) is another technique that can be applied for the analysis of compounds, though its application to this compound specifically is less frequently highlighted in the provided search results compared to LC-MS methods. GC/MS is generally suitable for volatile or semi-volatile compounds. While the search results mention GC/MS analysis in the context of other plant extracts and compounds journaljocamr.com, jpmsonline.com, nih.gov, and a study mentioning "Determination of this compound by Gas ChromatograpHy-Mass" , detailed findings specifically on this compound analysis by GC/MS are not extensively provided.
Two-Dimensional Liquid Chromatography (2D-LC)
Two-Dimensional Liquid Chromatography (2D-LC) is a technique that offers enhanced separation capabilities by coupling two different separation mechanisms in sequence. chromatographyonline.com A 2D-LC method has been developed for the simultaneous determination of Gelsemium alkaloids, including this compound, koumine, and humantenmine, in honey samples. mdpi.com, nih.gov This method typically involves solid phase extraction (SPE) for sample purification followed by 2D-LC analysis. mdpi.com, nih.gov The 2D-LC method for this compound and other alkaloids in honey demonstrated good performance with correlation coefficients higher than 0.998, recoveries in the range of 81%-94.2%, intraday precision (RSD) of ≤5.0%, and interday RSD of ≤3.8%. mdpi.com, nih.gov The limit of detection (LOD) for this compound was reported as 2 ng/g, and the limit of quantification (LOQ) was 5 ng/g. mdpi.com, nih.gov Retention times for this compound in this 2D-LC method were approximately 7.28 minutes. mdpi.com Compared to traditional HPLC and mass spectrometry, this 2D-LC method offers a balance of lower cost than MS and higher sensitivity than standard HPLC. mdpi.com
Mass Spectrometry Applications
Mass spectrometry techniques are crucial for the identification and structural characterization of this compound based on its mass-to-charge ratio and fragmentation patterns.
Tandem Mass Spectrometry (LC-MS/MS) for Alkaloid Profiling
Liquid Chromatography-Tandem Mass Spectrometry (LC-MS/MS) is a highly sensitive and specific technique widely used for the profiling and quantification of this compound and related alkaloids in various matrices. oup.com, nih.gov, frontiersin.org This method is particularly valuable for the collective detection of Gelsemium alkaloids. oup.com
An LC-MS/MS protocol has been optimized for the detection of this compound and related alkaloids from Gelsemium elegans. oup.com This protocol has been applied in the investigation of suspected gelsemium poisoning cases by analyzing alkaloid profiles in urine samples. oup.com Reliable identification of this compound by LC-MS/MS is ensured by monitoring specific mass transitions and its chromatographic retention time. oup.com For example, the protonated ion of this compound ([M+H]⁺) at m/z 323 is typically monitored, with fragmentation optimized at different collision energies. oup.com
LC-MS/MS is also used for the quantification of this compound and other alkaloids in plant tissues. mdpi.com, semanticscholar.org Studies have quantified this compound in different organs of G. elegans, such as roots, stems, and leaves, using LC-MS/MS, providing data on its distribution and accumulation during plant growth. mdpi.com, semanticscholar.org For instance, in mature leaves, the content of this compound was reported as 122.4 µg/g. mdpi.com, semanticscholar.org The LOD and LOQ for this compound by LC-MS/MS have been reported as 5 ng/mL and 10 ng/mL, respectively. semanticscholar.org
Ultra-performance liquid chromatography/tandem mass spectrometry (UPLC-MS/MS) is a sensitive and rapid method developed for the detection of Gelsemium alkaloids, including this compound, in rat plasma for toxicokinetic studies. nih.gov This method utilizes positive electrospray ionization and multiple reaction monitoring for quantitative analysis. nih.gov Validation of UPLC-MS/MS methods for alkaloids like this compound in plasma has shown good precision (less than 16%), accuracy (between 86.9% and 113.2%), extraction efficiency (better than 75.8%), and matrix effects (88.5% to 107.8%). nih.gov Calibration curves for this compound in plasma using UPLC-MS/MS are linear in the range of 0.1–200 ng/mL with a correlation coefficient (R²) greater than 0.995. nih.gov
Electrospray Ionization Mass Spectrometry (ESI-MS) for Molecular Characterization
Electrospray Ionization Mass Spectrometry (ESI-MS) is a soft ionization technique commonly used for the molecular characterization of this compound and other alkaloids. oup.com, frontiersin.org, nih.gov ESI-MS typically produces protonated or deprotonated molecules, providing information about the molecular weight of the analyte. oup.com
Positive electrospray ionization (ESI) is effective for detecting this compound, typically yielding the protonated ion [M+H]⁺. oup.com The use of a simple solvent system, such as acetonitrile and formic acid, can promote the formation of the protonated ion without significant adduct formation with sodium or potassium. oup.com ESI-MS is often coupled with chromatographic techniques like LC (LC-ESI-MS) for the analysis of complex samples. frontiersin.org Desorption electrospray ionization mass spectrometry imaging (DESI-MSI), a variant of ESI-MS, has been used to visualize the spatial distribution of this compound and other alkaloids in plant tissues. mdpi.com, frontiersin.org This imaging technique allows for the in situ visualization of alkaloid distribution within different organs and tissues of the plant. mdpi.com, frontiersin.org
Molecular characterization using ESI-MS can involve obtaining full scan MS data to determine the molecular ion mass and then performing MS/MS or MSⁿ experiments to obtain fragmentation patterns that provide structural information. frontiersin.org, mdpi.com These fragmentation patterns can be compared with databases or reference standards for identification. frontiersin.org
Table of Compounds and PubChem CIDs
Compound Name | PubChem CID |
This compound | 279057 |
Koumine | 102004413 |
Gelsenicine | 21123652 |
Humantenmine | 11969479 |
Gelsevirine | 14217344 |
Interactive Data Tables
Based on the search results, here are some data points that can be presented in interactive tables:
Table 1: Quantitative Analysis of Alkaloids in Gelsemium elegans Mature Leaves by LC-MS/MS
Alkaloid | Content (µg/g) | Source |
This compound | 122.4 | mdpi.com, semanticscholar.org |
Koumine | 272.0 | mdpi.com, semanticscholar.org |
Gelsenicine | 155.1 | mdpi.com, semanticscholar.org |
Table 2: Performance Parameters of 2D-LC Method for Alkaloids in Honey
Parameter | Value | Alkaloid (if specific) | Source |
Correlation Coefficient (R²) | > 0.998 | This compound, Koumine, Humantenmine | mdpi.com, nih.gov |
Recovery (%) | 81-94.2 | This compound, Koumine, Humantenmine | mdpi.com, nih.gov |
Intraday Precision (RSD %) | ≤ 5.0 | This compound, Koumine, Humantenmine | mdpi.com, nih.gov |
Interday Precision (RSD %) | ≤ 3.8 | This compound, Koumine, Humantenmine | mdpi.com, nih.gov |
LOD (ng/g) | 2 | This compound, Koumine, Humantenmine | mdpi.com, nih.gov |
LOQ (ng/g) | 5 | This compound, Koumine | mdpi.com, nih.gov |
LOQ (ng/g) | 20 | Humantenmine | mdpi.com, nih.gov |
Retention Time (min) | ~7.28 | This compound | mdpi.com |
Retention Time (min) | ~8.30 | Koumine | mdpi.com |
Retention Time (min) | ~11.30 | Humantenmine | mdpi.com |
Table 3: Performance Parameters of UPLC-MS/MS Method for Gelsemium Alkaloids in Rat Plasma
Parameter | Range | Source |
Precision (%) | < 16 | nih.gov |
Accuracy (%) | 86.9-113.2 | nih.gov |
Extraction Efficiency (%) | > 75.8 | nih.gov |
Matrix Effects (%) | 88.5-107.8 | nih.gov |
Calibration Curve Range (ng/mL) | 0.1-200 | nih.gov |
Correlation Coefficient (R²) | > 0.995 | nih.gov |
(Note: The interactive nature of the tables is a conceptual representation based on the data found. Actual implementation depends on the display environment.)
Distribution and Quantification in Biological and Plant Samples for Research Purposes
Understanding the distribution and concentration of this compound within biological systems and its source plant, Gelsemium, is crucial for research into its properties and potential applications. Various analytical methodologies are employed to achieve this, providing insights into its spatial localization within plant tissues and its quantitative levels in different matrices.
Spatial Distribution Studies in Gelsemium Plant Tissues (e.g., roots, stems, leaves)
Research into the spatial distribution of alkaloids, including this compound, within Gelsemium elegans tissues has been conducted using techniques such as desorption electrospray ionization mass spectrometry imaging (DESI-MSI). This approach allows for the direct visualization of target molecules in situ without extensive extraction and processing frontiersin.org. Studies have visualized the distribution of this compound, alongside other alkaloids like koumine and gelsenicine, in different organs and tissues of G. elegans at various growth stages nih.govmdpi.comdntb.gov.uanih.gov.
Findings indicate that this compound, koumine, and gelsenicine are primarily located in the pith region of roots and stems, with concentrations decreasing gradually towards the epidermis nih.govmdpi.comnih.govnih.gov. Interestingly, these alkaloids have also been found in higher abundance in the leaf veins nih.govmdpi.comnih.gov. The spatial distribution can vary between different medicinal plants, suggesting tissue specificity in alkaloid localization frontiersin.org. The accumulation of these alkaloids in root and stem tissues has been observed to increase with plant growth and development nih.govmdpi.comnih.gov.
DESI-MSI has enabled the visualization of multiple alkaloids, with studies detecting numerous compounds in roots, stems, and leaves at both seedling and mature stages frontiersin.orgnih.gov. In mature G. elegans, specific patterns of distribution have been noted, with alkaloids found in the vascular bundle region of roots, the pith region of stems, and the leaf veins frontiersin.orgdntb.gov.uanih.gov.
Quantitative Analysis in Experimental Matrices
Quantitative analysis of this compound is essential for determining its concentration in various experimental matrices, including plant tissues and biological samples from research studies. Techniques such as High-Performance Liquid Chromatography coupled with UV detection (HPLC-UV) and Ultra-High Performance Liquid Chromatography coupled with tandem mass spectrometry (UHPLC-MS/MS or UPLC-MS/MS) are commonly employed for this purpose nih.govscienceopen.comnih.govresearchgate.netresearchgate.net.
HPLC-UV has been used for the quantitative analysis of major alkaloids like this compound, koumine, and gelsenicine in different parts and origins of G. elegans, revealing significant variations in content nih.govresearchgate.net. UHPLC-PDA-QTOF/MS has also been applied for the characterization and differentiation of alkaloids in various plant parts nih.gov.
UPLC-MS/MS methods have been developed and validated for the sensitive and rapid detection and quantification of this compound in biological matrices such as rat plasma and tissues for pharmacokinetic and tissue distribution studies scienceopen.comnih.gov. These methods typically involve sample preparation steps like acetonitrile precipitation and utilize internal standards for accurate quantification nih.gov. Calibration curves are established over a specific concentration range, and method validation parameters such as linearity, precision, accuracy, limits of detection (LOD), and limits of quantification (LOQ) are determined scienceopen.comnih.govresearchgate.netmdpi.com. For instance, a UPLC-MS/MS method for this compound in rat plasma and tissues showed linearity over a range of 1-500 ng/mL with an LOQ of 1.0 ng/mL nih.gov. Another UPLC-MS/MS method for multiple Gelsemium alkaloids in rat plasma had a calibration range of 0.1–200 ng/mL with a correlation coefficient greater than 0.995 scienceopen.com.
Quantitative analysis in plant tissues has shown varying concentrations of this compound depending on the plant part and potentially geographical origin and harvest season mdpi.comresearchgate.net. Studies quantifying this compound, koumine, and gelsenicine in mature G. elegans leaves reported content ranges, with koumine generally present at higher concentrations than this compound and gelsenicine in roots, stems, and leaves mdpi.comdntb.gov.uanih.gov. For example, in mature leaves, this compound content ranged from 114.7 to 130.1 μg/g mdpi.com.
Methods for quantifying this compound in other matrices like honey have also been developed using techniques such as solid phase extraction (SPE) combined with two-dimensional liquid chromatography (2D-LC), demonstrating good linearity, recovery, and precision mdpi.com.
Research findings on the quantitative distribution of this compound and other alkaloids in G. elegans tissues highlight the differences in content across different plant parts and growth stages.
Plant Part | Growth Stage | This compound Content (µg/g) | Other Alkaloids Mentioned | Analytical Method | Source |
Mature Leaves | Mature | 114.7 - 130.1 | Koumine, Gelsenicine | DESI-MSI, LC-MS/MS | mdpi.comdntb.gov.uanih.gov |
Mature Roots | Mature | Not specified (lower than Koumine) | Koumine (249.2), Gelsenicine | DESI-MSI, LC-MS/MS | mdpi.comdntb.gov.uanih.gov |
Mature Stems | Mature | Not specified (lower than Koumine) | Koumine (149.1), Gelsenicine | DESI-MSI, LC-MS/MS | mdpi.comdntb.gov.uanih.gov |
Roots | Seedling (30, 60, 90 day) | Accumulation increased with age | Koumine, Gelsenicine | LC-MS/MS | mdpi.com |
Stems | Seedling (30, 60, 90 day) | Accumulation increased with age | Koumine, Gelsenicine | LC-MS/MS | mdpi.com |
Leaves | Seedling (30, 60, 90 day) | Accumulation increased with age | Koumine, Gelsenicine | LC-MS/MS | mdpi.com |
Note: Content values for this compound in roots and stems were not explicitly provided in the snippets but were indicated as lower than Koumine.
Quantitative analysis methods are crucial for toxicokinetic studies and understanding the behavior of this compound in biological systems after administration scienceopen.comnih.gov. These studies provide valuable data for research purposes, including assessing tissue distribution and elimination nih.gov.
Matrix (Research Context) | Analytical Method | Linearity Range | LOQ | Source |
Rat Plasma | UPLC-MS/MS | 0.1–200 ng/mL | Not explicitly stated (calibration range starts at 0.1 ng/mL) | scienceopen.com |
Rat Plasma and Tissues | UPLC-MS/MS | 1-500 ng/mL | 1.0 ng/mL | nih.gov |
Honey | 2D-LC with SPE | 5–1000 ng/g | 5 ng/g | mdpi.com |
Preclinical Pharmacological Research in Experimental Models
Antinociceptive Actions in Pain Models
Gelsemine has demonstrated antinociceptive effects in various experimental pain models, including those simulating neuropathic and tonic pain states. researchgate.netualberta.ca
Studies in Neuropathic Pain Models (e.g., mechanical allodynia, thermal hyperalgesia)
Research has shown this compound's efficacy in models of neuropathic pain. Single intrathecal injections of this compound produced antinociception in models such as bone cancer-induced mechanical allodynia and spinal nerve ligation-induced painful neuropathy in rats. researchgate.netnih.govresearchgate.net In mice subjected to partial sciatic nerve ligation (PSNL), this compound administered intraperitoneally increased the mechanical threshold, indicating a reduction in mechanical allodynia. medchemexpress.comarctomsci.comnih.gov It also prolonged thermal latencies, suggesting an effect on thermal hyperalgesia in this model. medchemexpress.comarctomsci.comnih.gov The antinociceptive effects in PSNL mice were observed for several hours post-administration. medchemexpress.comarctomsci.comnih.gov Studies using intrathecal injection in rats with spinal nerve ligation also showed that this compound could elevate the down-regulated mechanical threshold. nih.gov
Antinociception in Tonic Pain Models (e.g., formalin-induced)
This compound has also shown antinociceptive activity in tonic pain models, such as the formalin test. researchgate.netualberta.caresearchgate.net Single intrathecal injections of this compound produced potent antinociception in formalin-induced tonic pain in rats. researchgate.netnih.govresearchgate.net
Mechanisms of Antinociception (e.g., spinal α3 GlyR activation)
A key mechanism proposed for this compound's antinociceptive effects involves the activation of spinal α3 glycine receptors (GlyRs). researchgate.netualberta.canih.govresearchgate.net Studies have shown that spinal this compound antinociception is blocked by intrathecal administration of strychnine, a specific glycine receptor antagonist. researchgate.netnih.govresearchgate.net Furthermore, gene ablation of the GlyR α3 subunit, but not the α1 subunit, nearly completely prevented this compound-induced antinociception in neuropathic pain models, providing strong evidence for the involvement of α3 GlyRs. researchgate.netnih.govresearchgate.net this compound has been shown to concentration-dependently displace H(3)-strychnine binding in rat spinal cord homogenates, indicating a direct interaction with GlyRs. researchgate.netnih.govresearchgate.netncats.io Electrophysiological studies suggest that this compound can modulate GlyRs, showing concentration-dependent inhibition on α2 and α3 GlyRs. mdpi.com The proposed mechanism for analgesic actions also involves GlyR-triggered stimulation of spinal neurosteroid production, which in turn may potentiate the activity of spinal GABAA receptors. frontiersin.orgnih.gov
Anxiolytic-like Activities in Behavioral Models
This compound has demonstrated anxiolytic-like activities in various behavioral models in rodents. frontiersin.orgresearchgate.netfrontiersin.orgresearchgate.net
Stress-Induced Anxiety Models (e.g., chronic unpredictable mild stress)
Studies have investigated this compound's effects in stress-induced anxiety models, including the chronic unpredictable mild stress (CUMS) model in mice. researchgate.netnih.govresearchgate.netnih.gov In CUMS-induced anxiety-like behavior in mice, this compound treatment alleviated the observed behavioral alterations. nih.govresearchgate.netnih.gov This was evidenced by increased distance traveled in the central zone in the open-field test, increased time spent and distance traveled in the light compartment, and an increased number of transitions in the light/dark transition test. nih.govresearchgate.netnih.govmdpi.com this compound also increased the percentage of entries and time spent in the open arm of the elevated plus-maze, another indicator of reduced anxiety-like behavior. nih.govresearchgate.netnih.gov
Neurobiological Correlates of Anxiolytic Effects
The neurobiological correlates underlying this compound's anxiolytic effects have been explored. In the CUMS mouse model, this compound treatment decreased the levels of pro-inflammatory cytokines, including interleukin (IL)-1β and IL-6, in the hypothalamus and hippocampus. nih.gov Further investigations in this model revealed that this compound inhibited the CUMS-induced activation of NLRP3 inflammasome pathways and modulated the expression of cAMP-response element-binding protein (CREB) and brain-derived neurotrophic factor (BDNF) in the hypothalamus and hippocampus. nih.gov The proposed mechanism for anxiolytic actions involves the GlyR-triggered stimulation of spinal neurosteroid production, such as allopregnanolone, which may then potentiate spinal GABAA receptors. frontiersin.orgnih.govfrontiersin.orgmdpi.comcore.ac.ukfrontiersin.org
Data Tables
Pain Model | Species | Administration Route | Key Finding | Citation |
Bone cancer-induced allodynia | Rat | Intrathecal | Potent and specific antinociception, dose-dependent | researchgate.netnih.govresearchgate.net |
Spinal nerve ligation neuropathy | Rat | Intrathecal | Potent and specific antinociception, dose-dependent | researchgate.netnih.govresearchgate.net |
Partial sciatic nerve ligation | Mouse | Intraperitoneal | Increased mechanical threshold and prolonged thermal latency | medchemexpress.comarctomsci.comnih.gov |
Formalin-induced tonic pain | Rat | Intrathecal | Potent and specific antinociception | researchgate.netualberta.canih.govresearchgate.net |
Anxiety Model | Species | Stressor Type | Key Behavioral Findings | Citation |
Chronic unpredictable mild stress | Mouse | Unpredictable stressors | Increased time/distance in light compartment (LDT), increased entries/time in open arms (EPM), increased distance in central zone (OFT) | nih.govresearchgate.netnih.govmdpi.com |
Anti-Inflammatory Activities in Experimental Systems
Studies have indicated that this compound possesses anti-inflammatory effects in experimental systems. In mouse models, this compound has been shown to decrease the levels of pro-inflammatory cytokines, including interleukin (IL)-1β and IL-6, in the hypothalamus and hippocampus nih.govnih.gov. Furthermore, investigations suggest that this compound can inhibit the activation of the NLRP3-inflammasome pathway, which is involved in inflammatory responses nih.govresearchgate.net. Its anti-inflammatory actions have also been noted in the context of alleviating pain and neuroinflammation in experimental settings researchgate.netmdpi.comualberta.ca.
Anti-Cancer Activities in In Vitro and In Vivo Cancer Models
This compound and other alkaloids from Gelsemium elegans have demonstrated anti-tumor activities in both in vitro and in vivo models against a range of cancer cell lines. These include inhibitory effects on the proliferation of liver cancer, colon cancer, gastric cancer, rectal cancer, lung adenocarcinoma, and epidermoid carcinoma cells mdpi.com. Studies have shown that this compound exhibits anti-tumor activity in a dose-dependent manner in the digestive tract mdpi.com. While comparisons are often made with other alkaloids like koumine, this compound itself has been tested for cytotoxic activity, such as on PC12 cells acs.org.
Neuroprotective Effects in Models of Neurodegenerative Conditions (e.g., Alzheimer's disease cellular models)
This compound has shown significant neuroprotective effects in experimental models, particularly those related to neurodegenerative conditions like Alzheimer's disease (AD) nih.govmdpi.commdpi.commdpi.comresearchgate.netnih.gov.
Attenuation of Aβ Peptide-Induced Neurotoxicity
Research indicates that this compound exerts neuroprotective effects against neurotoxicity induced by beta-amyloid (Aβ) oligomers, a key peptide implicated in the pathogenesis of AD nih.govmdpi.commdpi.commdpi.comresearchgate.netnih.gov. This compound has been found to modulate the aggregation process of the Aβ peptide, leading to a significant reduction in its neurotoxicity at the cellular level nih.govmdpi.comresearchgate.netnih.gov. A crucial molecular target identified for this compound's action is transglutaminase 2 (TG2), an enzyme involved in protein cross-linking and with emerging roles in Aβ aggregation and neurotoxicity nih.govmdpi.commdpi.commdpi.comresearchgate.netnih.gov. Molecular modeling and biochemical analyses suggest that this compound interacts with the catalytic site of TG2, resulting in its inhibition nih.govmdpi.comresearchgate.netnih.gov. Studies in Aβ oligomer-treated mice have shown that this compound can improve cognitive and spatial impairments and reduce neuroinflammation by inhibiting the overactivation of microglia and astrocytes nih.gov.
Molecular docking calculations have provided insights into the interaction between this compound and the TG2 catalytic site. The docking score and binding free energy (ΔG) indicate a favorable interaction. mdpi.com
Compound | Docking Score (Glide) | Binding Free Energy (ΔG, kcal/mol) |
---|---|---|
This compound | - | -32.24 |
Koumine | - | -31.35 |
Gelsevirine | - | -22.07 |
Gelsenicine | - | -21.77 |
Note: Docking scores were not explicitly provided in the snippet, only binding free energy (ΔG) values. mdpi.com
Protection Against Oxidative Stress
This compound has also demonstrated protective effects against oxidative stress in experimental neuronal models frontiersin.orggoogle.comnih.govtaylorandfrancis.com. It has been shown to protect neurons against cell death induced by oxidative stress, such as that caused by hydrogen peroxide (H2O2) google.com. Pre-treatment of neuronal cells with this compound has been observed to increase cell viability and reduce cell mortality in response to oxidative stress google.com. This neuroprotective effect against oxidative stress contributes to the potential of this compound in preventing or treating neurodegenerative conditions google.com.
Experimental results in neuronal cell cultures exposed to oxidative stress (H2O2) have shown that this compound treatment can increase the number of living neurons. google.com
Treatment | Effect on Neuronal Viability (relative to untreated control) |
---|---|
Oxidative Stress (H2O2) | Decreased viability (increased cell death) |
Oxidative Stress + this compound (various concentrations, e.g., 2CH) | Increased viability (reduced cell death) by at least 15-30% or more |
Note: Specific percentage increases varied depending on this compound concentration and pre-treatment conditions in the source. google.com
Q & A
Basic: What are the primary natural sources of gelsemine, and how are they identified in plant extracts?
This compound is primarily isolated from Gelsemium elegans and Gelsemium sempervirens. Identification involves chromatographic techniques (e.g., HPLC-UV) coupled with mass spectrometry (UHPLC-MS/MS) to distinguish this compound from structurally similar alkaloids like koumine and gelsenicine. Quantitative analysis of plant parts (roots, stems) reveals significant variability in alkaloid content, necessitating standardized extraction protocols .
Basic: What experimental models are commonly used to study this compound’s biological effects?
Common models include:
- Invertebrates : Tetrahymena thermophila for oxidative stress and DNA damage studies, where this compound inhibits growth (IC₅₀ = 0.8 mg/mL) and alters antioxidant enzyme activity (p < 0.05) .
- Mammals : Rodent models for neuropathic pain, where this compound (0.1–1 mg/kg) acts via spinal glycine receptors (GlyRα3) .
- In vitro : Human neuroblastoma cells for gene transcription analysis at low concentrations (6.59 × 10⁻⁹ M) .
Advanced: How can researchers reconcile contradictions in this compound’s solubility and bioactivity across studies?
Discrepancies arise from solvent residues (e.g., ethanol) in this compound extracts, which dominate at high dilutions (e.g., 10,000:1 ethanol-to-gelsemine ratio in homeopathic preparations). To mitigate:
- Use solvent-free purification methods (e.g., solid-phase extraction).
- Include solvent controls in behavioral assays to isolate this compound-specific effects .
Advanced: What molecular mechanisms underlie this compound’s analgesic effects, and how are they validated?
This compound binds selectively to GlyRα3 subunits in the spinal cord, confirmed via gene ablation studies. In GlyRα3-knockout mice, this compound’s analgesic effect in neuropathic pain models (e.g., spinal nerve ligation) is abolished, while GlyRα1 ablation shows no impact. Mechanistic validation requires:
- Electrophysiology to confirm glycine receptor modulation.
- Competitive assays with strychnine (GlyR antagonist, ID₅₀ = 3.8 μg) .
Basic: What analytical methods are recommended for quantifying this compound in biological matrices?
- Hair samples : UHPLC-MS/MS with LOQ = 0.5 pg/mg for forensic toxicology, enabling detection of single-dose exposure .
- Plant extracts : HPLC-UV with C18 columns, validated against reference standards to account for inter-plant variability .
Advanced: How do coexisting alkaloids in Gelsemium extracts confound this compound-specific research?
Extracts contain neurotoxic alkaloids (e.g., gelsenicine) that synergize or antagonize this compound’s effects. To address:
- Isolate this compound via preparative HPLC.
- Perform comparative bioassays (pure this compound vs. crude extract) to dissect individual contributions .
Basic: What are the critical parameters for designing dose-response studies on this compound?
- Range : 0.1–1 mg/kg in rodents for analgesia; 0.1–0.8 mg/mL in T. thermophila for toxicity.
- Endpoints : Oxidative stress markers (SOD, CAT activity), DNA damage (comet assay), and gene expression (qPCR for MAPK pathways) .
Advanced: What strategies resolve challenges in this compound’s total synthesis?
Key steps include:
- Cyclopentane functionalization : Aubert’s formal synthesis (2007) uses radical cyclization to construct the tetracyclic core.
- Stereocontrol : Qin’s 2012 synthesis employs asymmetric catalysis to set C9 and C20 stereocenters .
Basic: How can researchers ensure reproducibility in this compound studies using plant-derived material?
- Standardize plant sourcing (geographical origin, harvest season).
- Quantify alkaloid profiles (this compound, koumine, gelsenicine) for each batch .
Advanced: What computational tools are used to predict this compound’s pharmacokinetics and toxicity?
Retrosynthesis Analysis
AI-Powered Synthesis Planning: Our tool employs the Template_relevance Pistachio, Template_relevance Bkms_metabolic, Template_relevance Pistachio_ringbreaker, Template_relevance Reaxys, Template_relevance Reaxys_biocatalysis model, leveraging a vast database of chemical reactions to predict feasible synthetic routes.
One-Step Synthesis Focus: Specifically designed for one-step synthesis, it provides concise and direct routes for your target compounds, streamlining the synthesis process.
Accurate Predictions: Utilizing the extensive PISTACHIO, BKMS_METABOLIC, PISTACHIO_RINGBREAKER, REAXYS, REAXYS_BIOCATALYSIS database, our tool offers high-accuracy predictions, reflecting the latest in chemical research and data.
Strategy Settings
Precursor scoring | Relevance Heuristic |
---|---|
Min. plausibility | 0.01 |
Model | Template_relevance |
Template Set | Pistachio/Bkms_metabolic/Pistachio_ringbreaker/Reaxys/Reaxys_biocatalysis |
Top-N result to add to graph | 6 |
Feasible Synthetic Routes
Featured Recommendations
Most viewed | ||
---|---|---|
Most popular with customers |
Haftungsausschluss und Informationen zu In-Vitro-Forschungsprodukten
Bitte beachten Sie, dass alle Artikel und Produktinformationen, die auf BenchChem präsentiert werden, ausschließlich zu Informationszwecken bestimmt sind. Die auf BenchChem zum Kauf angebotenen Produkte sind speziell für In-vitro-Studien konzipiert, die außerhalb lebender Organismen durchgeführt werden. In-vitro-Studien, abgeleitet von dem lateinischen Begriff "in Glas", beinhalten Experimente, die in kontrollierten Laborumgebungen unter Verwendung von Zellen oder Geweben durchgeführt werden. Es ist wichtig zu beachten, dass diese Produkte nicht als Arzneimittel oder Medikamente eingestuft sind und keine Zulassung der FDA für die Vorbeugung, Behandlung oder Heilung von medizinischen Zuständen, Beschwerden oder Krankheiten erhalten haben. Wir müssen betonen, dass jede Form der körperlichen Einführung dieser Produkte in Menschen oder Tiere gesetzlich strikt untersagt ist. Es ist unerlässlich, sich an diese Richtlinien zu halten, um die Einhaltung rechtlicher und ethischer Standards in Forschung und Experiment zu gewährleisten.